Understanding Solar Cell Voltage: A Technical Overview
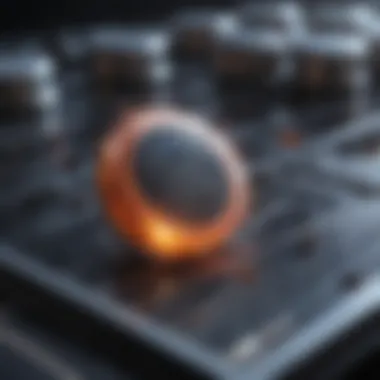
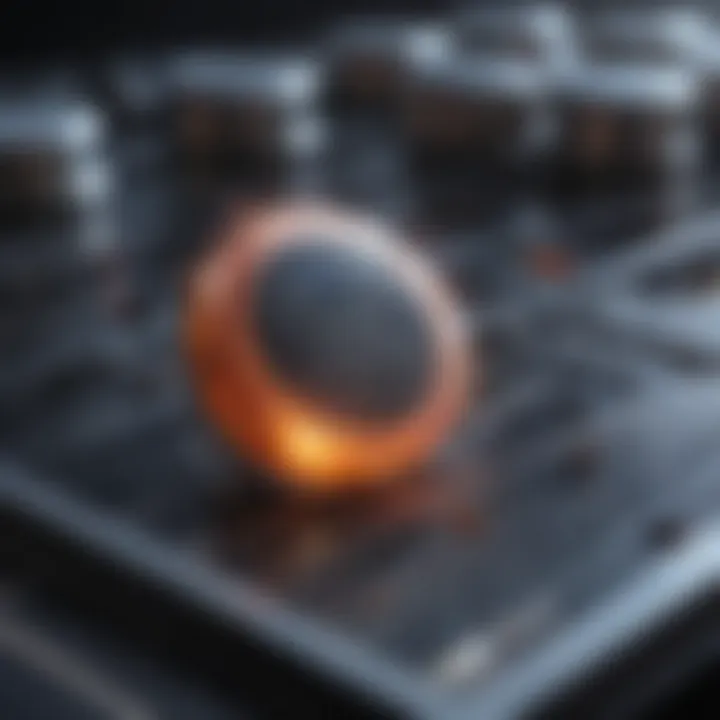
Intro
In recent years, solar energy has emerged as one of the most promising solutions for sustainable power generation. Central to this technology is the concept of solar cell voltage. Understanding how voltage operates within solar cells is critical for optimizing their performance and expense. This article provides a comprehensive technical overview of solar cell voltage, delving into its foundational principles, the factors that influence it, and the methods for measuring it. By exploring these elements, we can grasp how solar voltage plays a role in the efficiency and viability of solar energy systems.
Background and Context
Overview of the Research Topic
Solar cells convert sunlight into electricity, operating with a basic principle of photovoltaic effect. The voltage generated by solar cells is essential for determining the power output of the solar energy system. The efficiency at which these cells convert light energy into electrical energy depends on various factors, such as materials used, temperature, and even the angle of sunlight.
Historical Significance
The development of solar technology has evolved significantly since its inception. The first practical photovoltaic cell was developed in 1954 at Bell Labs. Initially, these cells had very low efficiency, but advancements in technology have allowed for greater efficiency and lower cost. The historical progression of solar cell technology has led to a better understanding of the voltage characteristics of these devices. Each advancement has contributed to improvements in the design and materials of solar cells, affecting their voltage output.
Key Findings and Discussion
Major Results of the Study
Research shows that the voltage output of solar cells is influenced by several key factors:
- Temperature: The performance and efficiency decrease as temperature rises, affecting the voltage output.
- Material Composition: Different semiconductors like silicon, cadmium telluride, and perovskite have unique voltage characteristics, impacting their suitability in various applications.
- Light Intensity: Higher light exposure generally increases the voltage output but has limits due to saturation.
Detailed Analysis of Findings
Analysis reveals that measuring solar cell voltage accurately is crucial. Common methods involve using voltmeters to assess direct output, as well as more complex instrumentation in lab settings. The interplay between environmental conditions and technological advancements influences the voltage capabilities of solar devices. Note that consistent research has highlighted the emerging technologies, such as bifacial solar cells and quantum dot solar cells, which offer potential enhancements in voltage outputs.
"Understanding the factors that influence solar cell voltage is crucial for future advancements in solar energy systems. This knowledge can lead to improvements in design and efficiency."
Preface to Solar Cell Voltage
Understanding solar cell voltage is essential for anyone engaged in solar technology, from students to professionals. This section aims to unpack the concepts surrounding solar cell voltage, eliminating misconceptions and presenting accurate information. The implications of solar cell voltage extend far beyond theoretical discussions; they influence practical aspects of solar energy systems, affecting efficiency and overall performance.
Defining Solar Cell Voltage
Solar cell voltage refers to the electrical potential difference produced by solar cells when they convert light energy into electricity. This conversion process is governed by the photovoltaic effect, where photons striking the solar cell generate electron-hole pairs. The resulting movement of these charge carriers establishes a voltage across the junction of the solar cell. For efficient energy production, an optimal voltage level is crucial. Typically, the open-circuit voltage—the maximum voltage available from a solar cell under open-circuit conditions—serves as a primary metric for assessing cell performance.
Voltage is influenced significantly by various factors, including light intensity, material properties, and temperature. Understanding these dynamics is vital for optimizing solar energy systems in real-world applications.
Importance in Solar Technology
The importance of solar cell voltage in solar technology cannot be overstated. Firstly, voltage directly correlates with the energy output of solar panels. Higher voltages can improve the overall efficiency of power systems, allowing for better energy capture and utilization.
Additionally, solar cell voltage plays a critical role in determining the compatibility of solar panels with various inverters and storage systems. Mismatched voltage levels can lead to inefficiencies or even damage in electrical circuits. Properly matching voltage levels ensures that solar energy systems function as intended, maximizing return on investment.
In the context of solar technology advancement, understanding voltage behavior under different operational conditions can lead to innovations in cell design and material use. Thus, studying solar cell voltage is not only vital for current applications but also crucial for future enhancements in solar technology.
"Solar cell voltage is a key determinant of how effectively we can harness the sun's energy. It affects everything from efficiency to compatibility with existing systems."
Fundamentals of Solar Cell Operation
Understanding the fundamentals of solar cell operation is crucial to grasp how solar energy is converted into usable electrical power. This section focuses on two key components: the photovoltaic effect and the various types of solar cells. Each component plays a significant role in determining the voltage output and efficiency of solar systems.
Photovoltaic Effect Explained
The photovoltaic effect is the foundation of solar technology. It describes the process by which solar energy is converted into electricity. When sunlight strikes a solar cell, its energy excites electrons in the material, allowing them to move freely. This movement creates a flow of electrical current. Essentially, the photovoltaic effect is what enables solar cells to harness sunlight and convert it into useful power.
Understanding this effect helps elucidate how different materials and designs influence voltage production. For instance, the choice of semiconductor material affects the efficiency with which sunlight is converted into electricity. This knowledge is essential for anyone looking to optimize solar cell performance and increase energy output.
Types of Solar Cells
Solar cells come in various types, each with its own characteristics and applications. Understanding the differences among these types is vital for selecting the appropriate cell for specific needs.
Monocrystalline
Monocrystalline solar cells are made from a single crystal structure, which gives them a uniform appearance. One of the key characteristics of monocrystalline cells is their high efficiency, often exceeding 20%. This makes them a beneficial choice for applications where space is limited, such as residential rooftops.
Their unique feature is the high pure silicon content, which allows for better electron flow. However, this advantage comes with a higher production cost. Thus, while they dominate in efficiency, cost considerations play a vital role in their overall adoption.
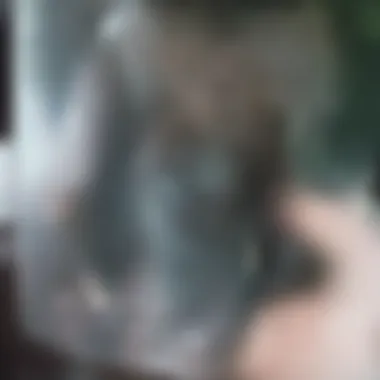
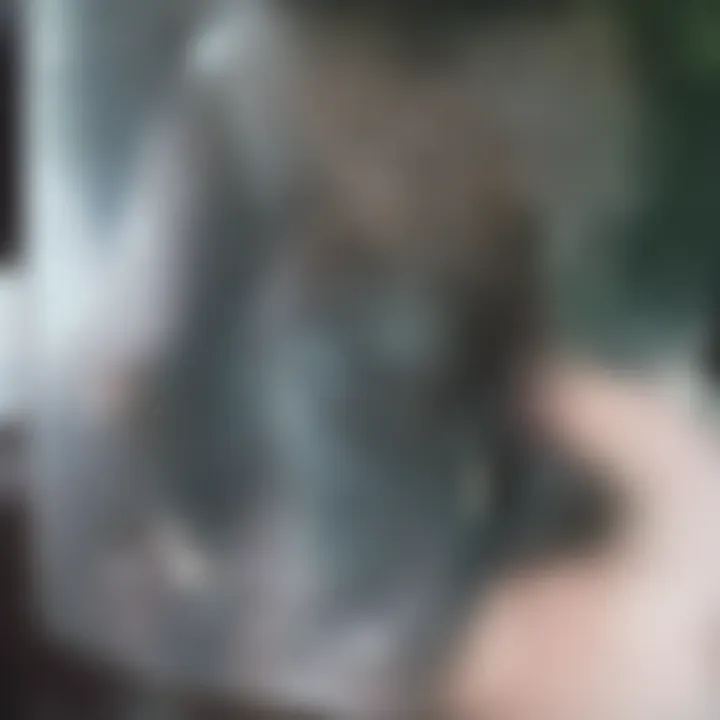
Polycrystalline
Polycrystalline solar cells are produced from multiple silicon crystals. This results in a less uniform appearance with a blue hue. The key characteristic of polycrystalline cells is that they are generally less expensive to produce compared to monocrystalline cells. This makes them a popular choice for large installations, like solar farms, where space is less of a concern.
A unique feature of polycrystalline cells is their manufacturing process, which is simpler and less energy-intensive, leading to lower costs. However, they are typically less efficient, often around 15-17%. This means that while they are cost-effective, they require more space to produce the same amount of power as monocrystalline cells.
Thin-film
Thin-film solar cells are made by depositing semiconductor materials in a thin layer on various substrates. This design allows for flexibility and versatility in application. The key characteristic of thin-film cells is their lightweight and flexible nature, making them ideal for unconventional surfaces, such as building-integrated photovoltaics.
A unique feature of thin-film technology is its ability to perform better in low-light conditions. However, the efficiency of thin-film cells is generally lower, around 10-12%. This warrants consideration regarding the tradeoffs between flexibility and power output in design decisions.
Factors Influencing Solar Cell Voltage
Understanding the factors that influence solar cell voltage is crucial for enhancing the performance and efficiency of solar energy systems. Solar cell voltage is not an isolated characteristic; it is strongly affected by various internal and external elements. Each factor can significantly affect the output voltage and overall efficiency of solar panels.
Material Properties
Bandgap Energy
Bandgap energy refers to the energy difference between the valence band and the conduction band in a semiconductor material. This property determines how much energy is needed to excite electrons to create an electric current. A key characteristic of bandgap energy is its role in defining the efficiency of a solar cell. Different materials have unique bandgap energies, which makes it essential to choose the right one for optimal performance in solar applications.
Choosing semiconductors with appropriate bandgap energy can enhance voltage output. For instance, silicon, which has a bandgap energy of about 1.1 eV, is widely used for its balance of efficiency and cost. However, there are trade-offs. A higher bandgap material may have better efficiency under certain conditions, but it may not absorb lower energy photons effectively. This feature can lead to a reduction in power generation under various light conditions, making it crucial to consider the specific application and environment of the solar cells.
Doping Levels
Doping levels refer to the intentional introduction of impurities into semiconductor materials to modify their electrical properties. Doping is critical for controlling the conductivity of the material. One of the prominent characteristics of doping levels is how it can enhance carrier concentration, thus improving the overall performance and efficiency of solar cells.
Using n-type and p-type dopants can help create a p-n junction, which is vital for voltage generation in photovoltaic cells. The unique feature of doping levels is that it allows for fine-tuning the solar cell's response to light and temperature. However, excessive doping can lead to recombination losses, reducing efficiency. Careful consideration of doping levels is needed to achieve the desired balance for optimal voltage output.
Temperature Effects
Temperature plays a significant role in the performance of solar cells. As the temperature increases, the voltage output of solar cells generally decreases. This phenomenon can be explained by the increase in carrier concentration at higher temperatures, which leads to more recombination events. Thus, while solar cells may produce more current in higher temperatures, the resulting voltage output often diminishes.
Additionally, thermal expansion can cause physical stresses in the materials, potentially leading to efficiency losses or durability issues. Understanding these temperature effects is key for system design, notably in ensuring that solar power systems are properly rated for thermal variations in their operating environments.
Light Intensity and Spectrum
Light intensity and the spectrum of light can significantly influence solar cell voltage output. The amount of light reaching the solar cell directly correlates with the energy available for conversion into electricity. Under normal conditions, higher light intensity results in increased voltage; however, this relationship isn't linear. As intensity rises, other factors must be considered to prevent possible saturation of the solar cell.
Moreover, light spectrum refers to the range of wavelengths of light. Solar cells respond differently to each wavelength, meaning that the overall performance may vary based on the light conditions. For example, certain materials are better suited for specific wavelengths. Understanding the light spectrum allows for better material selection and optimization of solar cell design to achieve greater efficiency and voltage output.
"The performance of solar cells is intricately linked to a multitude of factors, which justifies the necessity for detailed analysis in solar energy systems."
In summary, these factors—material properties, temperature, and light conditions—make significant contributions to the voltage output of solar cells. A comprehensive understanding of these elements is essential for the advancement and optimization of solar technology.
Measuring Solar Cell Voltage
Measuring the voltage of solar cells is vital to understand their performance and efficiency. Voltage gives insights into how effectively solar cells convert sunlight into electricity. The measurement of solar cell voltage serves various purposes, such as evaluating system performance, identifying potential issues, and optimizing energy output. It is important for both residential and commercial solar applications.
Voltmeters and Multimeters
Voltmeters and multimeters are essential tools for measuring solar cell voltage. A voltmeter measures the potential difference across two points in a circuit, offering an accurate reading of voltage. Multimeters, on the other hand, can measure various electrical parameters including voltage, current, and resistance. They are practical because they combine multiple functions in a single device. Understanding how to use these instruments properly ensures accurate data is collected from solar cells.
Techniques for Accurate Measurement
Accurate measurement techniques are crucial for reliable results. Various methodologies ensure that the obtained readings reflect true voltage levels. Two common techniques are:
Open Circuit Voltage Measurements
Open circuit voltage (Voc) is measured when the solar cell is not connected to any load. This means the circuit is open, allowing the voltage to reach its maximum value. Measuring Voc is a standard practice because it indicates the highest voltage the solar cell can provide under specific conditions.
- Key Characteristic: Voc measures the peak voltage available from a solar cell.
- Benefits: This technique is beneficial as it provides a clear indication of the solar cell's capability without load interference.
- Unique Feature: The most significant aspect of this measurement is its ability to anticipate performance under ideal conditions. However, it does not account for voltage drops during normal operation.
Short Circuit Conditions
Short circuit conditions involve connecting the solar cell directly across a very low resistance. Under these conditions, the current is maximized while the voltage drops to nearly zero. This condition measures the short circuit current (Isc) which shows how much current can be produced.
- Key Characteristic: This technique highlights the current output without load-induced voltage.
- Benefits: It is a popular choice because it helps in understanding how much current a solar cell can deliver when exposed to maximum sunlight.
- Unique Feature: A significant advantage of this measurement is that it allows one to quickly gauge performance. However, it does not provide direct voltage output data, thus limiting its use for efficiency assessments.
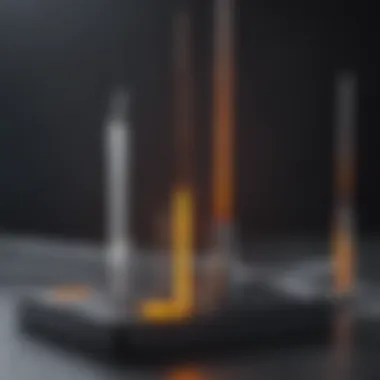
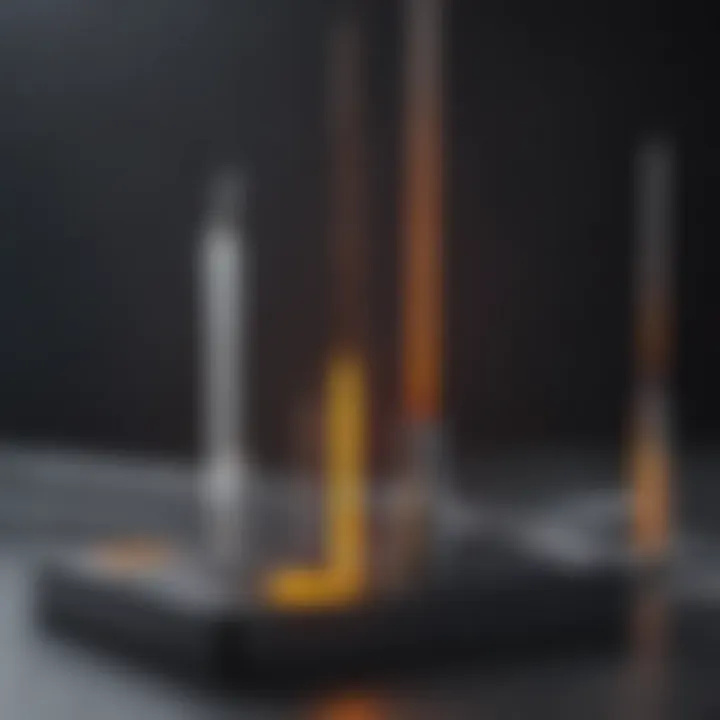
Accurate voltage measurements are essential for determining the performance and longevity of solar cells.
Through these methods, understanding solar cell voltage becomes much clearer. Each technique has its unique advantages, and knowing when to use them can lead to better decision-making in solar energy applications.
Voltage Characteristics Under Varying Conditions
Understanding the voltage characteristics of solar cells under varying conditions is crucial for optimizing their performance. Solar cells do not operate in a vacuum; rather, they are affected by many external factors. The behavior of voltage output plays an essential role in assessing the overall efficiency and effectiveness of solar technology. This section will discuss two key aspects: the I-V characteristics curve and the impact of shadows on voltage output. Both elements are integral for designing and deploying solar power systems successfully.
I-V Characteristics Curve
The current-voltage (I-V) characteristics curve of a solar cell is a fundamental concept in understanding its performance. This curve illustrates how the current output varies with changes in voltage across the solar cell. Typically, the curve forms a distinct shape: a curve starts from zero current at zero voltage, rises sharply then starts to flatten out until it reaches a maximum current output.
In this context, several key points must be noted:
- Short Circuit Current (Isc): This is the maximum current produced when the solar cell is shorted out. It represents the cell's ability to convert sunlight into current.
- Open Circuit Voltage (Voc): This indicates the voltage of the solar cell when no current is flowing. It's a critical parameter as it showcases the maximum voltage output.
- Maximum Power Point (MPP): This is where the product of current and voltage is highest, thus providing the optimal efficiency of the solar cell. Understanding where this point lies on the curve is vital for system design and efficiency optimization.
By analyzing the I-V characteristics curve, engineers can make informed decisions on system configurations, ensuring that the design operates near the MPP for prolonged periods, optimizing energy harvest.
Impact of Shadows on Voltage Output
Shadows pose a significant challenge in solar energy generation. The impact of shading on voltage output can be profound, often leading to reduced system performance. When a solar cell is partially covered by shadow, it affects how light reaches the cell, directly causing a drop in its voltage output.
Here are some considerations regarding the impact of shadows:
- Voltage Drop: Even small shadows can cause substantial voltage drops. This reduction is not linear. A small amount of shading can lead to a disproportional decrease in voltage and current output.
- Mismatched Cells: In a solar array consisting of multiple cells, shading can cause mismatched performance. If only some cells are shaded, they may underperform, reducing the overall system output due to series connections in an array.
- Bypass Diodes: To mitigate the effects of shading, bypass diodes can be installed within solar panels. They allow current to bypass shaded cells, thus preserving some performance but still may not totally negate the loss in voltage.
Shadows create a complex interplay of effects in solar cell performance. Understanding and managing them is critical in maximizing efficiency.
In summary, both the I-V characteristics curve and the shadow impact provide essential insights into how solar cells perform under variable conditions. This knowledge is vital for both researchers and practitioners in the solar energy sector to devise strategies that enhance energy capture and utilization.
Efficiency and Performance Metrics
Efficiency in solar energy systems is a critical subject that determines how effectively solar cells convert sunlight into usable electricity. This aspect affects not only the output of energy but also the overall performance of solar installations in various applications. Understanding performance metrics helps in evaluating investments in solar technology and guides adjustments to maximize output. It is essential to grasp these metrics to advocate for solar adoption in residential, commercial, and industrial settings.
Defining Conversion Efficiency
Conversion efficiency is defined as the ratio of the electrical output of a solar cell to the solar energy input it receives. This is typically expressed as a percentage. For instance, if a solar cell can convert 20% of the sunlight hitting its surface into electricity, it is said to have a conversion efficiency of 20%. Factors influencing this measurement include the quality of materials used in the cell, the design architecture, and external conditions such as temperature and light availability.
In practice, conversion efficiency is crucial because it indicates how much of the solar resource is effectively harnessed. Higher conversion efficiencies can lead to reduced space requirements for solar panels and lower costs per watt. Improvements in conversion efficiency are often pursued through advances in technology and material science, such as using multi-junction solar cells that can capture a broader range of the solar spectrum.
Link Between Voltage and Efficiency
Voltage plays an intrinsic role in determining the efficiency of solar cells. Generally, higher voltage output can lead to better overall performance in energy systems. However, this relationship is complex and influenced by several factors, including material composition and cell design. As voltage increases, it can improve power output without a corresponding increase in current, which can reduce thermal losses in solar cells.
Several relationships can be drawn from the interplay between voltage and efficiency:
- Operating Point: The maximum power point of a solar cell is influenced by voltage and stands as a critical factor for drawing optimal energy from the system.
- Load Matching: A higher voltage allows for better matching with grid voltage levels, which is favorable in many solar applications.
- Heat Generation: Higher voltage, combined with lower current, typically generates less heat, thus improving the thermal performance of solar modules.
In summary, understanding the link between voltage and efficiency gives valuable insight into solar energy system design and optimization, underscoring the technical considerations of solar cell deployments.
Challenges in Solar Cell Voltage Optimization
The quest for optimizing solar cell voltage is multifaceted. It encompasses several technical challenges that directly impact the efficiency and longevity of solar energy systems. As solar technology continues to evolve, understanding these challenges becomes essential not only for academics but also for industry practitioners. In this section, we will address two primary aspects: degradation over time and material limitations. Both play a significant role in the effective harnessing of solar energy.
Degradation Over Time
Degradation of solar cells refers to the gradual loss of performance over their operational lifespan. This phenomenon can significantly affect the voltage output, and therefore the overall efficiency, of the solar panels. Factors contributing to degradation include environmental stressors such as UV radiation, temperature fluctuations, moisture ingress, and other chemical interactions within the solar cell's materials.
Research indicates that different types of solar cells have varying degradation rates. For example, monocrystalline cells may withstand certain environmental pressures better than their polycrystalline counterparts. Acoustic techniques, environmental monitoring, and long-term studies are vital to understand specific degradation pathways.
Some of the common degradation mechanisms are:
- Potential Induced Degradation (PID): This occurs due to external voltage differences that promote leakage currents, negatively affecting voltage output.
- Light-Induced Degradation (LID): A temporary reduction in performance observed in some types of solar cells after exposure to sunlight, primarily due to the creation of defects.
- Thermal Cycling: Repeated heating and cooling cycles can create microcracks in the cell structure, leading to voltage drops.
Understanding these degradation processes not only helps in mitigating their effects but also guides the design of more durable solar technologies.
Material Limitations
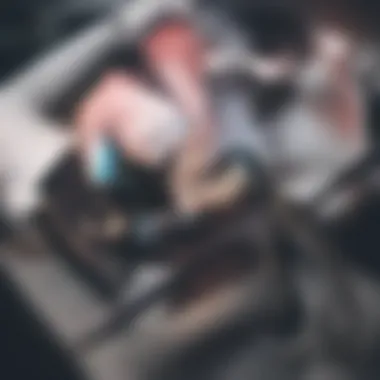
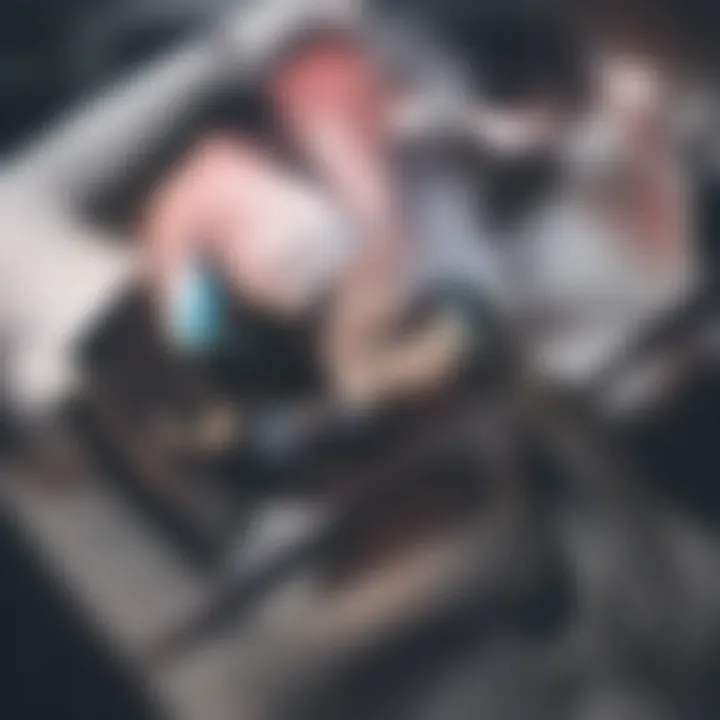
The materials used in the production of solar cells play a crucial role in their voltage efficiency. Each material possesses unique physical and electronic properties, determining its suitability for solar applications. Limitations can arise from:
- Bandgap Energy: The material's bandgap plays a major role in its voltage output. If the bandgap is too wide, the material may not absorb sufficient sunlight, whereas a narrow bandgap may lead to thermal loss.
- Doping Levels: The process of doping, which introduces impurities into the semiconductor material, must be optimized. Inadequate doping can lead to poor charge carrier mobility, adversely affecting voltage generation.
- Materials Quality: Impurities or defects in the crystal structure can significantly hinder performance. Quality control during manufacturing is paramount to minimize these impacts.
Progress in material science aims to reduce these limitations by developing innovative materials, such as perovskite cells, which offer a promising alternative to traditional silicon-based cells. However, the long-term stability and scalability of these materials remain in question.
"To enhance the efficiency of solar cells, continuous research is vital to overcome existing material limitations and address degradation challenges."
In summary, optimizing solar cell voltage is essential yet challenging. Factors like degradation over time and material limitations need to be closely monitored and addressed for effective solar technology deployment. Understanding these challenges can help advance research and innovation in solar energy, leading to improved performance and sustainability in energy generation.
Future of Solar Cell Voltage Research
Research in solar cell voltage is a critical area of study that holds significance for the advancement of solar energy technologies. Understanding the future direction of this research provides insights into potential improvements in efficiency and performance. Researchers focus on overcoming existing limitations, further optimizing voltage, and enhancing the overall productivity of solar cells.
Emerging Technologies
Emerging technologies play a pivotal role in the evolution of solar cell voltage enhancement. Innovations such as bifacial solar cells present opportunities to harness solar energy more efficiently. Bifacial technology collects sunlight from both sides of the panel, increasing overall energy output and voltage stability. Additionally, developments in concentrator photovoltaic technologies allow for better light collection and conversion, which can lead to higher voltage generation under specific conditions.
The integration of artificial intelligence is also becoming prominent in optimizing solar cell performance. AI can analyze data from solar farms to suggest adjustments that maximize voltage output based on real-time environmental data.
Some noteworthy technologies include:
- Perovskite Solar Cells: Known for their high efficiency and potential for lower production costs.
- Multi-junction Cells: Combining different materials to capture various parts of the solar spectrum can improve voltage.
Innovative Materials
Innovative materials are changing the landscape of solar cell development. The introduction of new semiconductor materials, such as perovskites, is particularly promising due to their ability to achieve higher efficiencies and potentially enhance voltage output. These materials can be produced with lower energy inputs compared to traditional silicon-based cells, suggesting a more sustainable production process.
Graphene-based materials are another area of interest. Their electrical properties may lead to improvements in charge carrier mobility, thus influencing solar cell voltage positively. Research is ongoing to discover how these innovative materials can be effectively utilized within existing solar technologies to maximize voltage and efficiency.
Research into hybrid solar cells, which combine organic and inorganic materials, is also gaining traction. These hybrids aim to leverage the strengths of both types of materials, potentially leading to better voltage performance and longevity.
Understanding how to implement these technologies and materials effectively can lead to significant strides in solar voltage research. As sustainability becomes a more pressing issue globally, the evolution of solar technologies will be essential, and voltage optimization is at the forefront of this research.
Practical Applications of Solar Cell Voltage
Understanding solar cell voltage is crucial for harnessing solar energy effectively. Its practical applications extend into various realms, emphasizing the core benefits in both residential and commercial environments. Solar cell voltage not only determines the performance efficiency of solar panels but also influences the design and integration of solar power systems.
Residential Solar Power Systems
In residential settings, solar cell voltage plays a significant role in the effective operation of photovoltaic systems. Homeowners increasingly adopt solar panels for their energy needs, making understanding voltage characteristics essential. The open-circuit voltage (Voc) is a primary metric that affects how much power a solar panel can produce under optimal conditions. Higher Voc values typically correlate with better efficiency and performance.
- Optimal System Design: When designing a residential solar system, understanding the voltage allows for better integration with inverters and battery storage systems. Matching voltage ratings helps ensure that energy losses are minimized and that the system operates at peak efficiency.
- Energy Independence: The ability to harness solar energy increases a home’s independence from the grid. A well-designed, high-voltage solar panel system can supply sufficient power for household needs, reducing dependency on external energy sources and lowering utility bills over time.
- Environmental Benefits: By maximizing solar cell voltage, homeowners contribute to environmental sustainability. Effective usage of solar energy reduces the reliance on fossil fuels, which can diminish carbon footprint.
Commercial Applications
In the commercial sector, solar cell voltage also serves pivotal roles, often linked to large-scale energy savings. Businesses are now adopting solar energy not only for cost reductions but also to promote sustainability. Understanding how solar voltage interacts with business operations can lead to better outcomes.
- Scalability and Cost Efficiency: Companies can scale solar power systems by integrating multiple panels in large arrays. With a solid understanding of voltage characteristics, businesses can evaluate how many solar panels are needed to achieve desired energy outputs without over-sizing, thereby controlling initial costs.
- Enhanced Energy Management: By monitoring solar cell voltage, commercial enterprises can optimize their energy consumption patterns. Incorporating battery storage solutions linked to solar output voltage ensures that energy is available during peak use periods without drawing from the grid.
- Regulatory Compliance and Incentives: Companies switching to solar power can often benefit from tax incentives and subsidies. Understanding the intricacies of solar cell voltage aids organizations in meeting the requirements for these programs, thus enhancing financial viability.
"Solar voltage enables practical approaches to energy management, often leading to direct savings and sustainable practices."
The End and Summary
A primary element discussed is the photovoltaic effect, which is essential for generating electricity from sunlight. Knowing how this effect is influenced by different materials can help in choosing the right type of solar cells for specific applications. Moreover, specific characteristics such as open circuit voltage and short circuit current are vital metrics that provide insight into system performance.
The challenges surrounding solar cell voltage optimization were also tackled. By understanding degradation over time and limitations of materials, we can better address the lifespan and efficiency of solar power systems. These aspects are particularly important for researchers and engineers, who continuously strive for improvements in technology and material science.
Finally, the future directions in solar cell voltage research highlight the importance of ongoing innovation. Emerging technologies can lead to better efficiency and performance metrics, ensuring that solar energy remains a viable option for power generation in various environments.
By synthesizing the information from this article, it is evident that solar cell voltage plays a pivotal role in solar technology. With practical applications ranging from residential systems to larger commercial uses, its optimization will lead toward more sustainable energy solutions.
Recap of Key Points
- Definition: Solar cell voltage is the electrical potential difference generated by solar cells.
- Importance: Voltage is a key factor for determining the efficiency of solar power systems.
- Factors Influencing Voltage: Material properties, temperature effects, and light intensity all affect voltage output.
- Measurement Techniques: Accurate measurement of voltage is crucial for understanding solar cell performance.
- Future Trends: Emerging technologies and innovative materials are set to enhance voltage characteristics in solar cells.
Final Thoughts on Solar Cell Voltage
Solar cell voltage encapsulates a significant aspect of solar technology. It serves not only as a measure of performance but also as a determinant of the viability of solar solutions in tackling energy demands. By comprehensively understanding the nuances surrounding voltage—its generation, optimization, and measurement—stakeholders can contribute to effective solar energy applications.
As we move forward, the focus should remain on innovations in materials and methods that enhance voltage performance. The quest for efficient solar energy solutions continues to necessitate a thorough tackle on the intricacies of solar cell voltage.
"Research in solar energy is about to take a leap forward, and understanding voltage will be key for future advancements."
Engagement with ongoing research and development in this field will keep professionals and enthusiasts informed on the path toward sustainable energy solutions.