Understanding SmFISH Probes in Molecular Biology
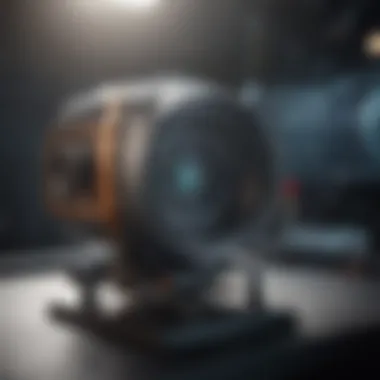
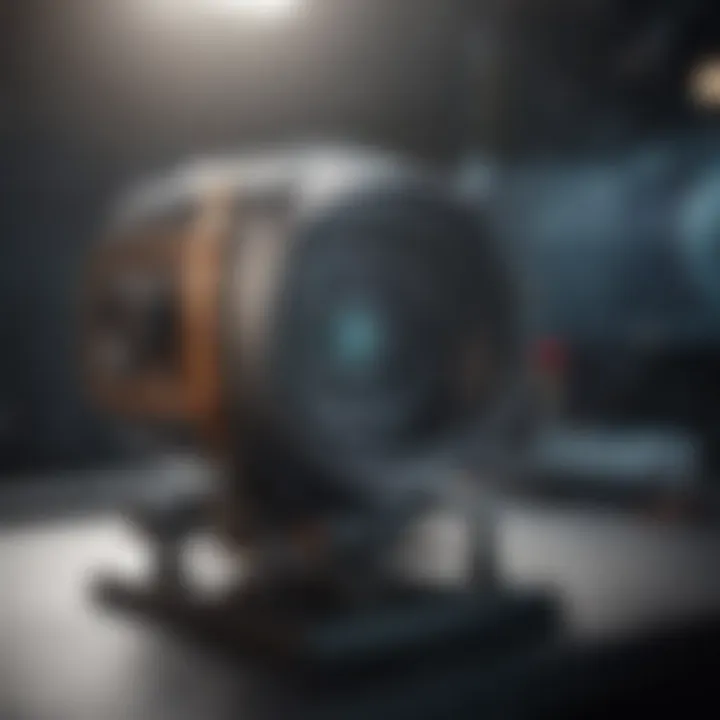
Intro
Single-molecule fluorescence in situ hybridization (smFISH) represents a pivotal advancement in molecular biology. It allows for the visualization and quantification of specific RNA molecules within cellular contexts. This methodology harnesses highly specialized probes that bind uniquely to target RNA sequences, leading to enhanced understanding of gene expression patterns. As biological systems become more complex, the development and application of smFISH probes provide a critical tool for researchers striving to uncover intricate cellular processes.
Background and Context
Overview of the Research Topic
The study of gene expression has long stood as a cornerstone of molecular biology. Traditionally, techniques like RT-PCR and Northern blotting provided valuable insights into RNA levels but often fell short in spatial resolution and single-molecule detection.
Contrastingly, smFISH takes a different approach. By employing fluorescently labeled oligonucleotide probes, this technique enables researchers to visualize individual RNA transcripts within their native cellular environments. This high level of specificity and sensitivity addresses limitations that have been present in earlier methodologies.
Historical Significance
The evolution of smFISH probes can be traced back to early fluorescence microscopy techniques. With advancements in molecular biology and imaging technology, the concept of visualizing single molecules became a reality. Key figures, such as Raj and Tyagi, significantly contributed to the theoretical framework and practical application of this innovation in the 2000s.
Since then, numerous studies have employed smFISH to elucidate developmental processes, disease mechanisms, and cellular responses to environmental stresses. The incorporation of smFISH into standard laboratory practices has reshaped the way gene expression studies are designed and executed, facilitating a more detailed understanding of cellular dynamics.
Key Findings and Discussion
Major Results of the Study
Recent research highlights several major advancements in smFISH probe design and application. For instance, the integration of branched DNA amplification strategies has markedly increased the sensitivity of traditional smFISH. This approach enables the detection of very low-abundance RNA molecules, which is crucial for studies involving rare cell types or transient gene expression.
Moreover, multiplexing capabilities have expanded, allowing researchers to visualize multiple RNA species simultaneously. This ability to perform concurrent assessments of different gene expressions facilitates comprehensive analysis of cellular behaviors in terms of pathways and networks.
Detailed Analysis of Findings
The analysis of smFISH applications reveals several advantages over conventional methods. Here are key points of the nuanced findings:
- Spatial Resolution: Unlike bulk RNA analysis techniques, smFISH provides insights with subcellular resolution.
- Quantitative Insights: The method allows for relative quantification of RNA molecules within cells, guiding conclusions on gene expression levels.
- Real-time Monitoring: With the use of live-cell fluorescence imaging, changes in RNA dynamics can be tracked over time, adding a temporal dimension to gene expression studies.
In the context of rising complexity in biological research, smFISH and its probes are becoming increasingly essential. Their unique capabilities offer profound implications for our understanding of gene regulation and expression in various biological contexts. Moreover, ongoing research is likely to uncover further innovations in probe design, enhancing their utility across numerous applications in molecular biology.
Preamble to SmFish Probes
The exploration of smFISH probes marks a significant advancement in molecular biology. Their precision in detecting single RNA molecules has transformed scientific research, allowing for unprecedented insights into gene expression and cellular processes. The ability to visualize RNA localization and quantify gene activity in situ enables researchers to understand complex biological systems at a granular level.
Definition and Overview
SmFISH, or single-molecule fluorescence in situ hybridization, refers to a technique that uses fluorescent probes to detect and localize specific RNA molecules within cells. The technique relies on the binding of multiple labeled probes to a target RNA sequence, yielding a fluorescent signal that can be visualized under a fluorescence microscope. This method offers high spatial resolution, making it ideal for studying the dynamics of gene expression.
Several key elements define smFISH probes:
- Target Specificity: Probes are designed to bind selectively to a particular RNA sequence, thus minimizing background noise and enhancing detection accuracy.
- Signal Amplification: The use of multiple probes binding to a single RNA molecule amplifies the fluorescent signal, allowing for the detection of low-abundance transcripts.
- Quantifiable Results: SmFISH permits not only qualitative analysis but also quantification of RNA levels, providing more robust data for researchers.
In essence, smFISH serves as a bridge between molecular biology and cellular imaging, allowing researchers to explore biological phenomena with greater depth and clarity.
Historical Context
The development of smFISH probes can be traced back to the evolution of fluorescence-based technologies and their application in molecular biology. Initial advances in in situ hybridization provided the groundwork for more refined techniques. Traditional methods were limited in terms of resolution and sensitivity, compelling scientists to seek improvements.
In 2008, the introduction of smFISH by Femke van Rooij et al. represented a pivotal shift. They demonstrated enhanced capabilities in detecting specific RNA molecules within their cellular context. This technique gained traction due to its robustness and the ability to analyze single molecules instead of bulk samples.
Today, smFISH is widely established in laboratories worldwide and continues to evolve. The integration of new fluorescent dyes and improved imaging technologies furthers its potential in research, making it indispensable for contemporary studies in transcriptomics and cellular dynamics. The historical trajectory underscores the interplay between technological innovation and scientific inquiry, highlighting how advances in one field can lead to transformative changes in another.
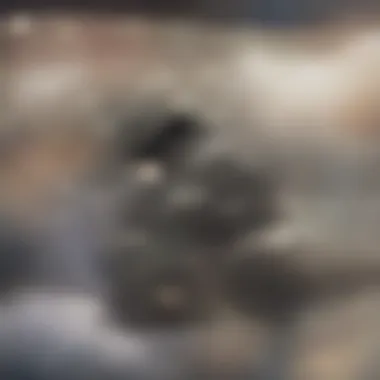
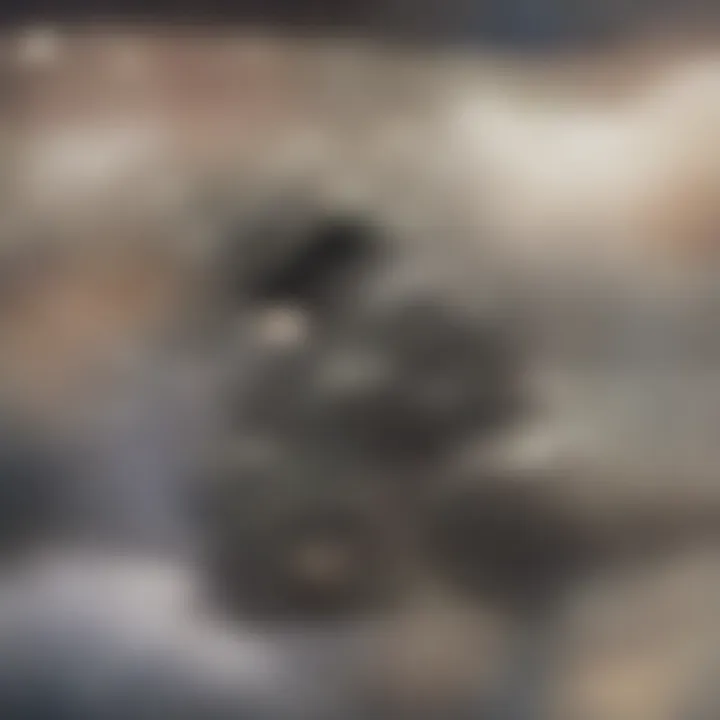
The Science Behind SmFISH Probes
The science underlying single-molecule fluorescence in situ hybridization (smFISH) probes is instrumental in advancing molecular biology. These probes offer unprecedented precision in detecting RNA molecules at the single-cell level. By focusing on the mechanisms of detection and the role of fluorescence, we can uncover how smFISH fulfills its potential as a powerful tool in various research domains. Understanding these aspects is crucial for researchers aiming to leverage smFISH in their studies.
Single-Molecule Detection Mechanism
At the core of smFISH technology is its ability to detect individual RNA molecules. This detection mechanism is founded on the principles of hybridization and fluorescence. Probes are designed to bind specifically to target RNA, ensuring high specificity. Once bound, these probes emit fluorescence upon excitation, allowing researchers to visualize and count the RNA molecules.
The key steps in this mechanism include:
- Probe Design: Custom probes are created to complement specific RNA sequences. The design process needs to consider factors like sequence specificity and the length of the probe.
- Hybridization: Probes hybridize to their respective targets within fixed cells or tissues. This step requires a controlled environment, as temperature and buffer composition can influence the binding efficiency.
- Detection: After hybridization, excess probes are washed away, and the remaining bound probes are imaged using a fluorescence microscope. This imaging enables the reliable detection of single RNA molecules.
A compelling aspect of this detection mechanism is its high sensitivity. Even in samples with low RNA abundance, smFISH can still deliver reliable data, making it a preferred choice in studies where understanding gene expression dynamics is critical.
"The invention of smFISH has revolutionized our understanding of RNA localization and expression patterns in cells."
Fluorescence and Its Importance
Fluorescence is fundamental to the smFISH method, as it provides the means to visualize and analyze the bound probes quantitatively. The choice of fluorophores is crucial because different fluorophores have unique excitation and emission spectra, determining how they can be used in combination.
Some significant points related to fluorescence in smFISH include:
- Fluorophore Selection: The right fluorophores must be chosen based on their brightness and stability. Commonly used fluorophores include Cy3 and Cy5, which have distinct emission wavelengths.
- Multiple Fluorescence Channels: Multiplexing is a feature in smFISH that allows researchers to use different colored probes simultaneously. This enables the examination of multiple RNA targets in a single experiment, providing rich insights into cellular processes.
- Photostability: Photobleaching, which occurs when fluorophores lose their ability to fluoresce after prolonged exposure to light, is a concern. Techniques that minimize photobleaching enhance the reliability of the results, allowing for extended observation times.
Ultimately, the importance of fluorescence in smFISH can be summed up as follows: it turns the abstract concept of RNA detection into a tangible, observable phenomenon, thereby facilitating a detailed understanding of molecular biology.
Designing SmFISH Probes
Designing SmFISH probes requires careful planning and consideratoin. The design phase is critical since it directly affects the probe's ability to bind specifically and efficiently to the target RNA. Effective designs will improve both sensitivity and specificity in detecting single-molecule RNA signals, which is essential for accurate gene expression analysis and cellular localization studies.
Choosing the Right Target Sequence
The foundation of any successful SmFISH experiment lies in selecting the right target sequence. Choosing an appropriate sequence demands an understanding of the RNA's structure and its context within the cellular environment. Factors influencing this decision include:
- Uniqueness: The selected sequence should be unique to the gene of interest to eliminate cross-reactivity with other RNA molecules.
- Length: Optimal lengths typically range between 20-30 nucleotides, which balances specificity and binding efficiency.
- Secondary Structures: Consideration of the RNA's secondary structure is crucial. A probe must bind effectively, avoiding regions that are less accessible due to complex folding.
Making the correct choice of a target sequence can greatly enhance the success rate of an experiment.
Probe Labeling and Construction
Once the target sequence is decided, the next step involves labeling and constructing the probe. There are several key factors to consider in this phase:
- Fluorophores: The choice of fluorophore affects the sensitivity and background noise of the detection method. Commonly used fluorophores include Cy3 and Cy5, which provide distinct wavelengths for signal differentiation.
- Chemical Modifications: Incorporating modified nucleotides can improve properties such as stability, binding affinity, and resistance to nucleases.
- Linkers: Appropriate linkers can enhance the flexibility and positioning of the fluorophore relative to the RNA target, which is essential for maximizing signal output.
The construction of a well-designed probe can significantly impact imaging outcomes.
Optimizing Probe Performance
After probe design and labeling, optimizing performance is vital. Proper optimization ensures reliable and reproducible results. Here are strategies to enhance performance:
- Concentration Tuning: It is important to test different concentrations of the probe to find the optimal balance between signal intensity and background noise.
- Annealing Conditions: Varying temperature during hybridization can affect the specificity of binding. A better match between the hybridization temperature and the probe's melting temperature leads to more effective signal detection.
- Imaging Parameters: Adjusting imaging settings, such as exposure time and gain, can improve signal clarity and minimize artifacts.
By attending to these considerations, researchers can significantly improve the quality of their fluorescence signals, leading to more accurate observations and data interpretation.
Effective designing of SmFISH probes is key to unlocking insights into complex biological systems.
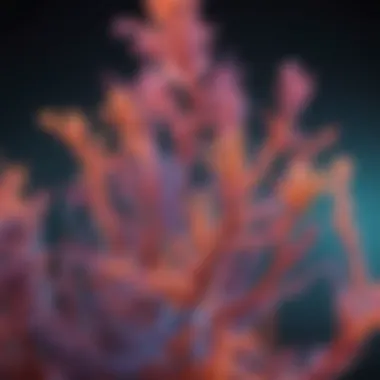
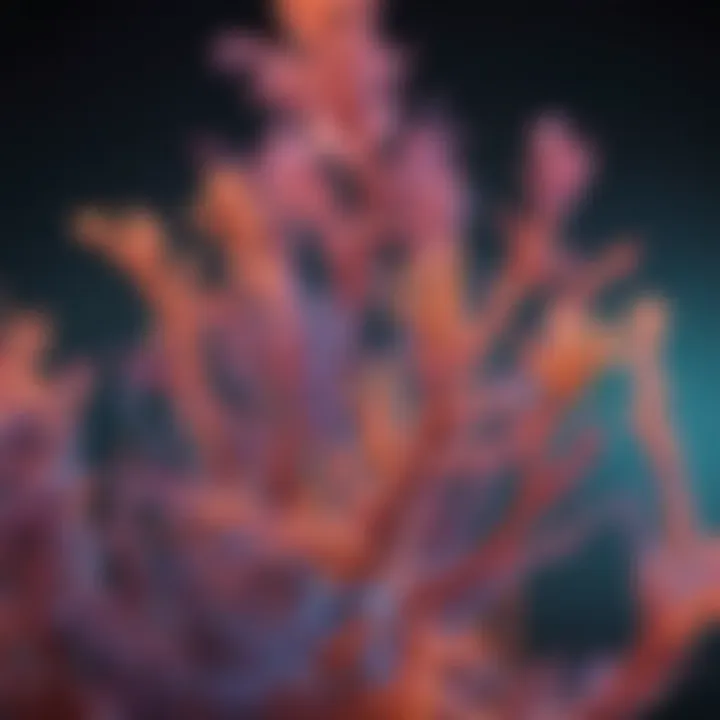
Applications of SmFISH Probes
Single-molecule fluorescence in situ hybridization (smFISH) probes serve an integral role in molecular biology. Their applications stretch across various areas, providing insights into the behavior of RNA within cells and tissues. Understanding these applications is critical for exploiting the full potential of smFISH technology in research. Each application highlights unique benefits, allowing researchers to comprehend complex biological phenomena at an unprecedented resolution.
Gene Expression Analysis
smFISH probes are essential tools in gene expression analysis. By using these probes, scientists can measure the levels of specific mRNA molecules in cells. This quantitative analysis is possible because smFISH enables the detection of single RNA molecules, providing a precise count that traditional techniques cannot achieve.
Some important aspects include:
- Higher Resolution: smFISH reveals expression patterns at the cellular level, enabling researchers to identify heterogeneity within populations of cells.
- Spatial Context: It allows for the localization of mRNA in situ, showing where within the cell gene expression occurs.
- Dynamic Studies: Researchers can utilize time-lapse imaging to observe changes in expression over time, interpreting signals in a dynamic context.
Gene expression analysis through smFISH has transformed our understanding of gene regulation and has significant implications for diseases, such as cancer, where aberrant expression patterns can be diagnostic.
Studying RNA Localization
Understanding RNA localization is crucial for deciphering the functional roles of specific RNA molecules. smFISH probes enable researchers to visualize the spatial distribution of RNA within cells, providing insight into how the localization contributes to RNA function. The technique helps illustrate how mRNA molecules are directed to specific cell regions, impacting processes such as protein synthesis.
Key benefits of studying RNA localization with smFISH include:
- Identification of Localization Patterns: By employing specific smFISH probes, researchers can discern when and where certain mRNAs are accumulated in the cell.
- Functional Insights: Localized RNA is often crucial for cellular functions. smFISH helps correlate localization with protein production and cellular behavior.
- Disease Relevance: Abnormal RNA localization may signal disease states. This methodology can reveal potential markers for early diagnosis in diseases.
Investigating Cellular Dynamics
The study of cellular dynamics involves understanding how cells respond to various stimuli over time. smFISH probes facilitate this investigation by providing a powerful means to monitor RNA responses in real time. Researchers can conduct live-cell imaging experiments to observe transcriptions and other dynamic processes relevant to cellular function.
- Transient Events Tracking: With smFISH, researchers can track the production and degradation of RNA in real time, letting them study transient changes in gene expression patterns.
- Combined Analysis: The method can be paired with other imaging techniques, such as fluorescence recovery after photobleaching (FRAP), to add layers of data about RNA mobility and interactions.
- Understanding Response Mechanisms: Cellular response to external signals is often reflected in RNA synthesis and degradation. By analyzing these dynamics, one can gain crucial insights into cellular behavior under various conditions.
smFISH probes are integral for a comprehensive exploration of gene expression, RNA localization, and cellular dynamics. In acknowledging the importance of these applications, one can fully appreciate the transformative impact smFISH technology has on molecular biology research.
Comparative Techniques to SmFISH
In the landscape of molecular biology, understanding the various methodologies for studying RNA is essential. Comparative techniques to single-molecule fluorescence in situ hybridization (smFISH) offer unique insights, broaden analytical capabilities, and allow for a more nuanced interpretation of data. Such techniques include in situ hybridization and RNA sequencing, each with its distinct advantages and limitations.
In Situ Hybridization Techniques
In situ hybridization (ISH) refers to the methodology that allows for the localization of specific nucleic acid targets within fixed tissues and cells. Unlike smFISH, which detects single molecules, ISH can visualize larger quantities of RNA. This characteristic makes it suitable for observing spatial gene expression patterns across different tissues.
Several important aspects of ISH are worth noting:
- Sensitivity and Specificity: ISH techniques can reach high specificity due to the design of probes that hybridize to complementary RNA sequences. However, sensitivity may vary based on the quality and nature of the probes used.
- Visual Representation: ISH allows for the incorporation of a morphological context within the study, providing images of cell types and tissue structures alongside the expression data. This context is crucial for understanding the biological role of the genes being studied.
Despite these advantages, ISH may not be able to achieve the same level of resolution as smFISH. Additionally, the interpretation of results can be complicated by background noise and signal variability.
"While ISH provides valuable information regarding gene localization, it may not match the sensitivity and resolution of smFISH."
RNA Sequencing
RNA sequencing (RNA-seq) is an advanced method that allows for comprehensive analysis of the transcriptome. This technique facilitates an understanding of global gene expression profiles, enabling researchers to quantify RNA levels from a large number of genes simultaneously.
Key elements related to RNA sequencing include:
- Quantitative Analysis: RNA-seq offers a quantitative measure of gene expression across different conditions. This capacity allows for deep insights into how genes interact and the dynamics of cellular responses.
- No Pre-conceived Bias: Unlike other techniques, RNA-seq does not necessitate prior knowledge of transcript sequences, allowing for the discovery of novel transcripts and splice variants. This characteristic underscores its applicability in exploratory studies.
However, RNA-seq cannot provide spatial localization information of RNA within the tissues, which is where techniques like smFISH and ISH excel. Therefore, the choice of technique often depends on whether the focus is on spatial context or large-scale expression analysis.
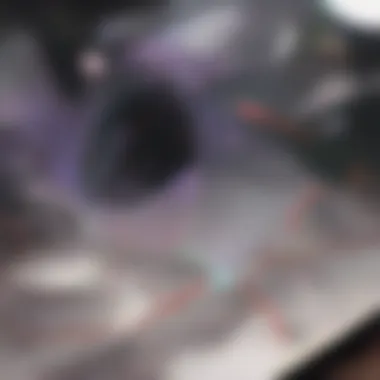
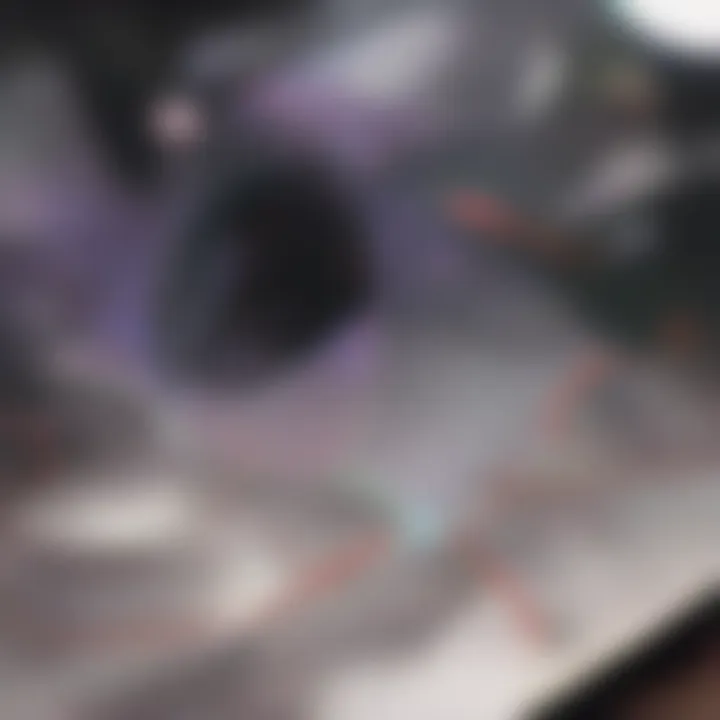
In summary, comparative techniques such as in situ hybridization and RNA sequencing are pivotal in broadening the understanding of RNA biology. Each methodology presents its own strengths and weaknesses, shaping experimental design decisions based on scientific objectives.
Challenges in SmFISH Probe Studies
SmFISH probe studies present unique challenges that can impact the quality and reliability of experimental results. Understanding these challenges is crucial for researchers aiming to achieve accurate and reproducible findings in their studies. Common issues such as signal variability, noise, tissue penetration, and accessibility play significant roles in the effectiveness of smFISH techniques.
Signal Variability and Noise
Signal variability is a major concern when using SmFISH probes. This variability can arise from multiple factors such as probe design, hybridization efficiency, and the inherent properties of the fluorophores used. For instance, when probes do not bind efficiently to their target sequences, inconsistent signals can be detected, leading to erroneous interpretations of gene expression levels. Additionally, background noise can obscure relevant signals, further complicating the analysis.
Researchers must carefully optimize hybridization conditions to minimize these negatives effects. Factors such as temperature, salt concentration, and incubation times can all influence the specificity and sensitivity of the signals detected. It is also important to select high-quality probes with low non-specific binding to enhance the clarity of the signals. Maintaining consistent experimental procedures can greatly aid in reducing variability and improving data quality.
"Minimizing signal variability is key to achieving reliable results in SmFISH studies."
Tissue Penetration and Accessibility
Another challenge is related to tissue penetration and accessibility of SmFISH probes. The ability of these probes to reach their desired targets within complex cellular architectures can be limited. Tissues, especially dense ones or those with high lipid content, can act as barriers to probe infiltration, resulting in incomplete or uneven labeling.
In order to address this, researchers may need to employ different strategies such as:
- Optimizing Fixation Protocols: The way tissues are fixed can influence the permeability of the probes. Fixation methods must allow for adequate penetration while preserving the cellular structure.
- Using Enhanced Delivery Techniques: Techniques like microinjection or electroporation may be explored to increase probe accessibility in specific cells or tissues.
- Exploring Alternative Formulations: Modifying the chemical properties of probes, such as increasing hydrophilicity, can facilitate better tissue penetration.
A deeper understanding of the physical properties of tissues and probes allows researchers to enhance their experimental setups. Balancing the need for effective penetration against the risk of damaging cellular integrity is essential for successful SmFISH probe studies.
Future Perspectives of SmFISH Probes
The continued evolution of molecular biology often hinges on innovations in probe technology. SmFISH probes stand at the forefront of this advancement. Their unique capability to visualize RNA molecules at a single-molecule level can drastically improve our understanding of gene expression and regulation, providing significant insight into cellular processes. As research progresses, identifying what lies ahead for SmFISH probes becomes crucial. The growing complexity of biological systems demands more refined tools for accurate analysis.
Emerging Technologies
Recent advancements in imaging technologies are set to enhance the efficacy of smFISH probes. Techniques such as super-resolution microscopy can provide even greater spatial resolution for visualizing RNA. The combination of smFISH with techniques like CRISPR imaging allows for dynamic tracking of RNA in living cells, enabling researchers to observe real-time transcript behavior and localization. This fusion of technologies can yield rich datasets that not only broaden our understanding of cellular function but also facilitate discovery in various fields like developmental biology and genetics.
Moreover, machine learning algorithms can assist in analyzing large amounts of imaging data, leading to improved accuracy in identifying and quantifying RNA molecules. This integration opens avenues for automated analysis, which saves time and enhances reproducibility.
Integration with Other Techniques
The strength of smFISH can be amplified through its integration with other experimental approaches. When combined with RNA sequencing, researchers can compare the results of transcript abundance with localization data. This multi-faceted approach can deliver a more holistic view of gene expression dynamics.
Integrating smFISH with immunofluorescence can also prove valuable. By simultaneously visualizing RNA and protein targets, scientists can investigate the relationship between transcript levels and protein production, providing insights into post-transcriptional regulation.
The ability to visualize RNA and protein simultaneously can unravel complex interactions within the cellular environment, fostering a deeper understanding of biochemical pathways.
As the landscape of molecular biology transforms, the future of SmFISH probes will likely shape new experimental paradigms. This merging of technologies underlines how critical it is for researchers to stay informed about advancements, ensuring they utilize the best methods available for their inquiries.
End
Understanding SmFISH probes brings significant insight into molecular biology's evolving landscape. With their unique ability to enable single-molecule detection, these probes have enhanced our comprehension of gene expression and cellular mechanisms. The intricate design of SmFISH probes is fundamental in yielding accurate and reproducible data. Each component, from sequence specificity to labeling techniques, plays a critical role in their effectiveness.
Summary of Key Insights
The discussion surrounding SmFISH probes has highlighted several key elements:
- Precision: SmFISH probes allow for high-resolution detection at the single-molecule level. This can reveal spatial and temporal gene expression patterns that are otherwise obscured using bulk methods.
- Applications: Whether in gene expression analysis, studying RNA localization, or investigating dynamic cellular processes, SmFISH applications are broad and increasingly vital.
- Challenges: As discussed, signal variability and tissue penetration remain challenges. Addressing these issues is crucial for maximizing the potential of SmFISH in diverse biological contexts.
Implications for Future Research
Looking ahead, the implications of SmFISH probe research are vast:
- Technological Advancements: Emerging technologies promise to enhance the capabilities of SmFISH. Developments in imaging techniques, for example, may further increase sensitivity and reduce background noise.
- Interdisciplinary Integration: The future of SmFISH lies in its integration with other molecular techniques. Combining RNA sequencing and in situ hybridization can provide a more robust understanding of gene regulation mechanisms.
- Broader Applications: As researchers continue to explore complex biological questions, SmFISH may find new applications in developmental biology, neurology, and cancer research. This versatility will drive innovations in how we approach problems in molecular biology.
Through these insights, it is clear that SmFISH probes will continue to influence the trajectory of scientific inquiry. As our understanding deepens, their role in advancing the field will become more pronounced, forever altering how we study the biological world.