Understanding Soil Corrosion: Mechanisms and Solutions

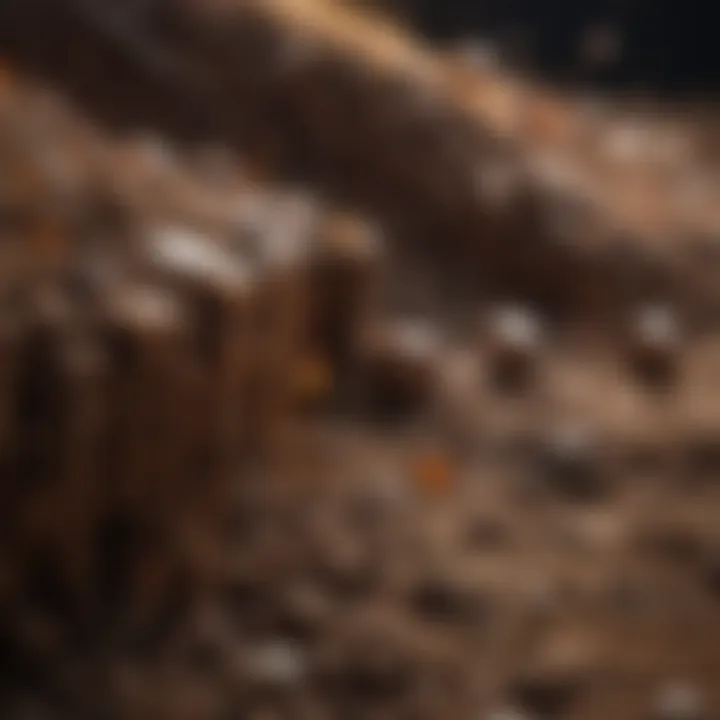
Background and Context
Soil corrosion is an essential subject to understand for those involved in infrastructure, ecology, and agricultural studies. It can impact buildings, roads, and even vegetation. This phenomenon occurs when metals corrode due to chemical reactions in the soil, influenced by moisture, pH, and microbial activity. As layers of soil can encapsulate structures, the severity of corrosion may vary widely. A comprehensive examination of this topic can help in developing strategies to minimize damage and enhance durability of structures.
Historically, the awareness of soil corrosion has grown, especially as urbanization increases and more concrete and steel structures are built. Earlier research focused primarily on the corrosion of metals in isolated environments. However, with urban development and the need for sustainable practices, soil corrosion has gained significant visibility in research and development agendas.
Key Findings and Discussion
Research has unveiled several key findings in the domain of soil corrosion. One significant result is the correlation between soil characteristics and corrosion rates. The presence of chloride ions, for example, often accelerates corrosion. This awareness enables professionals to assess sites for potential corrosion risks.
Major results of the study:
- Soil Moisture: High moisture content often promotes electrochemical reactions leading to corrosion.
- pH Levels: Soils with low pH (acidic) can enhance corrosion rates for certain metals.
- Microbial Influence: Microorganisms in soil can contribute to corrosion by altering the chemical composition around metals.
A deeper analysis reveals that some metals are more susceptible than others. For instance, galvanized steel tends to have better corrosion resistance compared to uncoated steel. This variability underscores the importance of material selection in design and construction phases.
"Mitigating soil corrosion requires both a scientific understanding and practical application to ensure long-lasting infrastructure."
Additionally, understanding the environmental impact is crucial. Soil erosion and degradation of local ecosystems can occur as a secondary effect of unchecked corrosion. It is vital to prioritize both prevention and remediation methods to address these challenges effectively.
In summary, the findings indicate that effective management requires a multidisciplinary approach. Engineers, environmentalists, and policymakers must collaborate to develop comprehensive strategies that not only focus on immediate structural integrity but also on long-term environmental sustainability.
Foreword to Soil Corrosion
Soil corrosion is a critical issue that deserves attention in various fields such as engineering, agriculture, and environmental science. Understanding it is key to protecting infrastructure, ensuring agricultural productivity, and safeguarding ecosystems. This topic explores the intricacies of corrosion processes in soil, which are influenced by various physical and chemical factors.
Addressing soil corrosion is essential. Its implications touch on public safety, financial investments, and environmental sustainability. A deeper knowledge of this subject can lead to informed decision-making and innovative solutions.
Definition of Soil Corrosion
Soil corrosion refers to the degradation of materials, particularly metals, due to electrochemical reactions occurring within the soil environment. These reactions are often a result of the soil's composition, moisture content, and chemical makeup. Specifically, when metals come in contact with corrosive substances in the soil, they can undergo a process leading to their deterioration. This can manifest in several forms, such as rusting or significant thinning of the metal structures.
It is important to note that soil corrosion can occur at various rates, depending on the environmental conditions. For example, oxygen levels, acidic or alkaline conditions in the soil, and the presence of salts can accelerate this degradation.
Relevance of Soil Corrosion in Modern Society
The relevance of soil corrosion in today's society cannot be overstated. Its impact spans several sectors. In urban areas, infrastructure such as pipelines, bridges, and buildings can suffer from corrosion, leading to costly repairs and safety hazards.
In agriculture, soil corrosion can affect farming equipment and irrigation systems, potentially leading to crop losses. Moreover, as society becomes more aware of environmental issues, understanding the ramifcations of soil corrosion on ecosystems becomes crucial.
Furthermore, there is a growing need for materials that resist corrosion and innovative strategies to manage soil conditions. Addressing soil corrosion aligns with broader goals of sustainability and environmental stewardship.
"Soil corrosion is not just a technical problem; it is a matter of public safety, economic investment, and environmental health."
As various industries seek to lessen the impact of soil corrosion, the implications span realms beyond material integrity, highlighting its relevance in modern society.
The Science Behind Soil Corrosion
Soil corrosion is a complex phenomenon influenced by various scientific factors. Understanding these elements is crucial for addressing the effects of soil corrosion on infrastructure and the environment. The study of the science behind soil corrosion not only helps in identifying the mechanisms at play but also guides effective management practices. By examining electrochemical processes, microbial activity, and the different types of corrosion, we can develop better strategies to mitigate the impacts on our surroundings. This section delves into the intricate science behind soil corrosion and the implications it holds.
Electrochemical Processes
Electrochemical processes are foundational to the understanding of soil corrosion. These occur when electrodes, which can be made of metals, are immersed in an electrolyte, like soil containing moisture. When these electrodes are exposed to the positive and negative ions in the soil, a reaction takes place. This reaction can result in the dissolution of metal, leading to deterioration over time.
The rate of corrosion often depends on several variables, such as the conductivity of the soil and the presence of corrosive agents. Soil with high moisture content typically increases electrochemical activity. As a result, metals lose their structural integrity faster.
Microbial Contributions to Corrosion
Microbial activity adds a layer of complexity to soil corrosion. Microorganisms, including bacteria and fungi, can substantially accelerate the corrosion process. Certain bacteria produce acids as metabolic byproducts, which can lead to a more aggressive corrosion environment. The presence of biofilms, which form when microbes settle on a surface, can also result in localized corrosion, presenting challenges for maintaining infrastructure.
The impact of microorganisms raises concerns regarding the durability of materials used in construction and agriculture. Understanding how these biological factors contribute to corrosion can help find better solutions for its management.
Types of Soil Corrosion
Soil corrosion manifests in various forms, each with distinct characteristics and implications.
Uniform Corrosion
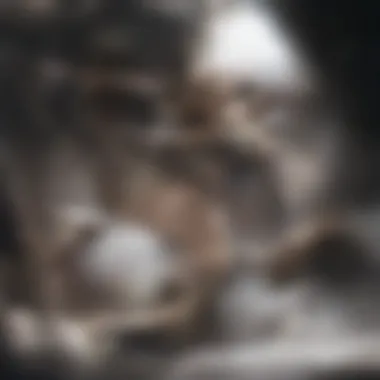
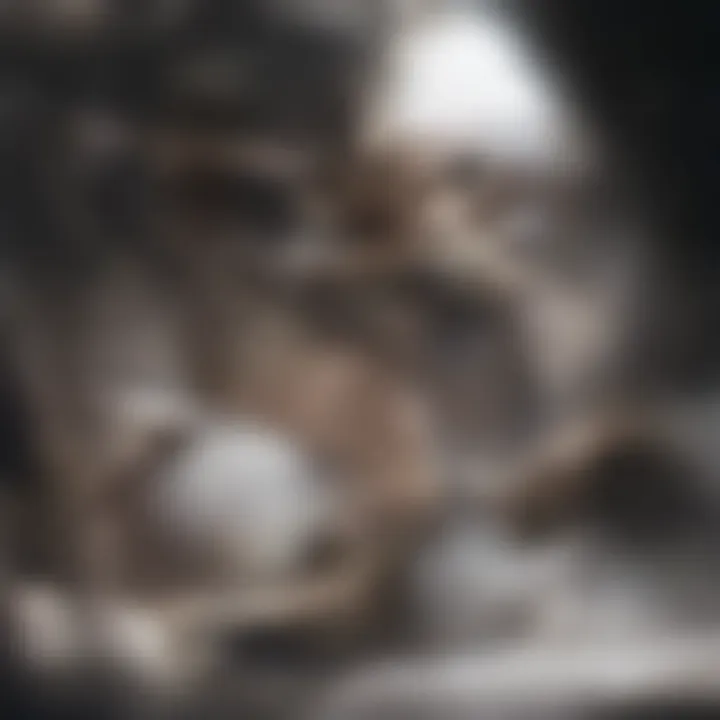
Uniform corrosion is characterized by a consistent and even deterioration of the metal surface. This type occurs across the entire exposed area, making it somewhat predictable. The primary benefit of recognizing uniform corrosion is that it allows for fairly straightforward calculations of metal loss over time. This predictability aids engineers in planning maintenance and replacements.
However, this form of corrosion can still pose challenges. If left unchecked, uniform corrosion can lead to significant structural damage, affecting various engineering applications.
Pitting Corrosion
Pitting corrosion stands distinct due to its localized nature, often appearing as small holes or pits on the metal surface. This form of corrosion can be particularly problematic, as it may not be immediately visible and can lead to sudden failures. Pitting corrosion usually develops in environments with localized chemical imbalances, often influenced by flushing of water or binary metal contact in soil.
The unique challenge posed by pitting corrosion necessitates vigilant monitoring and sometimes, unexpected costs in repair or replacement.
Galvanic Corrosion
Galvanic corrosion occurs when two dissimilar metals are in electrical contact in the presence of an electrolyte, like moist soil. In this situation, the more noble metal gains electrons and is less likely to corrode, while the less noble metal accelerates corrosion. This interaction can lead to rapid deterioration of certain parts of an infrastructure if not managed properly.
Understanding galvanic corrosion is essential for proper material selection in construction projects, ensuring that galvanic couples are minimized or appropriately managed.
In summary, the science behind soil corrosion combines electrochemical reactions, microbial influences, and various types of corrosion. Each element offers valuable insight into soil corrosion's mechanisms, impacting various fields from engineering to environmental management. By grasping these core principles, stakeholders can work towards effective solutions.
Factors Influencing Soil Corrosion
Understanding soil corrosion necessitates a comprehensive examination of its influencing factors. These factors determine the rate and extent of corrosion, which can have significant implications for infrastructure and environmental health. Factors such as soil composition, environmental conditions, and chemical reactions play crucial roles in this process. Analyzing these elements allows for the development of mitigation strategies and informed decisions in both urban planning and agricultural management.
Soil Composition
Soil composition encompasses the minerals, organic matter, water, and air present in soil. This mixture markedly affects its corrosive properties. For instance, sandy soils tend to drain quickly, affecting moisture retention. On the other hand, clay soils can hold water, increasing the potential for corrosion. Moreover, the presence of salts, such as chlorides, can greatly accelerate corrosion rates. Understanding these variations is beneficial for predicting corrosion risks and taking preemptive action in construction and land management.
Environmental Conditions
The environmental conditions surrounding soil greatly influence corrosion processes. Key aspects of these conditions include moisture levels, pH levels, and temperature variations. Each of these factors can either exacerbate or mitigate the rate of corrosion, affecting both infrastructure and plant growth.
Moisture Levels
Moisture levels in soil are critical. When soil is saturated, the water acts as an electrolyte, facilitating electrochemical reactions that lead to corrosion. Higher moisture content is linked to increased corrosion rates. This characteristic of moisture is essential because it underscores the need for effective water management practices. A unique feature of moisture levels is their variability; depending on rainfall and irrigation patterns, moisture can fluctuate considerably, impacting the stability of buried structures and piping systems.
pH Levels
Soil pH levels indicate the acidity or alkalinity of the soil. They significantly influence microbial activity and chemical reactions. Lower pH can enhance the solubility of certain metals, making them more prone to corrosion. Conversely, a higher pH may hinder corrosive processes. Therefore, knowing the pH is an invaluable choice for those seeking to understand soil conditions. A unique feature of pH levels is the fact that they can change over time due to land use practices, necessitating regular monitoring for effective management in both agricultural and urban settings.
Temperature Variations
Temperature variations impact the rate of chemical reactions, including those leading to corrosion. Higher temperatures tend to accelerate these reactions, potentially increasing the degradation of materials exposed to corrosive environments. Temperature changes can also affect moisture levels, which is intertwined with the overall corrosion process. The distinctive feature of temperature variations is their seasonality; for example, extreme cold can change soil conditions significantly, resulting in freeze-thaw cycles that further contribute to the corrosion of materials. Understanding temperature's impact is beneficial for long-term infrastructure planning and agricultural practices.
Chemical Reactions in Soil
Chemical reactions in soil involve interactions between soil minerals, moisture, and air. These reactions can lead to the formation of corrosive agents that contribute to the degradation of materials. Common processes include oxidation, reduction, and hydrolysis, all of which can lead to various forms of soil corrosion. Exploring these reactions is necessary, as they can inform the choices of materials used in construction and agriculture.
"Factors such as soil composition and environmental conditions directly dictate the behavior and rate of soil corrosion. Ignoring these elements can lead to costly consequences."
Analyzing chemical reactions alongside soil composition and environmental conditions provides a holistic understanding of soil corrosion. This multifaceted approach is essential for effective assessments and tailored mitigation strategies.
Effects of Soil Corrosion
Soil corrosion is an issue that penetrates deep into various societal functions. Understanding its effects is crucial for students, researchers, educators, and professionals alike. The term encapsulates the degradation of materials when they are in contact with moist soil, which can lead to severe consequences. These consequences are not just confined to physical structures but extend to plant health and the broader environment.
Impact on Infrastructure
Infrastructure is undeniably at risk from soil corrosion. The materials, mainly metals, experience deterioration due to corrosive soil environments. Structures such as pipelines, bridges, and underground tanks are prone to corrosion. The costs associated with repairs and replacements are significant.
- Cost Implications: It is estimated that billions of dollars are lost each year due to corrosion-related failures in infrastructure. Regular maintenance is often insufficient to mitigate the damage.
- Safety Risks: Infrastructure failures can jeopardize public safety, leading to accidents that may cause injuries or fatalities.
- Long-term Solutions: Engineers and planners need to be proactive. Implementing anti-corrosion systems, monitoring technology, and regular inspections can help minimize the risks.
With the increasing strain on existing infrastructure, addressing soil corrosion becomes even more relevant.
Influence on Plant Health
Soil corrosion also has a detrimental effect on plant health. When corrosion occurs, it often leads to the release of toxic elements into the soil. As a result, the ecosystem surrounding the contaminated areas can be severely impacted. This phenomenon has several considerations:
- Nutrient Imbalance: Corrosive substances can disrupt the nutrient composition of soil. Essential elements for plant growth, such as nitrogen and phosphorus, may be diminished.
- Toxicity Issues: Heavy metals and other harmful substances released during corrosion can create hostile environments for plants. Ingesting these toxins can lead to stunted growth and decreased productivity.
- Agricultural Challenges: Farmers are particularly affected. Reduced plant health can lead to lower crop yields and consequently impact food supply.
In studying soil corrosion's impact on agriculture, it's important to search for sustainable alternatives.
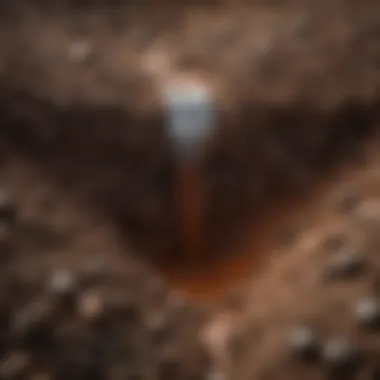
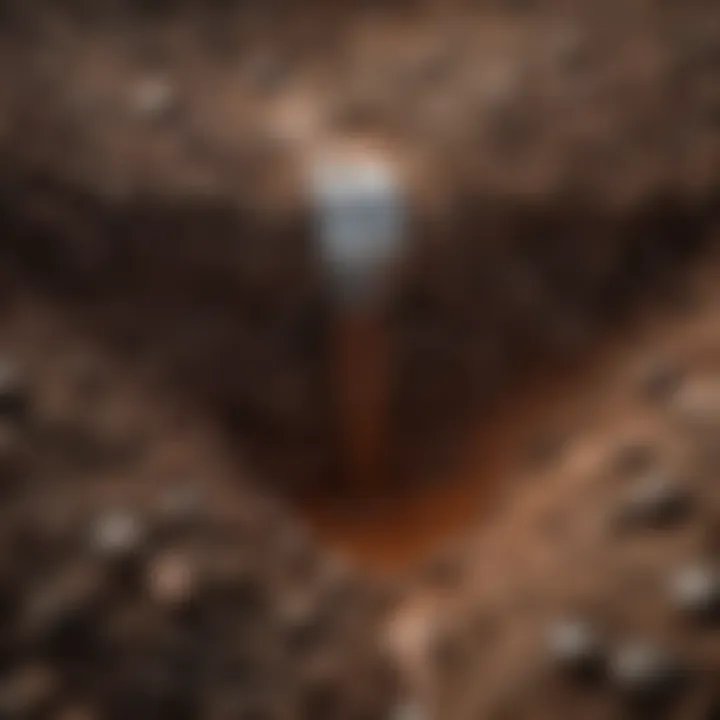
Environmental Ramifications
The environmental ramifications of soil corrosion extend far beyond local immediate impacts. When studying this issue, the interconnections within ecosystems become clear.
- Water Contamination: Corrosion can lead to metal leaching into groundwater or surface water bodies. This contamination poses risks to local wildlife and drinking water supplies.
- Biodiversity Loss: Soil quality is essential for sustaining diverse biological communities. With soil degradation, flora and fauna struggle to survive, potentially leading to a profound loss of biodiversity.
- Climate Change Interactions: There are implications regarding soil corrosion in relation to climate change. Certain conditions, such as increased moisture, may exacerbate corrosion effects, creating a vicious cycle of degradation.
"The understanding of soil corrosion effects holds the key to developing strategies to protect our environment and infrastructure."
Overall, the effects of soil corrosion are multifaceted and far-reaching. The implications for infrastructure, plant health, and the environment call for immediate attention and sustained research efforts. Proper assessments and strategies are vital. Only by recognizing the broad impacts can we begin to formulate effective mitigations.
Assessment and Monitoring of Soil Corrosion
The assessment and monitoring of soil corrosion is essential for effective management of various infrastructures and agricultural practices. Identifying corrosion early enables timely intervention, reducing the risk of structural failures and protecting both plants and the environment. The complexity of soil environments means that without adequate monitoring, corrosion processes can remain hidden until significant damage has occurred. This makes systematic evaluation critical, especially in urban and agricultural settings.
Proper evaluation can provide valuable data on how soil conditions evolve over time. Understanding these dynamics can help in developing strategies to mitigate adverse effects. Improved monitoring practices ensure informed decision-making, targeted remediation efforts, and can optimize maintenance schedules for infrastructure systems. Additionally, this proactive approach often results in cost savings over time by preventing catastrophic failures and maintaining optimal growth conditions for plants.
"Regular monitoring not only safeguards against immediate risks but also contributes to long-term sustainability efforts."
Standard Testing Methods
Standard testing methods are crucial in the assessment of soil corrosion. They allow for the quantification of various parameters that influence corrosion processes. Common tests include:
- Soil Resistivity Testing: Measures the electrical resistivity of soil, which indicates how easily electrical currents can flow through it. Low resistivity values often correlate with higher corrosion rates.
- pH Testing: Understanding the acidity or alkalinity of the soil is fundamental since it affects the solubility of metal ions. Lower pH values can increase corrosion risks.
- Redox Potential Measurement: This assesses the oxidizing or reducing conditions in the soil. High reducing potentials typically enhance microbial activity that can contribute to corrosion.
- Moisture Content Assessment: Evaluating how much water is present in the soil is vital. Waterlogged conditions can facilitate corrosion through electrochemical processes.
Each of these methods provides insight into the factors contributing to soil corrosion, enabling better management practices.
Field Monitoring Techniques
Field monitoring techniques are implemented to continuously assess soil conditions and corrosion rates in real time. This ongoing observation is critical for managing risks effectively. Techniques include:
- In-Situ Sensors: These sensors can be deployed directly in the soil to measure parameters like moisture, pH, and electrical conductivity. The data collected allows for quick adjustments in management practices.
- Corrosion Rate Measurement Devices: By incorporating devices that measure corrosion rates in metallic structures within the soil, one can closely track the effectiveness of protective measures.
- Soil Sampling: Periodic soil sampling, followed by analysis in laboratories, helps validate field data and reveals changes in soil chemistry over time. This approach provides a clearer picture of underlying processes.
- Surveying Techniques: Using geophysical methods, such as ground penetrating radar, one can identify potential corrosion-affected zones without intrusive sampling.
Mitigation Strategies for Soil Corrosion
Soil corrosion represents a significant challenge affecting various sectors, including construction and agriculture. Effective mitigation strategies can tremendously reduce corrosion effects. This subheading emphasizes the importance of selecting the right approaches to address soil corrosion while considering factors such as cost, effectiveness, and practicality. A multi-faceted approach is often beneficial in dealing with soil corrosion, blending several strategies for optimum results.
Soil Amendments
Soil amendments play a crucial role in mitigating soil corrosion. These amendments can enhance the chemical and physical properties of the soil, making it less conducive to corrosion. For instance, adding lime can help to raise the pH of acidic soils, promoting a more neutral environment that is less corrosive.
Common soil amendments include:
- Lime
- Gypsum
- Organic matter
- Biochar
Applying these materials can change the soil composition, improving its ability to support infrastructure. As a result, soil amendments not only assist in corrosion prevention but can also enhance plant growth and overall soil health.
Using Corrosion-Resistant Materials
Incorporating corrosion-resistant materials is vital in protecting structures from the harmful effects of soil corrosion. Materials such as stainless steel, fiberglass, and specially coated metals resist corrosion more effectively than standard options. These materials can withstand the harsh conditions associated with soil environments, thereby prolonging the lifespan of infrastructure.
When selecting materials, it is essential to consider factors such as:
- Cost
- Availability
- Engineering requirements
Strategies that involve the careful choice of corrosion-resistant materials can yield long-term benefits, preventing extensive damage and reducing maintenance costs over time.
Water Management Practices
Water management practices are another essential aspect of soil corrosion mitigation. Properly managing water can significantly lower the risk of corrosion. Excess moisture increases the conductivity of soil, which accelerates electrochemical reactions that lead to corrosion.
Some effective water management practices include:
- Install drainage systems to control water flow.
- Use mulch to conserve soil moisture and reduce erosion.
- Conduct regular inspections to identify and remedy drainage issues.
By implementing effective water management strategies, it is possible to create a less favorable environment for corrosion to occur. Integrating these practices with other mitigation strategies provides a comprehensive approach to managing soil corrosion.
In summary, addressing soil corrosion requires a mix of techniques. The use of soil amendments, corrosion-resistant materials, and effective water management are key strategies in mitigating the effects of this pervasive problem.
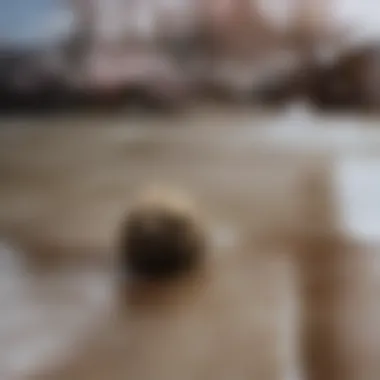
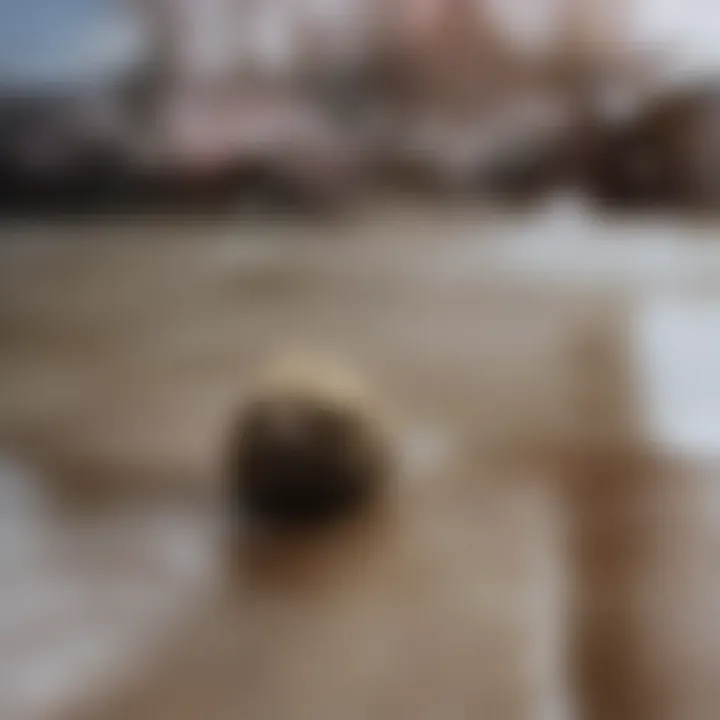
Case Studies of Soil Corrosion
Understanding the real-world consequences of soil corrosion requires examining specific case studies. This section emphasizes how theoretical concepts apply to practical situations. Learning from such examples can offer valuable insights into the mechanisms at play, the resulting impacts, and potential mitigation efforts. Through these case studies, we can appreciate the complexity of soil corrosion and why it matters in various contexts.
Urban Infrastructure Corrosion
Urban settings often feature extensive underground infrastructure that can be severely affected by soil corrosion. This is particularly evident in cities where water and sewage systems are buried in soil that may contain high levels of chlorides or sulfates. When moisture levels rise, these chemicals can initiate and accelerate electrochemical processes leading to corrosion.
A notable case is the water supply system in Chicago. Over the years, the aging pipes made of cast iron have experienced substantial degradation. This corrosion not only compromises the integrity of the infrastructure but also poses health risks. Corroded pipes may lead to metal leaching into the drinking water. Monitoring such infrastructures is crucial for public health and safety.
Factors that contribute to urban infrastructure corrosion include:
- Electrical resistivity variations in soil
- Nearby construction activities that alter drainage patterns
- Pollutants affecting the soil chemistry
It's essential to study these elements to effectively identify solutions. Implementing corrosion-resistant materials or protective coatings can significantly minimize the impact of corrosion in these urban environments.
Agricultural Soil Degradation
Agricultural practices can also fall victim to soil corrosion, impacting crop yields and soil quality. When ferrous and ferric ions from corroded pipes leach into the soil, the nutrient composition changes and can affect plant health. One significant example is seen in areas where fertilizers high in nitrates are used extensively. This practice may alter pH levels, which, combined with high moisture, can accelerate corrosion.
Farmers must deal with a double-edged sword here. While they aim to enhance productivity, they may inadvertently create conditions conducive to soil corrosion.
Key factors influencing agricultural soil degradation include:
- Soil texture and structure
- Organic matter content
- Irrigation methods that may exacerbate moisture retention
To counteract these effects, farmers are encouraged to adopt sustainable practices. These include the use of less corrosive fertilizers, crop rotation, and the inclusion of organic amendments to enhance soil health.
Case studies illustrate that understanding the dynamics of soil corrosion is crucial for informed decision-making in both urban infrastructure management and agricultural practices.
Future Research Directions in Soil Corrosion
Soil corrosion presents a complex set of challenges that affect many systems, from urban infrastructure to agriculture. Understanding the future directions in research is essential for developing effective solutions to mitigate these issues. This section delves into the emerging trends and areas in soil corrosion studies that hold promise for more sustainable management and prevention techniques.
Research in this field is critical not only for improving existing practices but also for tackling new problems arising from urbanization and climate change. By investigating innovative technologies for detection and advancements in remediation techniques, future research can offer significant benefits.
Some considerations influencing future research are:
- Technical Advancements: Incorporating new materials and detection methods.
- Environmental Impact: Analyzing how changes in soil chemistry and structure affect corrosion rates.
- Policy Frameworks: Understanding regulatory needs can guide effective research.
"Addressing soil corrosion effectively requires a blend of scientific inquiry and practical application in engineering solutions."
Innovative Technologies for Detection
The role of technology in soil corrosion detection is increasingly important. The development of innovative detection technologies can revolutionize how engineers and researchers monitor corrosion processes. Current methods may involve soil sampling and laboratory analysis, which can be time-consuming and costly. Newer approaches include:
- Non-invasive Sensors: These devices can monitor soil conditions continuously, providing real-time data on moisture, pH, and resistivity.
- Remote Sensing: Satellite imagery and unmanned aerial vehicles (UAVs) can assess large areas of infrastructure for early signs of corrosion.
- Smart Systems: Integrating Internet of Things (IoT) devices can offer a comprehensive network for continuous monitoring and data analysis.
The use of these innovative technologies can significantly enhance the efficiency of maintenance strategies and lead to early identification of at-risk areas.
Advancements in Remediation Techniques
As the understanding of soil corrosion evolves, so do the remediation techniques available. Future research is likely to focus on more sustainable and effective methods for mitigating corrosion damage. Key advancements may include:
- Bioremediation: Leverage microbial processes to restore soil health and limit corrosion.
- Electrochemical techniques: Using electric currents to inhibit corrosion can offer precise control over the mitigation process.
- Green Chemistry Approaches: Developing eco-friendly chemicals for soil treatment can minimize environmental impact.
Focusing on these advancements aligns with the broader context of sustainable development and environmental conservation. A thorough understanding of these techniques can lead to improved soil management plans that reduce corrosion while maintaining soil integrity.
The ongoing research efforts in these areas will ideally create more resilient infrastructures, reduce costs, and protect ecological health.
Closure
Soil corrosion poses significant challenges across various sectors, impacting infrastructure, ecosystems, and agricultural productivity. Its complexities demand thoughtful analysis and methods of mitigation. Understanding the mechanisms behind soil corrosion is essential for developing effective solutions. The findings from this article highlight crucial considerations for researchers and practitioners alike.
Summary of Key Findings
- Mechanisms of Soil Corrosion: Electrochemical reactions play a fundamental role in the corrosion of metal structures embedded in soil. Such interactions often cause uniform, pitting, or galvanic corrosion, which can lead to severe structural failures if unaddressed.
- Influencing Factors: The type of soil, moisture levels, pH, and temperature greatly influence the rate of corrosion. These elements must be monitored to protect infrastructures effectively.
- Mitigation Strategies: Various strategies can be implemented to combat soil corrosion. These include using corrosion-resistant materials, soil amendments, and effective water management techniques.
- Future Research Directions: Ongoing research is needed to explore innovative technologies for detecting corrosion and enhancing remediation techniques, ensuring infrastructures can withstand adverse soil conditions.
Implications for Future Practice
The insights gained from this study about soil corrosion inform practices across engineering, agriculture, and environmental management sectors. As urban areas expand and climate challenges become pressing, addressing soil corrosion through informed decisions is paramount.
- Engineers should consider soil properties when designing infrastructure.
- Farmers can implement soil management practices that promote plant health while minimizing corrosion risks.
- Environmental policies can be developed to account for the effects of soil corrosion on ecosystems.
Engaging with these implications ensures that professionals remain proactive in addressing soil corrosion, fostering resilience in infrastructure and cultivating sustainable practices.