Exploring Surface Plasmon Resonance: Key Insights
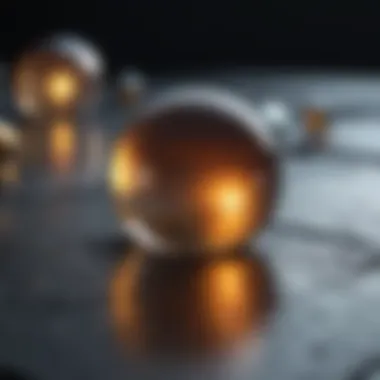
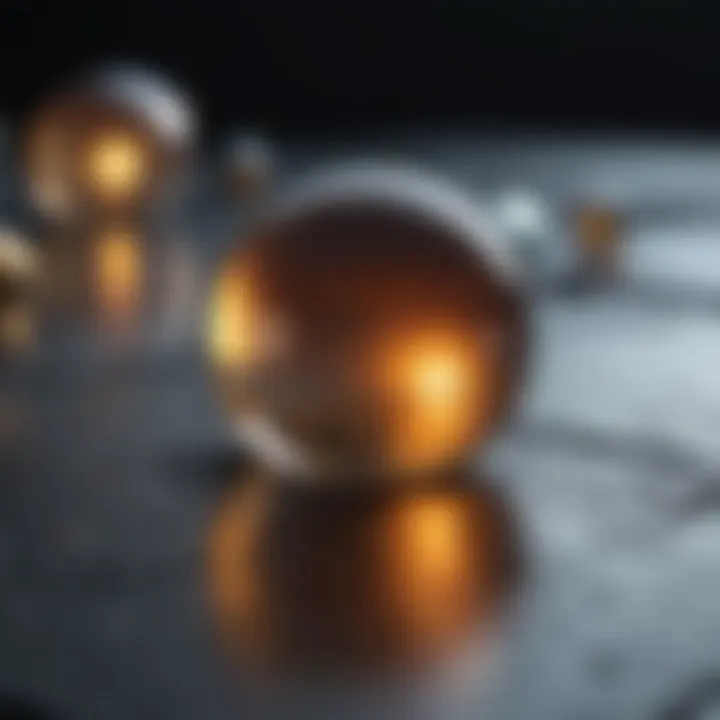
Intro
In the ever-evolving landscape of scientific research, technologies that enable precise measurements have become crucial. One such technology is surface plasmon resonance, often abbreviated as SPR. It is a powerful optical method for studying molecular interactions in real-time. The ability to monitor these interactions has far-reaching implications, particularly in areas such as biosensing and material characterization.
The principles behind SPR instruments revolve around the interaction of light with metal films. When light hits a metal surface at a particular angle, it can excite surface plasmons, leading to a resonance condition that reflects changes in the refractive index near the metal surface. This is not just a footnote in a physics textbook; it forms the backbone of many advanced applications ranging from healthcare diagnostics to cutting-edge material science research.
As we navigate through this article, we'll uncover the fundamental workings of SPR instruments, their design considerations, and their multifaceted applications. By the end, you’ll glean a comprehensive overview on why these tools are deemed indispensable in contemporary research.
Preamble to Surface Plasmon Resonance
Surface Plasmon Resonance (SPR) represents a pivotal technology in various scientific disciplines, serving as a bridge between fundamental physics and practical applications in fields like biochemistry and biosensing. Understanding SPR is essential for appreciating its transformative impact on research methodologies and technologies that enable us to probe molecular interactions in real time.
Definition and Overview
At its core, Surface Plasmon Resonance is an optical phenomenon that occurs when light hits a metal-dielectric interface at a specific angle, prompting surface plasmons—coherent electron oscillations that are sensitive to changes in the local refractive index. In simpler terms, it's a way to measure how molecules bind to surfaces by observing how light reflects off them. This technique has upended traditional analysis methods, thanks to its capacity to produce real-time data without labeling the analytes, thus preserving the natural state of the molecules involved. It’s almost like having a window into molecular interactions without distorting the view.
Historical Context of SPR Development
The roots of SPR trace back to the late 20th century, with significant developments arriving in the 1980s when researchers first began to harness this phenomenon for practical sensing applications. Figures like Toini K. Vainionpää and others demonstrated that SPR could be utilized to monitor biomolecular interactions effectively. This early work paved the way for commercial SPR instruments that are now staples in laboratories around the globe. Each step in the history of this technology has enhanced its precision and reliability, making it a beloved tool among scientists.
Importance in Modern Scientific Research
In today’s world, the relevance of SPR cannot be overstated. It plays a crucial role in various sectors, particularly in biochemistry, genetics, and material sciences. The ability to perform real-time, label-free monitoring of binding interactions between molecules has allowed researchers to refine drug development processes, optimize biosensors, and even evaluate the immune response in medical applications. Not only is it pivotal in academic laboratories, but industry giants also leverage SPR for quality control and research. The benefits are manifold:
- Real-time Data: Enables immediate feedback on molecular interactions.
- Label-free Detection: Minimal alteration of the sample, maintaining its integrity.
- High Sensitivity: Capable of detecting small analyte concentrations.
"SPR stands as a cornerstone of modern analytical techniques, pushing the boundaries of what is possible in molecular diagnostics and bioresearch."
Mechanisms of Surface Plasmon Resonance
Understanding the mechanisms behind Surface Plasmon Resonance (SPR) serves as the backbone of this discussion. The intricacies of how SPR operates not only sheds light on its effectiveness in diverse fields but also plays a significant role in the development of advanced applications that benefit from this technology.
Principles of Surface Plasmons
Surface plasmons are essentially coherent oscillations of electrons at the interface between a metal and a dielectric material. When light hits the metal surface under specific conditions, it can induce these oscillations, generating a phenomenon that is sensitive to slight changes in the local environment. The concept is wrapped around the ability of these surface plasmons to couple with light, which is crucial for the functionality of SPR instruments.
The unique property of surface plasmons is that they are bound to the surface, which means their energy is concentrated in a layer right at the interface. This spatial confinement enhances sensitivity, allowing for the detection of minute changes in mass or refractive index. For researchers, grasping these principles is essential for optimizing SPR setups and interpreting the data they produce effectively.
Excitation of Surface Plasmons
Exciting surface plasmons is a critical aspect of utilizing SPR technology. This process typically requires a specific alignment of light incident on the metal-dielectric interface. There are mainly two methods for excitation: the Kretschmann configuration and the Otto configuration.
In the Kretschmann method, incident light hits a prism which is coated with a thin layer of metal. When the angle of incidence reaches a certain threshold, known as the resonance angle, surface plasmons are excited, and a dip in reflectivity can be observed.
The versatility provided by these configurations allows scientists to tailor their SPR experiments according to different research needs. Understanding the nuances of excitation methods not only affects the setup but also influences the clarity of results produced during experiments.
Role of Light in SPR
Light plays a pivotal role in the functioning of SPR instruments—specifically, the wavelength and polarization of light can greatly influence the resonance condition. The interaction between the electromagnetic waves and the surface charges leads to enhanced energy transfer that is characteristic of surface plasmon resonance. This interplay is fine-tuned by adjusting aspects like the angle of incidence and the refractive index of the surrounding medium.
In many systems, the use of lasers offers a consistent source that can be finely controlled. However, it’s not merely the source of light that matters; the specific characteristics of the light—such as coherence and intensity—affect the overall efficiency of SPR measurement. Consequently, a deeper insight into the light’s role is necessary when designing experiments, ensuring that researchers can maximize the output of their SPR setups.
The effectiveness of SPR technology fundamentally hinges on the successful excitation of surface plasmons, which, in turn, is intricately linked to the careful management of light interactions at the sensor surfaces.
In summation, the mechanisms of SPR reveal a sophisticated interplay of physics that is at the heart of numerous applications. grasping these fundamentals not only empowers students and professionals to utilize SPR effectively but also invites them to contribute to the ongoing advancements in the technology.
Design and Components of SPR Instruments
Understanding the design and components of surface plasmon resonance (SPR) instruments is not just a technical necessity; it's a gateway to comprehending how these systems measure interactions at the molecular level. This section elaborates on the central components integral to SPR technology, ensuring anyone looking to leverage these instruments grasps their functionality and importance in varied applications.
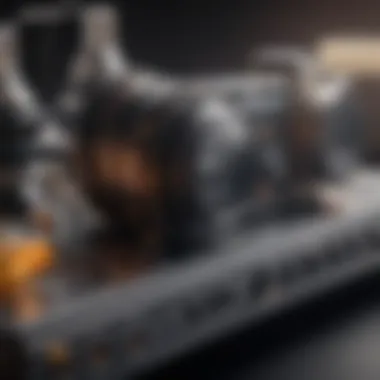
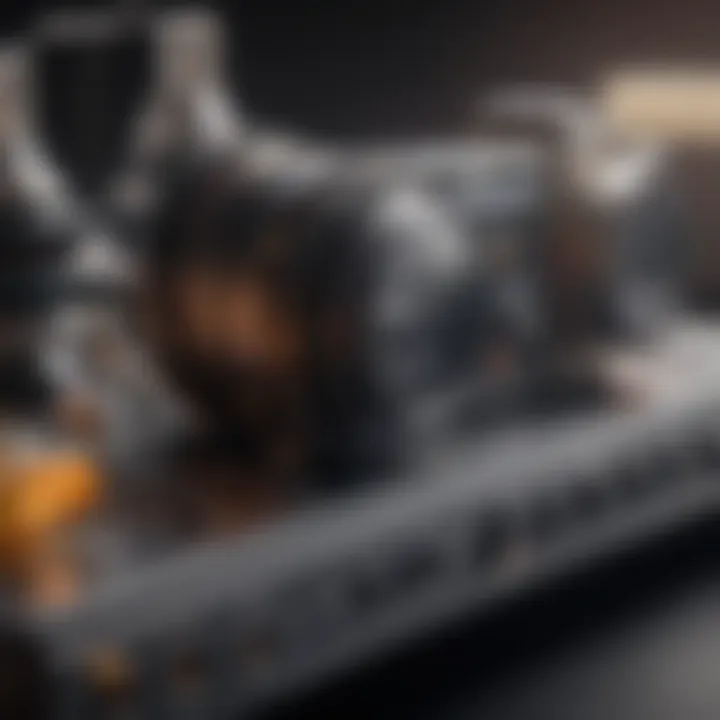
Basic Components: The Sensor Chip
At the heart of an SPR instrument lies the sensor chip, a key player in the journey of light and its interactions with the sample. Typically made from gold or silver films, these chips are engineered with utmost precision. When light hits these metal layers, surface plasmons are excited, leading to a change in refractive index that the instrument measures.
The design of the sensor chip can influence its performance. For instance, the thickness of the metal film and its smoothness are crucial in determining sensitivity. Using a high-quality chip ensures that less noise interferes with the signal, thus improving the overall resolution of measurements. Sometimes, researchers take it a step further, incorporating specific chemical modifications on the chip’s surface to selectively bind to target analytes. This enables specificity and enhances the chip’s performance in clinical or environmental applications.
Optical Setup and Configuration
How the optical setup is configured determines how effectively an SPR instrument can gather data. The typical arrangement involves a light source, a prism, and a detector. Light from sources like lasers or LEDs is directed through a prism, which then reflects onto the sensor chip at various angles. The angle of incidence is crucial; it impacts how surface plasmons are excited and thus influences the measurements.
In more complex configurations, adaptive optics may be utilized, allowing for finer control over the light pathways. This is particularly useful when dealing with small or low-concentration samples, where maximizing light interaction can make or break the measurement data. It’s like trying to find a needle in a haystack; every detail in the setup can either help or hinder the search.
Signal Detection Mechanisms
After the light interacts with the sensor chip, the next step is signal detection. This phase involves capturing the tiny changes in light intensity and angle as the surface plasmons are active. Detectors commonly used in SPR systems are photodiodes or CCD cameras, tasked with converting light signals into readable data. The precision here is paramount, as even minor variations can provide insights into biomolecule interactions or material properties.
Moreover, the data processing algorithms play a pivotal role. They analyze the captured signals, providing quantifiable metrics like binding affinities and kinetics. The accuracy of these calculations can drastically influence research outcomes, making knowledge in both hardware and software aspects indispensable for users. To put it plainly, it’s akin to having a high-end camera for stunning photos, but sans a knowledgeable photographer, you miss capturing the moment's essence.
In summary, understanding the intricate design and components of SPR instruments is essential for leveraging their capabilities effectively. Each element, from the sensor chip to the optical configuration and signal detection mechanisms, intertwines to create a sophisticated tool for molecular analysis. For those exploring deeper into this fascinating field, grasping these principles can pave the way for innovative discoveries in biosensing and beyond.
Applications of Surface Plasmon Resonance
Surface Plasmon Resonance (SPR) technology has caused ripples across a variety of fields, spurred by its unique capacity to deliver real-time analysis and high sensitivity. This section unveils a range of applications where SPR is making significant impacts. Whether it's in the realm of healthcare, environmental science, or food safety, the flexibility and capabilities of SPR instruments open doors to innovative solutions and advancements. Below, I present an exploration of four major applications:
Biosensing and Biomedical Applications
When it comes to biosensing, SPR instruments have carved out a niche that is hard to ignore. These devices are instrumental in detecting biomolecular interactions, which are critical in diagnostic applications. For instance, the ability to monitor hormone levels, detect pathogens, or analyze protein interactions in real-time offers a distinct advantage over traditional methods.
In biomedical research, SPR is employed for studying drug interactions and identifying potential biomarkers for diseases. Researchers have found that SPR can detect smaller concentrations of analytes, which is vital in the early diagnosis of diseases such as cancer. The non-invasive nature of SPR measurements enhances its utility, making it an attractive tool in clinical settings.
"In the fast-paced world of medical diagnostics, precision and speed are paramount. Surface Plasmon Resonance delivers both, positioning it as a powerful ally in the fight against diseases."
Material Science and Nanotechnology
Material science professionals have also embraced SPR technology, particularly in the examination of thin films and nanostructured materials. The ability to analyze changes in refractive index at the surface level makes SPR a go-to method for characterizing new materials. This is crucial when trying to understand the properties and behaviors of nanoparticles, which can lead to the development of novel materials with tailored functionalities.
In nanotechnology, SPR aids in the design and characterization of sensors used for environmental monitoring or biomedical applications. The interaction between light and nanoparticles provides insights into surface phenomena, which can inform advancements in catalysis and energy storage devices.
Food Safety and Quality Control
Food safety is an issue of paramount importance, and SPR instruments are stepping up to the plate. They help ensure quality control by detecting contaminants—like pathogens, toxins, or allergens—in food products quickly and reliably. The rapid nature of SPR analysis reduces the time between testing and result, leading to faster decision-making in food safety protocols.
For instance, companies can utilize SPR to monitor food processing environments effectively. This not only enhances consumer trust but also mitigates potential public health risks. Besides, by integrating SPR into their quality control processes, businesses can better comply with stringent regulations and standards in food production.
Environmental Monitoring
Lastly, the application of SPR technology extends to environmental monitoring, where it is used to detect pollutants in air, water, and soil. With global environmental concerns on the rise, the need for reliable and rapid detection methods becomes crucial. SPR systems can identify trace levels of various contaminants, including heavy metals or toxic substances, providing valuable data to researchers and policymakers.
In summary, the versatility of SPR applications highlights its significance across diverse sectors. Whether improving health outcomes or enhancing environmental sustainability, it is clear that this technology has a promising future ahead. By further exploring these applications, researchers and practitioners can unlock new opportunities for innovation and development.
Advantages of SPR Technology
Surface Plasmon Resonance technology offers a myriad of benefits, making it an invaluable tool in scientific research and clinical applications. The ability to gather real-time data without the need for labels is a principle advantage that sets it apart from traditional methodologies. Additionally, its sensitivity and specificity cater to the demanding requirements of diverse fields like biosensing and material sciences. This technology does not just serve one purpose; rather, its versatility opens doors to numerous applications.
Real-time and Label-free Analysis
One of the handiest features of SPR technology is its ability to perform real-time and label-free analysis. This means that researchers can monitor molecular interactions as they occur, without the interference of dye or other labeling agents. Imagine studying biomolecular interactions without worrying about the fluorescent markers that can, at times, alter the reaction kinetics. Not only does this facilitate precision, but it also significantly reduces analysis time.
- Key Benefits of Real-time Measurement:
- Instant Feedback: Researchers can adjust parameters on the fly, enhancing the robustness of experiments.
- Natural Conditions: Because it operates without external labels, the native state of interactions is preserved.
- Dynamic Studies: You can evaluate how interactions evolve over time, which is central in fields like drug discovery.
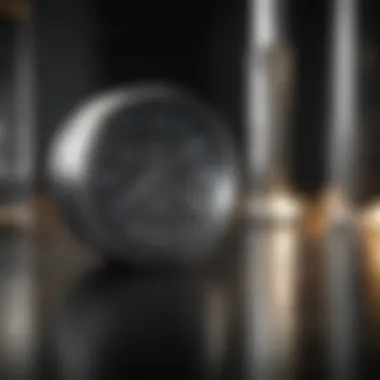
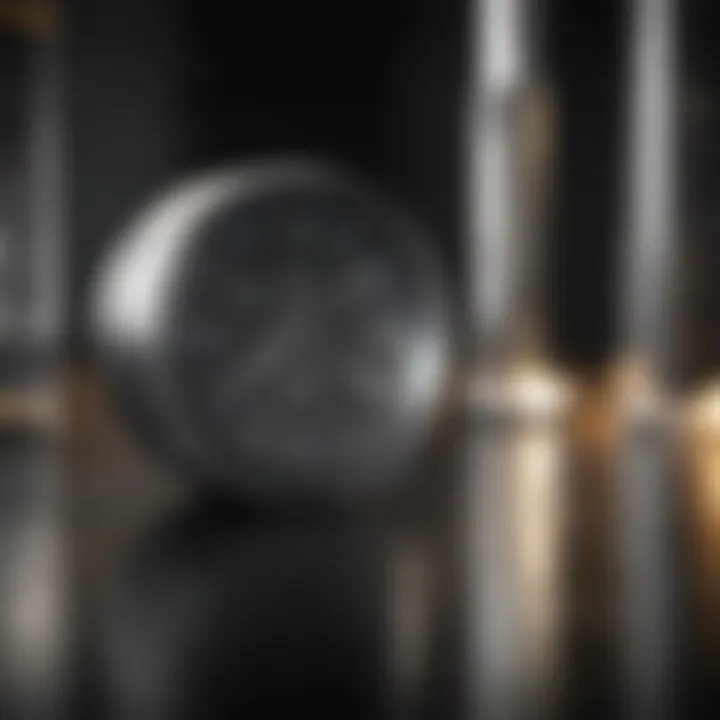
Sensitivity and Specificity
SPR technology is particularly lauded for its sensitivity and specificity. It can detect minute changes in refractive index near the sensor surface, allowing it to identify even the slightest molecular interactions. This extraordinary sensitivity is crucial when studying low-abundance biomolecules.
- Factors contributing to Sensitivity:
- Surface Chemistry: SPR surfaces can be engineered to enhance specific interactions, improving overall detection.
- Optical Properties: Advanced optics enable the detection of small shifts, which enhances sensitivity.
In terms of specificity, the technology utilizes biomolecular recognition layers that selectively bind to targeted molecules, minimizing background noise and enhancing signal quality. This specificity makes the technology suitable for diagnosing diseases by accurately detecting pathogen or biomarker presence without false positives.
Versatility in Various Applications
The versatility of SPR extends to numerous applications, allowing fields like biosensing, material science, and environmental monitoring to benefit greatly. Researchers employ this technology for a variety of uses:
- Biosensing: SPR is extensively employed for detecting proteins, nucleic acids, and even entire pathogens in clinical diagnostics.
- Material Science: The technology assists in characterizing thin films and nanostructures, essential for developing new materials.
- Environmental Monitoring: It plays an essential role in assessing pollutants and toxins, lending aid to regulatory compliance and public health.
In summary, the advantages of SPR technology make it a crucial asset in scientific research. Its real-time, label-free analysis provides researchers with a clearer lens to explore molecular interactions. Meanwhile, sensitivity ensures that even minor events are captured, and versatility allows for a diverse range of applications across multiple fields. As we delve deeper into the intricacies and innovations surrounding SPR, we can anticipate its expanding influence in future scientific endeavors.
"The evolution of SPR technology highlights its critical role in pushing the boundaries of scientific understanding and application."
For those interested in a deeper dive into the principles of surface plasmon resonance, more information can be found at Wikipedia.
Research institutions and professionals will find that fully leveraging SPR's capabilities can yield significant breakthroughs in their respective disciplines.
Limitations and Challenges of SPR Instruments
Surface Plasmon Resonance (SPR) instruments have brought significant advancements in scientific research, but no technology is without its hurdles. Understanding these challenges is crucial for optimizing their use in various fields. The limitations can impact the sensitivity, data interpretation, and cost, affecting researchers and institutions in their work. This section delves into specific issues related to sensitivity, data analysis, and cost implications, highlighting the importance of addressing these challenges.
Sensitivity Issues Under Certain Conditions
SPR technology is known for its exceptional sensitivity in detecting molecular interactions. However, there are specific scenarios where limitations in sensitivity could arise. For instance, the environmental conditions in which the experiments are conducted can play a pivotal role. High concentrations of noise, interference from other molecules, or changes in temperature might lead to compromised signal strength, affecting the detection accuracy.
Also, the kind of surface functionalization used on the sensor chip can lead to variability in the binding efficiency of the analytes. In certain instances, the analyte molecules may not adequately interact with the sensor surface, causing lower-than-expected signal responsiveness. Such sensitivity issues require researchers to carefully optimize their experimental protocols to minimize external influences.
Complex Data Analysis
In SPR, data analysis can become quite complex. The raw measurement obtained often necessitates sophisticated algorithms for interpreting the binding events. The interpretation of the sensogram, the graphical representation of the response over time, can be tricky. Researchers with varying levels of expertise might struggle to extract meaningful information without considerable trial and error.
Moreover, the multi-layered nature of the interactions that occur during experimentation can create challenges in discerning specific binding events. Often, background noise and other interactions can obscure the desired signal, requiring advanced statistical methods for accurate interpretation. This complexity highlights the importance of having trained personnel who can navigate through the data intricacies to provide reliable results.
Cost Implications for Research Institutions
The financial burden associated with acquiring and maintaining SPR instruments can be significant. For research institutions, especially those with limited funding, investing in SPR technology might not be feasible. The initial costs can include not only the instrument itself but also associated expenses for maintenance, consumables like sensor chips, and software updates for data analysis.
Moreover, due to the specialization required to operate SPR effectively, there is a need for well-trained personnel, which adds to the overall expenditure. In an era where budget constraints are commonplace, these costs can discourage potential users or lead to compromises in research quality and quantity.
"Balancing the benefits of SPR technology against the operational costs and challenges is essential for optimizing its impact in research."
Addressing these limitations is vital for any researcher considering the implementation of SPR techniques. As the technology evolves, it's crucial to also focus on solutions for these challenges to fully exploit the potential of SPR in diverse applications.
Recent Advances and Trends in SPR Technology
Recent years have seen significant strides in surface plasmon resonance (SPR) technology, propelling its application beyond traditional boundaries. These advancements not only enhance the capabilities of SPR instruments but also broaden their potential applications across various fields. With the rapid development in this area, it is crucial to understand these trends to fully grasp their implications for future research and industry practices.
Integration with Microfluidics
The melding of SPR technology with microfluidics represents a pivotal advance in the pursuit of more efficient and streamlined analyses. This integration enables researchers to conduct high-resolution studies with minimal sample volumes, making it particularly valuable in biosensing applications.
Microfluidic systems allow for precise control of liquid samples in tiny channels. This results in enhanced sensitivity and the ability to manipulate conditions with astounding accuracy.
- Benefits include:
- Reduced Sample Size: Scientists can work with smaller quantities of reagents, thus saving costs and reducing waste.
- Increased Throughput: Multiple reactions can occur simultaneously, expediting the data collection process.
- Improved Control over Experimental Conditions: Researchers can modulate flow rates, temperature, and chemical environments more easily.
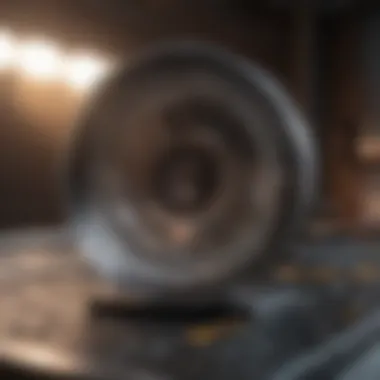
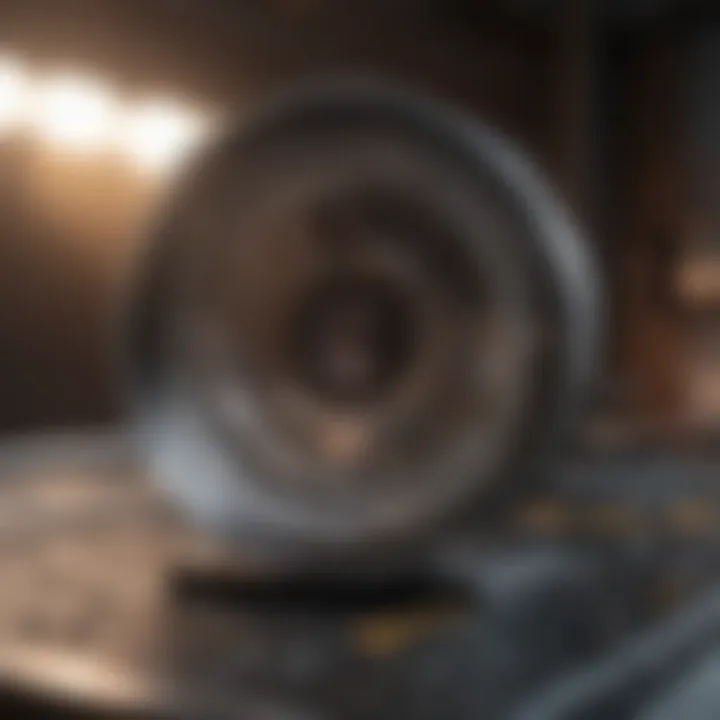
Moreover, combining microfluidics with SPR facilitates real-time monitoring of reactions, offering critical insights that were previously challenging to obtain.
Adaptation for High-Throughput Screening
High-throughput screening is a revolutionary method that allows for the rapid testing of thousands of samples simultaneously, an essential feature for speeding up drug discovery and development. Recent developments in SPR technology that accommodate high-throughput formats involve the use of multichannel sensors and automated systems that vastly improve efficiency.
Incorporating high-throughput capabilities means that researchers can detect biomolecular interactions at an unprecedented scale. With this capability, the following aspects are highlighted:
- Rapid Data Generation: Results that once took days can now be obtained in a matter of hours, driving faster decision-making in research.
- Broad Applications: Beyond pharmaceuticals, sectors such as agriculture and environmental monitoring can benefit from faster research cycles.
- Cost-Effectiveness: Reducing the time required for data collection also translates to significant financial savings for institutions.
Emerging Surface Plasmon-Based Techniques
As the field of SPR continues to evolve, several cutting-edge techniques are emerging, promising to take advantage of unique properties of surface plasmons. These new methods leverage the sensitive nature of plasmon resonance, enabling innovative applications that were once the stuff of dreams.
Some notable techniques include:
- Localized Surface Plasmon Resonance (LSPR): This technique focuses on nanoparticle-based systems that exhibit enhanced detection capabilities due to confinement effects.
- Three-Dimensional Plasmonic Structures: These structures improve light-matter interaction, expanding the potential for applications in sensor technology and imaging.
- Plasmon-Enhanced Fluorescence: This hybrid technique opens pathways to enhanced sensitivity that benefits analytical applications.
The fusion of traditional SPR methods with emerging techniques signifies a trailblazing path forward for both fundamental and applied research.
In summary, the recent advances and trends in SPR technology not only illustrate the ongoing evolution of this field but also underline its importance in paving the way for future innovations. Understanding these trends equips students, researchers, and industry professionals with the knowledge necessary to harness the full potential of SPR in diverse applications.
Future Prospects of Surface Plasmon Resonance
Surface plasmon resonance (SPR) technology is poised for extended growth and innovation in various scientific arenas. This section focuses on how the future of SPR can reshape multiple fields, highlighting crucial developments in instrumentation, fostering cross-disciplinary collaborations, and outlining a forward-thinking vision for SPR technology.
Potential Innovations in Instrumentation
Looking forward, the landscape of SPR instruments is likely to see significant advancements. Innovations in instrumentation could hinge on miniaturization and integration with microfluidic systems that amplify detection capabilities while also lowering analysis time. For instance, the possibility of portable SPR devices could lead to on-site testing in clinical settings or environmental monitoring, enhancing real-time data acquisition.
Moreover, merging SPR with technologies like nanophotonics can improve sensitivity even further. Developing sensors with advanced materials, including graphene and metal-organic frameworks, promises enhanced performance, particularly in detecting low-abundance targets. Such potential could mean that scientists are no longer chasing shadows but finding concrete answers quickly and effectively.
Impact on Interdisciplinary Research
The ripple effect of SPR technology on interdisciplinary research cannot be overstated. As SPR integrates into diverse studies, including biology, chemistry, and material science, it paves the way for new methodologies and collaborative endeavors. For example, translating data from SPR experiments into fields like drug discovery and diagnostics could accelerate the development of tailored therapeutics, dramatically altering patient care.
Additionally, as researchers collaborate across various disciplines, they may uncover synergies that previously existed in silos. This teamwork could lead to innovative applications; for example, using SPR to study protein interactions in novel biomaterials or employing it for environmental surveillance to track pollutants more efficiently.
Long-term Vision for SPR Technology
Envisioning the long-term trajectory of SPR technology elicits enthusiastic discussions among researchers. An optimistic outlook includes the potential for automated and standardized protocols for SPR analysis, much like what is in the realm of high-throughput screening today. This would not only streamline research processes but also facilitate large-scale studies across numerous applications.
In the grand scheme, we might even see a unified platform that integrates various biosensors with SPR principles at the core, allowing for concurrent analyses of multiple parameters. This convergence could usher in a new era in diagnostics and environmental monitoring, enabling practitioners to address multifaceted challenges more comprehensively.
The future of surface plasmon resonance holds profound implications for both the scientific community and society at large.
In summary, while it is imperative to recognize the current challenges facing the field, the exciting developments on the horizon indicate a promising trajectory for SPR technology. Researchers, educators, and professionals alike should remain engaged with this evolving narrative, as the connections forged through innovation and collaboration may ultimately lead to groundbreaking discoveries.
Epilogue
In wrapping up the discussion on surface plasmon resonance (SPR) instruments, it's crucial to reflect on their multifaceted roles within scientific research and beyond. The exploration of how these tools operate not only enriches our understanding of physical principles at play but also highlights their practical applications in various fields, such as biomedical research and environmental monitoring.
Summation of Key Points
The primary takeaways from our deeper dive into SPR technology include:
- Core Principles: Understanding the fundamental processes that enable surface plasmon resonance provides insight into the delicate interplay between light and matter.
- Instrumental Design: The components and configuration of SPR instruments, including sensor chips and optical setups, are pivotal for achieving accurate results.
- Diverse Applications: From biosensing to material science, the versatility of SPR instruments demonstrates their value across numerous disciplines, making them indispensable tools in modern research settings.
- Advantages and Limitations: While the strengths of SPR technology are evident, such as real-time and label-free analysis, it's equally important to acknowledge the challenges, including costs and the complexity of data interpretation.
- Future Outlook: Innovations on the horizon, particularly in integration with microfluidic systems and adaptations for high-throughput applications, foreshadow exciting developments that will likely reshape the landscape of SPR technologies.
Call for Further Research and Exploration
Moving forward, there remains a significant need for further exploration in the realms of surface plasmon resonance. Several key areas warrant greater attention:
- Innovative Methodologies: As technology evolves, researchers should investigate new methodologies that enhance the sensitivity and specificity of SPR instruments.
- Interdisciplinary Collaborations: Effective application of SPR can benefit from collaborations across various scientific domains, fostering insights that may lead to breakthroughs in understanding complex biological interactions or materials science.
- Educational Endeavors: There's also an imperative to raise awareness of SPR technology in academic settings, ensuring emerging scientists and engineers grasp its potential impacts.
- Regulatory Perspectives: Moreover, as applications expand into food safety and environmental monitoring, advocacy for standardized protocols and regulations will be necessary to ensure reliable outcomes and public trust.
In sum, as we continue to peel back the layers of SPR technology, there remains a wealth of knowledge yet to be uncovered. The interplay of fundamental science and practical application continues to pave the way for advancements that can benefit society at large.
Through sustained research and collaboration, the future of SPR holds promise—one that challenges us to think critically and innovatively.