Exploring Electroporation: Methods and Applications
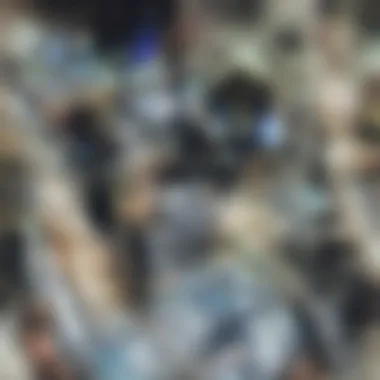
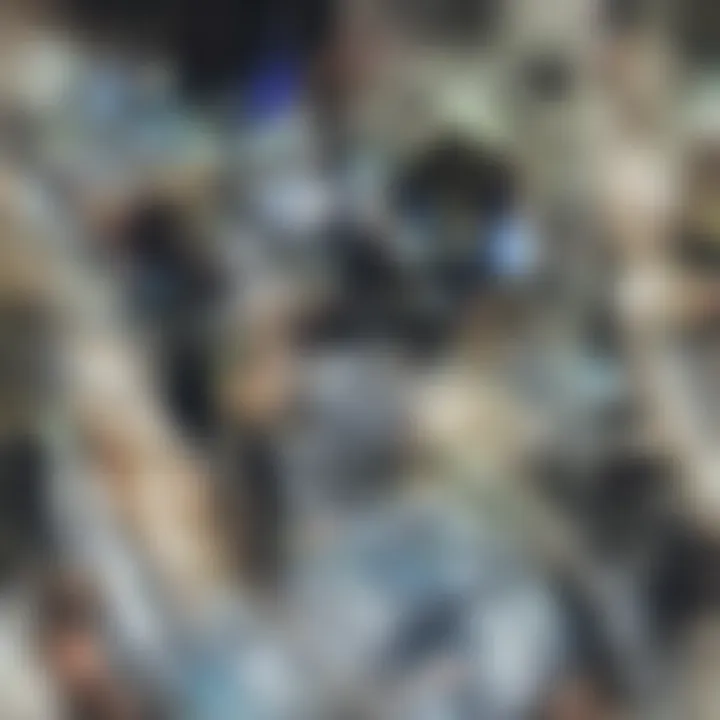
Intro
Electroporation is a pivotal technique in biotechnology that employs electric pulses to facilitate the transfer of molecules into and out of cells. In recent years, this method has gained traction, especially within the realms of gene therapy, drug delivery, and cell transformation. It effectively alters cell membranes, allowing for the introduction of foreign DNA, RNA, or other therapeutic agents. The implications of this technique are profound, influencing contemporary research and clinical applications.
Background and Context
Overview of the Research Topic
The principle of electroporation originated from early studies on how electric fields affect cell membranes. By applying short, intense bursts of electrical energy, researchers discovered that the permeability of cell membranes increases. This temporary increase allows larger molecules, such as plasmids or certain drugs, to enter cells that would otherwise be impermeable to them. Electroporation can be employed in a variety of settings including laboratory research, clinical applications, and agricultural modifications, demonstrating its versatility.
Historical Significance
The concept of electroporation was first introduced in the late 20th century. Scientists like Neumann and Rosenberg conducted ground-breaking work that laid the foundation for what would become a critical tool in molecular biology. The initial experiments involved bacteria, but the methodology quickly expanded to encompass a wide range of cell types, including mammalian cells. Over the decades, continuous improvements in electroporation technology have enhanced its efficiency and safety.
Key Findings and Discussion
Major Results of the Study
Numerous studies have demonstrated that electroporation is a highly effective method for gene transfer both in vitro and in vivo. Research indicates that electroporation can achieve transfer efficiencies exceeding that of traditional methods such as lipofection or viral transduction. The efficiency is related to various factors, including the intensity and duration of the electrical pulse, the size of the target molecules, and the type of cells being treated.
Detailed Analysis of Findings
The detailed analysis reveals several factors that influence the effectiveness of electroporation:
- Pulse Characteristics: The duration, shape, and intensity of the electrical pulse play crucial roles in achieving optimal electroporation effects. Short, high-voltage pulses are often the most successful for inducing membrane permeability.
- Cell Type: Different cell types respond variably to electroporation. For instance, plant cells often require different parameters compared to mammalian cells.
- Molecular Size: Larger molecules like plasmids generally have lower transfer efficiency compared to smaller ones. Researchers are investigating methods to improve uptake of larger compounds.
Electroporation stands at the forefront of modern biotechnology, paving the way for new therapies and advancements.
Applications of electroporation span various fields. In gene therapy, it serves as a delivery mechanism for therapeutic genes, allowing for targeted treatment of genetic disorders. In the context of drug delivery, electroporation can enhance the uptake of drugs in resistant tumors, providing a means to overcome challenges in treating certain cancers. Finally, in cell transformation, electroporation aids in the production of genetically modified organisms, crucial for agriculture and research.
Understanding the mechanisms and applications of electroporation is essential for advancing biotechnological innovations. Its relevance cannot be understated, offering insights that enhance both scientific knowledge and practical applications in medicine and agriculture.
Preamble to Electroporation
Electroporation serves as a critical technique in modern biotechnology, primarily due to its efficiency in introducing molecules into cells. This process has transformed several fields, especially in gene therapy and drug delivery systems. With more precise and controlled methodologies being developed, electroporation is becoming increasingly relevant in various experimental settings. Researchers appreciate its potential to enhance cellular uptake, making it invaluable for numerous applications in life sciences.
Definition and Historical Context
Electroporation refers to the method of applying short bursts of electric field strength to create temporary pores in cell membranes. The concept was first introduced in the 1980s when scientists began harnessing electrical pulses to facilitate molecular entry into cells, significantly changing genetic engineering's legislative landscape. Historic experiments demonstrated how DNA could be introduced into bacterial cells. As research progressed, the method expanded to include mammalian cells, paving the way for therapeutic advancements.
Electroporation in Contemporary Research
In recent years, the scope and applications of electroporation have diversified significantly. Current research explores various settings, including:
- Gene Therapy: Electroporation enables the transfection of therapeutic genes, addressing genetic disorders.
- Drug Delivery: Researchers utilize electroporation to deliver drugs directly into targeted cells, enhancing uptake and effectiveness.
- Cancer Treatment: The technique allows for the targeted delivery of chemotherapeutic agents, potentially reducing side effects while increasing efficacy.
Additionally, advancements in microfluidics and the development of novel pulsing techniques have led to improved efficiency and precision in electroporation protocols. As we continue to explore the mechanisms and variations of electroporation, its implications for clinical applications remain profound.
"Electroporation is not merely a technique; it represents a paradigm shift in cellular interaction and treatment methodologies."
Fundamental Principles of Electroporation
Electroporation is a critical technique in biotechnology, particularly because it enables the transfer of macromolecules across cell membranes. Understanding the fundamental principles behind electroporation is essential for the successful application of this method in various scientific fields. These principles lay the groundwork for optimizing electroporation techniques and improving efficiency in practical uses such as gene therapy, drug delivery, and cell transformation.
Mechanisms of Cell Membrane Permeabilization
Cell membranes are selectively permeable barriers. When exposed to a strong electrical field, the structure of the lipid bilayer changes temporarily. This process is known as membrane permeabilization. The effect occurs due to the formation of pores in the membrane, which allow for the passage of large molecules, such as DNA or therapeutic drugs, that would otherwise not penetrate the cell membrane.
This change in permeability happens through several mechanisms:
- Electroporation effectively creates pores through the rearrangement of lipids in the cell membrane.
- Duration and intensity of the electrical pulse affect pore formation significantly. Short pulses may result in transient pores, whereas longer exposures can compromise cell integrity.
- Molecular dynamics play a role. Depending on the size and charge of the molecule, the transport across the membrane can vary widely.
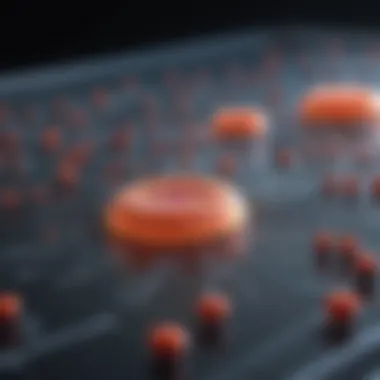
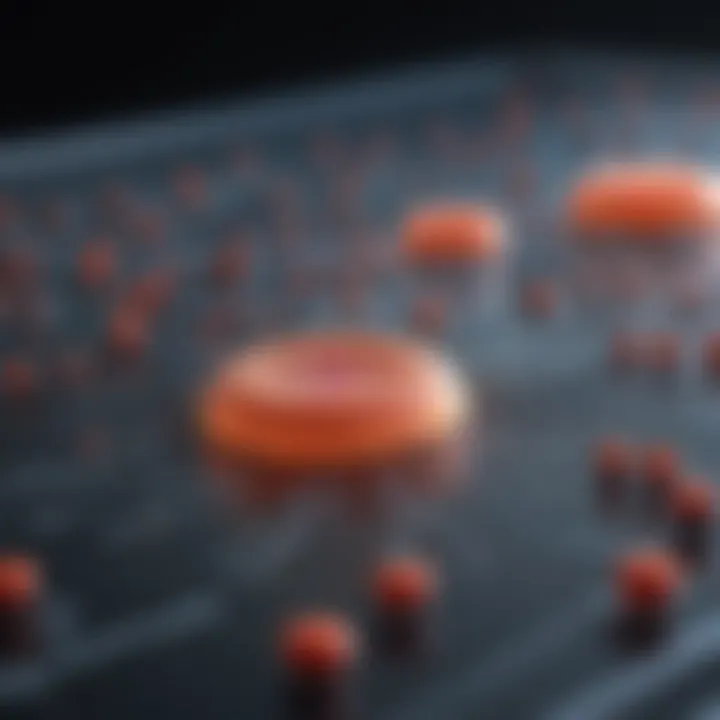
The ability to control these parameters ensures that electroporation can be tailored for specific applications, providing significant benefits in research and therapeutic settings. As such, careful consideration of these mechanisms is crucial for anyone working with electroporation.
Role of Electrical Parameters
The role of electrical parameters in electroporation is pivotal. Certain fundamental aspects need to be examined to understand how to maximize efficacy.
- Pulse Duration: The length of the electric pulse determines the time of membrane exposure to the field. Short, intense pulses increase pore formation but too much exposure can lead to cell death.
- Field Strength: The strength of the electrical field directly correlates with pore development and size. A stronger field can induce more pores, yet if it is excessively high, it may cause irreversible damage to the cell, leading to decreased viability.
- Frequency of Pulses: The frequency can affect the buildup of electric field strength within the cellular environment, impacting how effectively molecules are introduced into cells.
- Shape and Size of Electrodes: The geometry of the electrodes also influences how electric fields are distributed across the cell culture. Proper design leads to uniform electroporation, ensuring all targeted cells receive the intended effect.
Understanding and optimizing these electrical parameters allow researchers to enhance the overall effectiveness of electroporation techniques. This knowledge ultimately contributes to more successful applications in biotechnology.
In summary, the fundamental principles of electroporation are essential for advancing various biotechnological processes. A thorough grasp of membrane dynamics and electrical parameters will enhance the application of electroporation across diverse scientific fields.
Types of Electroporation Techniques
Understanding the various techniques of electroporation is critical in biotechnology. Each method offers unique advantages and limitations that can make a significant impact on experimental outcomes. Selecting the appropriate technique for a specific application can enhance efficacy and optimize results in tasks such as gene delivery or cell transformation. This section outlines the three principal techniques: standard electroporation, microfluidic electroporation, and nanosecond pulsed electroporation.
Standard Electroporation
Standard electroporation is perhaps the most common technique used in research and clinical applications. It involves applying a high-voltage electrical field to target cells, facilitating the uptake of foreign molecules through transient pores created in the cell membrane. This method is particularly useful for larger quantities of cells and the introduction of plasmid DNA.
Benefits of standard electroporation include:
- Versatility: Effective with various cell types, including bacteria, yeast, and mammalian cells.
- Established Protocols: Extensive research has led to well-defined protocols and parameters that simplify application in laboratory settings.
- High Efficiency: Capable of achieving relatively high transfection rates compared to some other methods.
However, there are considerations to be aware of:
- Cell Viability: The electroporation process can lead to reduced cell survival if not optimized correctly.
- Electroporation Time: Adjusting the duration of the pulse is crucial for maximizing efficiency without harming the cells.
Microfluidic Electroporation
Microfluidic electroporation represents a more recent advancement in the field. It involves the manipulation of fluids at the microscale, allowing for the precise control of the electroporation process. This technique offers several benefits:
- Higher Throughput: The ability to treat multiple samples simultaneously increases experimental efficiency.
- Reduced Reagent Volume: Utilizing smaller amounts of substances, which lowers costs and minimizes waste.
Nonetheless, this technique requires specialized equipment, and its complexity can lead to higher implementation learning curves for new researchers.
Considerations include:
- Equipment Cost: Financial investment in microfluidic devices may limit accessibility for some laboratories.
- Processes Optimization: Requires careful calibration of the microfluidic system to achieve desired outcomes.
Nanosecond Pulsed Electroporation
Nanosecond pulsed electroporation is an emerging technique gaining attention due to its unique process. Unlike traditional electroporation, it uses ultra-short electrical pulses, resulting in less extensive cellular damage. This approach is powerful for research involving sensitive cells or tissues.
Key features include:
- Cell Integrity: Minimal harm to cells, improving viability and functionality post-treatment.
- Rapid Process: Faster than conventional electroporation methods, which is beneficial in high-throughput scenarios.
Nevertheless, there are factors to contemplate regarding its practical application:
- Research Maturity: Still in development stages, requiring additional validation in various biological contexts.
- Complexity: The technique may necessitate sophisticated equipment and knowledge not available in all labs.
"Choosing the right electroporation technique is crucial for successful experiments in biotechnology. Each has unique benefits and challenges that must be carefully evaluated."
Optimization of Electroporation Conditions
Optimizing electroporation conditions is crucial for enhancing the delivery of molecules into cells. This phase of experimentation directly impacts the efficiency of electroporation techniques. Given the variable nature of cells and external conditions, careful adjustments can lead to significant improvements in outcomes. The primary goal is to maximize the permeabilization of cell membranes while minimizing toxicity, ensuring that cells remain viable post-treatment.
Factors Influencing Efficacy
Several factors influence the efficacy of electroporation. These elements include:
- Electrical parameters: Voltage, pulse duration, and number of pulses play a significant role in determining how effective electroporation will be. Higher voltages may increase permeabilization but also raise the risk of cell death.
- Cell type: Different cells exhibit varied responses to electroporation. For instance, lymphocytes are more susceptible to permeabilization compared to fibroblasts.
- Cell growth phase: Cells in the logarithmic growth phase tend to be more receptive to electroporation compared to those in stationary phase.
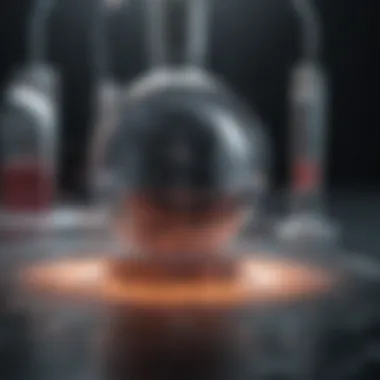
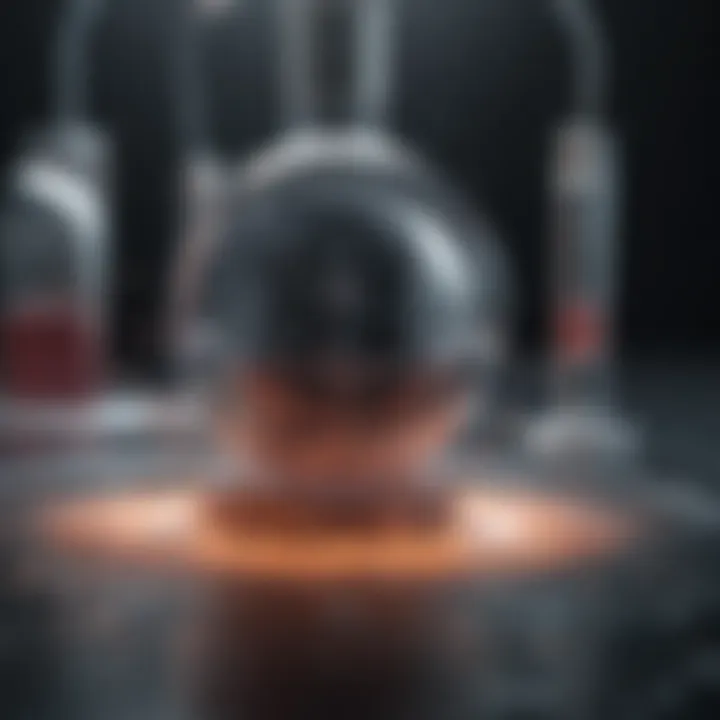
In evaluating these factors, researchers strive to find a balance that enhances delivery without harming cellular integrity.
Buffer and Cell Type Considerations
The choice of buffer and the specific cell types employed also play critical roles in optimizing electroporation conditions. Buffers are essential as they maintain osmotic balance during the process and can influence the electrical conductivity.
- Buffer composition: Phosphate-buffered saline (PBS) is common, but alternatives, including sucrose or glucose, may improve cell viability. Each buffer affects how cells interact with electric fields, therefore must be chosen wisely.
- Cell type considerations: Adjusting conditions for different cell lines is necessary. For instance, mammalian cells may require distinct treatment settings compared to bacterial cells.
"The right conditions can drastically change the effectiveness of electroporation, making it essential to tailor settings to each unique scenario."
By carefully considering factors such as electrical parameters and buffer selection, researchers can enhance the potential of electroporation in biotechnological applications.
Applications of Electroporation
Electroporation is a significant technique in biotechnology with diverse applications. Its ability to increase cell membrane permeability through controlled electrical pulses allows for various biotechnological interventions. The profound impact of electroporation can be observed particularly in gene therapy, drug delivery, and cell transformation. Understanding the applications of electroporation not only sheds light on its practical uses but also emphasizes its importance in addressing complex medical and scientific challenges.
Gene Therapy Techniques
Gene therapy leverages electroporation to introduce therapeutic nucleic acids into target cells. This method provides a more efficient delivery system compared to traditional viral vectors. Electroporation facilitates the uptake of plasmids, siRNA, and mRNA into cells while preserving the integrity of the cellular structure, reducing the risk of cytotoxicity. The electrical pulses create transient pores in the cellular membrane, allowing large molecules to pass through, an essential capability in the treatment of genetic disorders.
Given the complexity of diseases like cystic fibrosis or certain types of cancers, utilizing electroporation is vital for the direct approach in delivering corrective genes. Furthermore, the customization of electroporation protocols, such as adjusting pulse duration and field strength, allows for targeted treatment optimization based on cell type and desired outcomes. This flexibility enhances the therapeutic effectiveness while minimizing potential side effects.
Drug Delivery Systems
In the realm of drug delivery, electroporation offers a new frontier in improving the efficiency of pharmaceuticals. By using electroporation, drugs can be delivered directly into tissues, bypassing traditional systemic administration routes, which often lead to reduced efficacy or adverse effects. This technique is particularly advantageous for macromolecules, such as proteins and peptides, which typically struggle to cross lipid membranes.
The application of electroporation facilitates localized delivery, thus enhancing the therapeutic concentration at the site of action. This precision is especially critical in cancer therapies, where the goal is to maximize drug uptake in tumor cells while reducing exposure to healthy surrounding tissues. Additionally, research into optimizing electroporation parameters for different drug types shows promising potential to tailor therapies for individual patient needs, eventually leading to more successful treatment outcomes.
Cell Transformation in Research
Cell transformation is foundational in biotechnological research. Electroporation plays an essential role in the genetic modification of cells, allowing researchers to introduce new genes and study their effects. This capability is particularly crucial in producing genetically modified organisms for agricultural research or understanding gene function in cell models.
Electroporation is employed in creating stable cell lines, where researchers can maintain specific genetic traits over generations. Moreover, this technique supports high-throughput screening of various gene functions and drug responses, facilitating advancements in cell biology and pharmacology. The ability to manipulate cellular genetics speeds up the discovery of critical therapeutic targets and aids in the development of novel treatments.
Electroporation stands at the intersection of innovation and application in biotechnology, significantly enhancing methods in gene therapy, drug delivery, and cellular research decisions.
In summary, the applications of electroporation demonstrate its versatility and potential for transformative impact in the fields of medicine and research. The meticulous deployment of electroporation techniques ensures a promising future in biotechnology advancements.
Challenges in Electroporation Research
The exploration of challenges in electroporation research is vital to understanding its practical applications and limitations in biotechnology. As this technique evolves, researchers face various obstacles that can hinder its effectiveness and reliability. Addressing these challenges is crucial for advancements in fields such as gene therapy, drug delivery, and cellular transformation.
Among the primary challenges are cell viability and survival rates, which are critical indicators of the procedure's success. When cells are subjected to electrical pulses, they may experience stress that compromises their structural integrity and functionality. This increases the risk of cell death, which can significantly impact the outcomes of the experiment or treatment. Therefore, optimizing electroporation protocols to minimize cell damage while maximizing molecule transfer is essential.
Another challenge lies in the inefficiencies of current protocols. Many established techniques may have inconsistent outcomes or necessitate long optimization periods. Inefficiencies can lead to variability in results and make it difficult for researchers to reproduce findings. This inconsistency presents a barrier to the widespread adoption of electroporation in clinical and research settings.
In summary, fully understanding and overcoming these challenges will enhance the utility of electroporation techniques in biotechnology.
Cell Viability and Survival Rates
Cell viability is a crucial aspect of electroporation research. The application of electrical pulses aims to increase the permeability of the cell membrane, enabling the introduction of various molecules like DNA or therapeutic agents. However, this process can induce significant stress on the cells, leading to changes in membrane integrity and potentially triggering apoptosis or necrosis.
To illustrate the importance of cell viability:
- High cell viability ensures that the desired biomolecules are effectively introduced into living cells without causing detrimental effects.
- Low survival rates can result from suboptimal electrical parameters or improper cell handling, limiting the efficiency of molecule uptake and altering experimental results.
Therefore, researchers must carefully tailor their electroporation protocols to balance between maximizing permeability and maintaining cellular health. Various studies have indicated that employing smaller or shorter electrical pulses can enhance cell survival without compromising the permeabilization efficiency.
Inefficiencies in Protocols
The inefficiencies found within electroporation protocols present a significant barrier to their application in real-world settings. Many established protocols rely on trial and error, often requiring extensive optimization to achieve consistent results. This can consume valuable time and resources, especially in high-stakes environments like clinical research.
Common issues include:
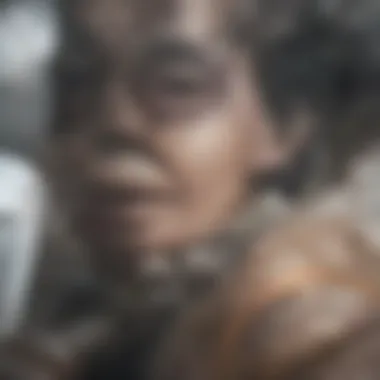
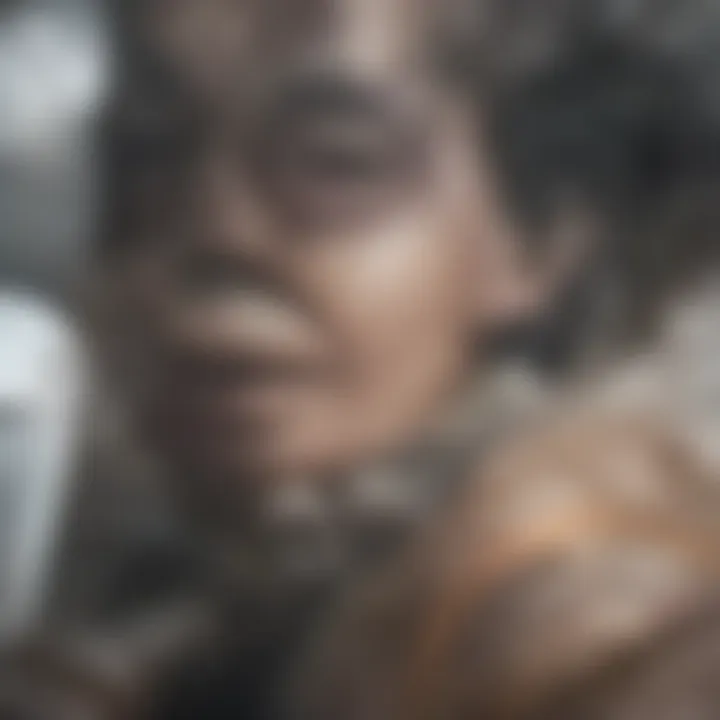
- Variability in experimental outcomes, which can arise from differences in cell types, electrical settings, or equipment.
- Time-consuming optimization processes that require repeated testing and adjustments, delaying project timelines.
To address these inefficiencies, researchers are developing standardized guidelines and protocols that may facilitate more predictable results across different studies. Additionally, advancements in technology, such as automated electroporation devices, could streamline processes and enhance reproducibility.
"Addressing the challenges in electroporation research is pivotal for its future success in biotechnology applications."
Ethical Considerations in Electroporation
As biotechnology progresses, the ethical considerations surrounding emerging techniques like electroporation become increasingly critical. This section delves into the specific elements that make the ethical landscape of electroporation paramount, focusing on regulatory frameworks and the public's acceptance of this technology.
Regulatory Frameworks
The introduction of any novel biotechnological method, including electroporation, often requires a robust regulatory framework. Such frameworks ensure the safety and efficacy of the technology while protecting human and environmental health. In many countries, regulatory bodies like the U.S. Food and Drug Administration (FDA) and the European Medicines Agency (EMA) play vital roles in assessing biotechnological innovations.
The guidelines set forth by these agencies cover various facets:
- Safety Assessments: Establishing the safety of electroporation techniques on human cells before any clinical trials.
- Efficacy Verification: Demonstrating that electroporation achieves its intended purpose effectively.
- Ethical Trials: Ensuring that all clinical trials follow ethical guidelines, such as obtaining informed consent from all participants.
These frameworks also aim to balance innovation with public health needs. As new products and applications emerge, regular updates to regulations are necessary to adapt to the evolving landscape of biotechnology.
Public Acceptance and Perspectives
Public perception plays a crucial role in the development and implementation of electroporation technologies. It is essential to gauge the societal acceptance of procedures that manipulate cell membranes for various applications, such as gene therapy. Public concern often gravitates toward potential risks, ethical implications, and benefits associated with biotechnology.
Several factors influence public acceptance:
- Transparency: Clear communication regarding the mechanisms and applications of electroporation helps address doubts and promotes understanding.
- Education: Informing the public about the benefits of electroporation in medical advancement can foster a positive view.
- Stakeholder Engagement: Involving community members and stakeholders in discussions can enhance trust.
"Public trust in biotechnology is foundational to its success and integration into healthcare systems."
While debates and discussions continue, it is increasingly essential to address these perspectives through educational initiatives and ethical dialogues. By doing so, biotechnology can progress with informed consent, fostering an environment where electroporation and similar techniques can flourish responsibly.
Future Directions of Electroporation Research
The future of electroporation research holds significant promise for advancing biotechnology applications. The ongoing exploration of electroporation techniques aims to enhance efficiency and effectiveness across various fields. Researchers are investigating new parameters and technologies that can push the boundaries of what is currently achievable. This section delves into emerging technologies and potential innovations in methodologies that could reshape the landscape of this critical technique.
Emerging Technologies
The evolving landscape of technology continually influences electroporation research. Recent innovations include the incorporation of more precise electrical control systems and real-time monitoring. These advances enable scientists to customize treatment conditions for specific cell types, enhancing the overall efficacy of electroporation.
- Microelectrode Arrays: These tools can deliver targeted pulses of electricity, which improves precision in applications such as gene therapy and drug delivery.
- Smart Electroporation Systems: These systems utilize artificial intelligence to optimize parameters dynamically, allowing for adjustments during the process based on feedback from cell responses.
- Integration with Other Methods: Combining electroporation with techniques like CRISPR and RNA interference shows potential for improving gene editing outcomes and therapeutic effectiveness.
These emerging technologies not only enhance the delivery of molecules but also facilitate the use of electroporation in a broader range of applications.
Potential Innovations in Methodologies
As researchers push the envelope of what is possible with electroporation, several innovative methodologies are under exploration. These innovations could significantly impact the way biotech applications are approached.
- Optimized Pulse Formats: Investigating different pulse waveforms could lead to better cell membrane permeability without compromising cell viability.
- Temperature-Tailored Protocols: The exploration of how temperature affects electroporation opens doors to new protocols that could amplify results.
- Non-traditional Cell Types: Researchers are now examining the effects of electroporation on non-mammalian cells, which could expand biotechnological applications significantly.
"Innovation in electroporation methodologies can usher in a new era for gene therapy and personalized medicine."
These methodologies strive for higher efficacy while maintaining cell integrity, which is crucial for clinical applications. Further exploration and validation of these techniques are essential for unlocking the full potential of electroporation in biotechnology.
Closure
In summary, the conclusion of this article encapsulates the critical insights into electroporation's mechanisms and applications within biotechnology. Electroporation stands as a transformative technique that has expanded the boundaries of what is achievable in genetic research and therapeutic applications. A clear understanding of the principles guiding electroporation, the intricacies of various techniques, and the potential impacts on gene therapy, drug delivery, and cell transformation offer significant advantages to researchers and practitioners in the field.
Summary of Key Insights
Electroporation enables the effective transfer of biomolecules across cell membranes using short electrical pulses. The main points discussed in this article include:
- Mechanisms of Cell Membrane Permeabilization: It has been established how electrical pulses can disrupt lipid bilayer integrity, allowing for enhanced molecule uptake.
- Applications in Biotechnology: Key applications such as gene therapy and drug delivery systems demonstrate electroporation’s vast potential. It serves as an effective method to introduce therapeutic substances into target cells.
- Challenges and Ethical Considerations: While electroporation shows great promise, ongoing challenges related to cell viability and protocol inefficiencies must be addressed. Additionally, ethical considerations surrounding the use of this technology underscore the importance of regulatory frameworks.
"The impact of electroporation is profound, potentially redefining therapeutic approaches across multiple disciplines of health and research."
Final Thoughts on Electroporation
As we look to the future, the ongoing evolution of electroporation technology may lead to significant enhancements in its efficacy and application. Advancements in methodologies, combined with an emphasis on ethical considerations, will ensure that electroporation continues to play a pivotal role in biotechnology. For scholars and professionals, grasping the relevance and capabilities of electroporation not only informs current practices but also inspires innovation in addressing future challenges in cellular biology. The importance of electroporation cannot be understated; it is a fundamental tool that bridges the gap between theory and application in modern biotechnology.