Understanding the Scientific Method: A Complete Guide
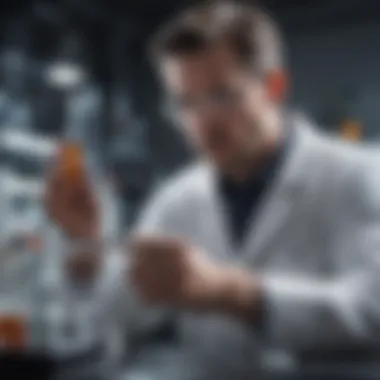
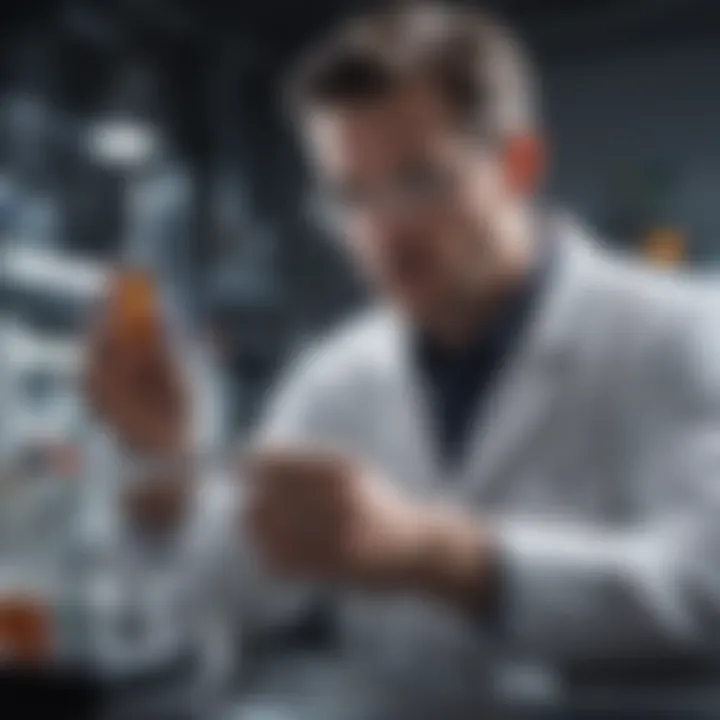
Background and Context
The scientific method is a time-tested framework that has guided researchers through the maze of inquiry, facilitating answers to some of the most profound questions about our existence and the universe at large. It's not just a checklist of steps; itās a living, evolving narrative that has stood the test of time, adapting to new findings and incorporating technological advancements.
Overview of the Research Topic
The scientific method consists of various stages, beginning with observation and proceeding through hypothesis formation, experimentation, and analysis. Each phase serves as a building block for understanding complex phenomena across fields such as biology, physics, and social sciences. While the methodology might seem straightforward, the depth of analysis and breadth of application underscore its undeniable significance.
Historical Significance
The roots of the scientific method can be traced back to ancient philosophers like Aristotle, who championed observation and logical reasoning. Fast forward to the 17th century, and we see pioneers like Galileo and Bacon formalizing these concepts into an organized approach. They laid down rigorous protocols for experimentation and established the importance of replicability in scientific inquiries. Over centuries, empirical findings replaced speculation, keeping pace with advancements in society and knowledge. Today, we stand on the shoulders of giants, using their foundations to explore everything from quantum mechanics to climate change.
Key Findings and Discussion
In peeling back the layers of the scientific method, we can identify the rational structure that supports scientific breakthroughs.
Major Results of the Study
Research abounds in emphasizing the practical application of the scientific method. From the groundbreaking studies in medicine that paved the way for vaccines to ecological research informing conservation efforts, the methodās influence is far-reaching. As we unpack its stages:
- Observation: This isn't just about what we see. It involves looking for trends or anomalous behavior in data, all while keeping cognitive biases at bay, ensuring clear sight.
- Hypothesis: Here, thinkers propose possible explanations or predictions based on observations. But itās not the end-all; hypotheses should be flexible enough to embrace new evidence.
- Experimentation: This phase can be laborious. It involves testing hypotheses through controlled and replicated tests. The methodology here can vary widely from field to field.
- Analysis: Finally, once the results come in, they must be scrutinized for reliability. Are they replicable? What do the statistics indicate about the strength of the findings?
Detailed Analysis of Findings
As we consider the component states, it becomes clear that this method is not meant for just scientists in lab coats. Educators, policymakers, and curious minds alike can harness its principles to enrich understanding in everyday life. Take, for example, the health field where epidemiologists use rigorous epidemiological principlesāoften rooted in the scientific methodāto track diseases and inform public health responses.
"The scientific method is the best tool we have for understanding the world; it pushes boundaries, fosters curiosity, and establishes a paradigm for inquiry."
The adaptability of the scientific method also addresses many misconceptions that swirl around scientific inquiry. It's not a step-by-step tutorial; instead, it embodies a circle of continuous questioning and revision.
In summation, the scientific method is not only a beacon of clarity in the quest for knowledge but also a testament to humanity's enduring quest for truth. It remains a dynamic, accessible process, ready to guide new generations of thinkers who dare to ask questions and seeks to unearth the layers of reality.
The Foundation of Scientific Inquiry
Defining the Scientific Method
The scientific method can be defined as a structured, logical process used to formulate hypotheses, conduct experiments, and analyze results. It begins with observation, where scientists look for phenomena to investigate. This is followed by research to gather background knowledge. After that, a hypothesis is formulated, laying down a testable prediction. The next stage involves experimentation, where variables are carefully controlled to establish cause-and-effect relationships. Finally, results are analyzed and conclusions drawn to either confirm or refute the initial hypothesis.
In sum, defining the scientific method sets a clear framework that maintains the integrity and rigor of scientific research.
Historical Perspectives
The evolution of the scientific method is not just a chronicle of procedural milestones; itās a tapestry woven with the contributions of brilliant minds who paved the way for modern science.
Key Figures in the Evolution
Key figures such as Galileo Galilei, Sir Isaac Newton, and Francis Bacon played pivotal roles in shaping the foundations of the scientific method. Their contributions were characterized by an unprecedented emphasis on empirical evidence and experimentation. For instance, Galileo's work with telescopes not only unveiled celestial bodies but also challenged existing beliefs, setting a precedent for questioning established doctrines. This iconoclastic approach fostered a culture of inquiry that remains vital in contemporary research.
One distinct characteristic of these figures is their relentless pursuit of knowledge through questioning and observationāan aspect thatās beneficial for understanding the evolution of the scientific method. Their insistence on systematic experimentation solidified the notion that knowledge is not static but a dynamic process of discovery.
Milestones in Scientific Thought
Milestones in scientific thought have marked significant advancements in the understanding of natural phenomena. The publication of Newton's "PhilosophiƦ Naturalis Principia Mathematica" in 1687 is a prime example, which formalized the laws of motion and universal gravitation. Such works represent not merely individualsā achievements but significant shifts in how humanity comprehends the universe.
A key characteristic of these milestones is their ability to distill complex ideas into understandable principles, making them accessible to a wider audience. This accessibility is a double-edged sword; while it prompts broader engagement with scientific thought, oversimplification can sometimes lead to misunderstandings.
In summary, both key figures and milestones in scientific thought play a significant role in addressing how we understand and apply the scientific method today. Each piece of this historical puzzle underscores the methodās foundational importance, guiding todayās researchers, students, and educators in their quest for knowledge.
Stages of the Scientific Method
The stages of the scientific method form the backbone of scientific inquiry. Each step is essential, operating as a cog in a well-oiled machine designed to explore questions about the world. By comprehensively understanding these stages, one can appreciate how scientists accumulate, refine, and validate knowledge. Gaining insights from this process benefits researchers and curious minds, as it enhances analytical thinking and critical reasoning abilities.
Observation and Research
Gathering Information
Gathering information is the first step, where raw data is collected from various sources. This isn't just about taking notes; it's about immersing oneself in the settings of interest to gain foundational understanding. Whether itās through field studies, literature reviews, or surveys, gathering information provides the groundwork for all subsequent steps in the scientific journey. The significant characteristic of this stage is the notable diversity of sources. This aspect makes it a powerful tool, as it allows for a richer canvas of knowledge upon which hypotheses can be built.
One unique feature of gathering information is its adaptability. Researchers need to chase leads and adapt their sources based on what they find. However, thereās a drawback: if not done meticulously, researchers risk biases creeping into their collection methods, potentially skewing the data.
Identifying Patterns
Once information is gathered, identifying patterns comes into play. This isnāt just recognizing shapes in clouds; it's about uncovering trends, correlations, and anomalies in the data. Recognizing patterns helps scientists to understand existing connections and prepares the ground for hypothesizing. This characteristic makes it crucial; it transforms raw data into potential theories.
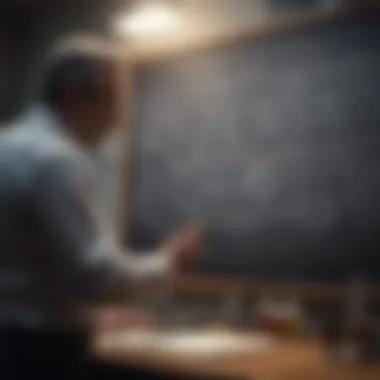
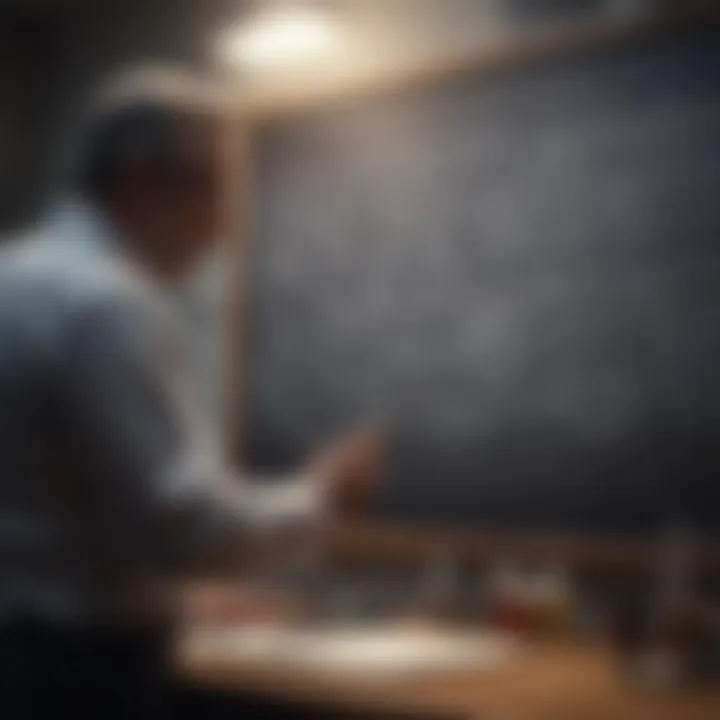
The unique feature of identifying patterns lies in its capacity for creativity. It requires both analytical skills and a degree of intuition. However, thereās a cautionary note: patterns identified may be mere coincidences, and hence the risk of jumping to conclusions must be carefully managed.
Formulating Hypotheses
Characteristics of a Good Hypothesis
A well-structured hypothesis serves as a guiding principle for experimentation. It should be clear, testable, and specific, framing the inquiries in a manner that can lead to empirical validation. The key characteristic here is clarityāwithout it, the scientific journey can veer off course. A good hypothesis not just proposes a possible explanation but sets the groundwork for probing deeper.
One unique aspect of a solid hypothesis is its flexibility; as new data emerge, it can evolve. However, a disadvantage is that if itās framed too narrowly, it can limit exploration and might restrict the scope of investigation.
Testability and Falsifiability
Testability and falsifiability underpin the experimental aspect of scientific inquiry. A hypothesis must be structured such that it can either be confirmed or disproven through experiments. This is crucial; a hypothesis that canāt be tested is often deemed unscientific. The highlighted characteristic is its objective nature, guiding researchers on how to design experiments effectively.
The unique feature of this stage is also the clear definition of success. If data supports a hypothesis, it suggests a viable explanation; if not, itās an equally important insight. The trick, however, is to recognize that failing to prove a hypothesis isnāt a defeatāit's a stride toward knowledge.
Conducting Experiments
Types of Experiments
Conducting experiments comes in various flavorsācontrolled, field, and natural experiments, each serving specific scientific purposes. The key characteristic is the breadth of approaches available. Different sciences require different types of experimentation to answer unique questions or test individual hypotheses.
The unique feature of this stage is that it enables hypothesis testing in real-world scenarios or controlled environments. This offers great flexibility in research design. However, the downside can be significant. Some experiments are simply impractical or unethical, which can hinder progress in certain fields.
Controlled vs. Uncontrolled Variables
Understanding controlled and uncontrolled variables is foundational to scientific experiments. Controlled variables ensure consistency, while uncontrolled variables can introduce chaos into results. The key characteristic of controlled variables is that they create a stable testing environment, allowing researchers to focus on the relationship between variables.
One uniqueness of this aspect is that it encourages rigor in research design. Keeping a fixed point amidst changing variables is what lends credibility to observations. But a drawback is that excessive control can lead to oversimplifications, stripping experiments of real-world complexity.
Analysis of Results
Data Interpretation
Once experiments yield results, data interpretation emerges as a critical step. Making sense of numbers isnāt about just crunching statistics; itās translating findings into understandable insights. The key characteristic is that this stage requires both analytical skills and creativity in reasoning.
A unique feature here is the potential for multiple interpretations; one set of data can lead to different conclusions depending on context. However, this subjectivity can also be a slippery slope toward bias, leading to questionable claims if not approached with diligence.
Statistical Relevance
Statistical relevance ensures that findings arenāt just random occurrences but reflect genuine relationships in the data. The key characteristic is its objectivityāstatistical tools provide a means to quantify uncertainty.
A notable aspect is how this lends authority to research; relevant statistics often bolster claims. Yet, the flip side is the risk of over-reliance on statistical significance without considering the practical implications or context of the findings.
Drawing Ends
Validating Hypotheses
Validating hypotheses is about confirming if a hypothesis holds when tested against empirical results. The key characteristic is that this fosters confidence in scientific knowledge, paving the way for broader acceptance of theories.
One unique feature is that validation can also lead to new avenues of inquiry. When hypotheses stand, they often reveal deeper insights worth exploring further. However, too much emphasis on validation can sometimes cloud the quest for alternative truths which might be equally informative.
Implications of Findings
The implications of findings extend beyond immediate results; they influence future research directions and societal understanding. The key characteristic is the potential for broader impact. A simple experiment can lead to vast advancements in understanding, technology, or health practices.
A distinctive aspect of this step is that it often necessitates interdisciplinary collaboration, as implications may resonate across various fields. Nevertheless, the risk lies in misinterpreting significance or making overstretched conclusions that might mislead.
In the stages of scientific inquiry, each step builds upon the last, ultimately yielding knowledge that is robust, thorough, and significant.
The Importance of Replicability
Replicability stands as a cornerstone in the realm of scientific research. It refers to the ability to repeat an experiment or study under the same conditions and obtain similar results. When results are replicable, it lends credence to the findings, suggesting that they are not mere flukes but rather reflect a fundamental truth about the phenomenon in question. The significance of replicability stems from several aspects. It enforces a rigorous standard, promotes confidence in scientific claims, and helps to filter out unreliable research. Researchers who build upon replicable studies can do so with a greater degree of assurance, which is crucial for advancing knowledge across various fields.
Understanding Replication
Replication, at its core, embodies the principle of scientific rigor. It is not just about redoing an experiment; it's about verifying findings to ensure that they are dependable. A well-replicated study is like a sturdy bridge, built upon a solid foundation of truth rather than flimsy materials that could collapse under scrutiny. In the scientific community, when an experiment is replicated successfully, it acts like a badge of honor, affirming the original researcher's claims. However, the process isn't always straightforward. Factors such as variations in methodology, sample size, and environmental conditions can influence the outcome, complicating the replication efforts.
Impact of Non-Replicable Results
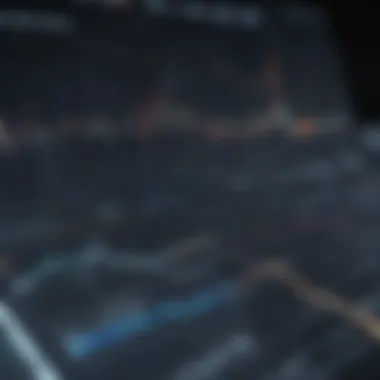
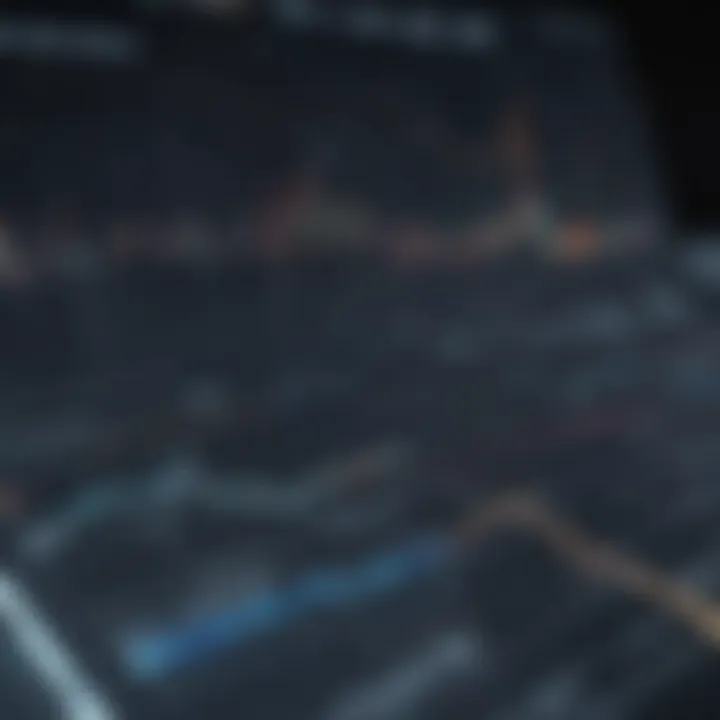
Non-replicable results can have serious consequences, casting shadows on the integrity of science itself. When findings fail to be reproduced, it raises questions about the original study's validity. This lack of replication can lead to a crisis of confidence not only in specific research but in the broader scientific institution. Scientists and laypeople alike may start to wonder whether the discipline can indeed produce reliable knowledge.
Case Studies
Case studies often serve as illustrative examples of the ripple effects caused by non-replicable results. A stark instance is the initial studies suggesting links between vaccines and autism, which, despite being highly publicized, could not be replicated. This led to widespread misinformation, vaccine hesitancy, and public health risks. The crucial characteristic of case studies is their capacity to highlight real-world consequences of research claims, emphasizing the importance of replicability in maintaining scientific credibility. While case studies offer detailed insights into specific instances, they can also be limited in scope, prompting researchers to tread carefully when generalizing findings.
Consequences for Scientific Progress
The consequences of non-replicable results extend far beyond individual studies; they can hinder the progress of science as a whole. When scientists cannot trust that their predecessors' findings are accurate, it stalls further exploration and innovation. The key characteristic of these consequences is that they can perpetuate cycles of uncertainty within the community, leading researchers to question established paradigms. If foundational studies are found to be erroneous, it could set back years of scientific advancement.
Common Misconceptions
Understanding common misconceptions about the scientific method is essential for grasping its true nature. Misconceptions can undermine the legitimacy of scientific inquiry and skew public perception. They can lead to a misunderstanding of what science truly entails and how it operates in the real world. Recognizing these misconceptions allows students, researchers, educators, and professionals to foster a more nuanced comprehension of scientific practices.
The Misunderstanding of Scientific Certainty
One prevalent fallacy surrounding science involves the notion of certainty. Many laypeople perceive scientific findings as absolute truths. However, this perspective does not truly reflect how science operates. In reality, scientific theories are always subject to revision in light of new evidence. One might say scientific understanding is more like a landscapeāit shifts and changes with the passing of time and the coming of new insights.
Consider the progression of the theory of gravity. What Isaac Newton proposed in the 17th century was revolutionary but not completely exhaustive. Albert Einsteinās theory of relativity later expanded on Newtonās work, providing a clearer picture of gravity in the context of spacetime. Thus, itās essential to understand that science does not rest on the pedestal of certainty. Instead, it continually seeks to refine theories based on new data, ideas, and observations.
"Science is not the accumulation of facts, but rather a never-ending search for understanding."
This fundamental shift in perception is important. It promotes intellectual humility and encourages critical thinking. Rather than cling to preconceived notions, one must remain open to revising perspectives based on ongoing investigations and results. It's through this iterative process that science advances.
Science is Just a Collection of Facts
Another widespread misconception is the belief that science is merely a pile of facts gathered together. Some may think that once a piece of information is established, it remains static. This viewpoint is misleading, as it overlooks the dynamic and iterative nature of scientific inquiry.
Science requires more than simply memorizing data points or isolating pieces of informationāit calls for interpretation and analysis. Just because a phenomenon has been observed doesn't mean we understand it fully. Theories arise out of consistent data patterns that can explain and predict outcomes in the natural world.
For instance, consider the field of chemistry, where understanding chemical cycles or reactions requires more than knowing individual elements. The relationships between elements, compounds, and the processes they undergo create a web of knowledge that informs the entire discipline. Here are some aspects to consider about the nature of science:
- Contextual Understanding: Facts gain significance within their context. Isolated data lacks meaning without interpretation and relation to existing knowledge.
- Evolving Understanding: Scientific knowledge evolves with each new discovery, and past truths can be reassessed.
- Predictive Power: Theories in science not only describe reality but also make predictions about future occurrences based on established principles.
In summary, misconceptions about the scientific method can cloud understanding and lead to misinterpretation of scientific endeavors. It's essential to instill a clear vision of what science is, grounded in continual inquiry and evolution, rather than merely a collection of facts.
Interdisciplinary Applications
The concept of interdisciplinary applications in science shines a light on how different fields can intersect and enhance one another. This approach facilitates a comprehensive understanding of complex issues which often cannot be fully addressed by any single discipline. By leveraging diverse methodologies, theories, and tools from various academic realms, researchers can illuminate patterns and solutions that traditional methods might overlook. The benefits of this approach are numerous, including innovation through collaboration and a broader perspective on scientific challenges.
Application in Biology
Case Studies
Case studies serve as a pivotal aspect in the realm of biology, contributing a rich narrative to the scientific method. Through in-depth exploration of specific instances, they allow scientists to delve deep into real-world phenomena. They can reveal insights that statistical data alone cannot capture. For example, the case study of the GalƔpagos finches not only demonstrated natural selection but also opened a treasure chest of knowledge on adaptation in biology. However, these studies have a unique feature: they are often limited in generalizability. While they can illuminate specific scenarios, their findings may not always apply broadly across different contexts, leading to potential pitfalls in interpretation.
Real-World Implications
The implications of biological inquiries resonate well beyond the lab. They change lives, open doors to new medical treatments, and enhance our understanding of ecosystems. For instance, research on antibiotics from soil bacteria has real-world applications in combating infections that threaten public health. A key characteristic of these implications is their immediate relevance; they not only inform scientific theory but also lead to tangible benefits for society at large. Yet, the reliance on real-world applications can sometimes overshadow the underlying scientific principles, risking the integrity of the conclusions drawn.
Application in Chemistry
Experimental Techniques
In chemistry, experimental techniques are the backbone of scientific discovery. They involve a variety of methods, from titration to spectroscopy, which scientists use to observe reactions and gather data. The rigorous nature of these techniques ensures controlled conditions, leading to consistent and reliable results. A notable aspect is their adaptability; they can be tailored to fit specific research questions or contexts. However, the complexity of these methods can be a double-edged sword, as they often require significant resources and expertise to implement effectively.
The Role of Chemistry in Scientific Inquiry
Chemistry is often described as the central science, and rightly so. It's the glue that binds various scientific disciplines, linking biology and physics in a way that allows holistic understanding. The role of chemistry extends its reach into fields like pharmacology and environmental science. Its importance lies in its capacity to inform and refine scientific inquiry across the board. However, the nuanced nature of chemical phenomena can sometimes create a barrier to understanding, making interdisciplinary communication crucial but challenging.
Application in Physics
Notable Experiments
Notable experiments in physics showcase the elegance and power of the scientific method. For example, the double-slit experiment not only elucidates aspects of wave-particle duality but also highlights the profound philosophical questions surrounding observation itself. A significant characteristic of these experiments is their ability to provoke new inquiries; each investigation paves the way for a multitude of others. Nonetheless, the high level of abstraction in physics experiments can alienate those not steeped in the discipline, underscoring the necessity for effective communication.
Quantum Mechanics and the Scientific Method
Quantum mechanics poses unique challenges to the scientific method, bringing with it a wave of complexity. This field revolutionizes how scientists understand reality at the micro level, often defying common sense. The interplay between quantum mechanics and the scientific method reveals the need for flexibility in theoretical frameworks and methodologies. While the rigor of the scientific method remains essential, quantum physics often requires a rethinking of traditional approaches, representing both an exciting and daunting frontier for researchers.
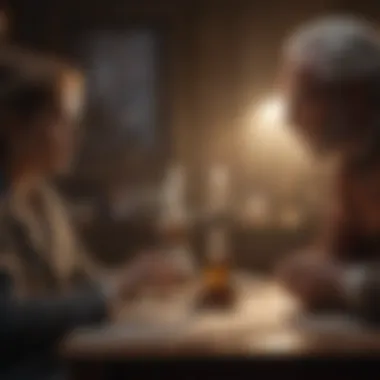
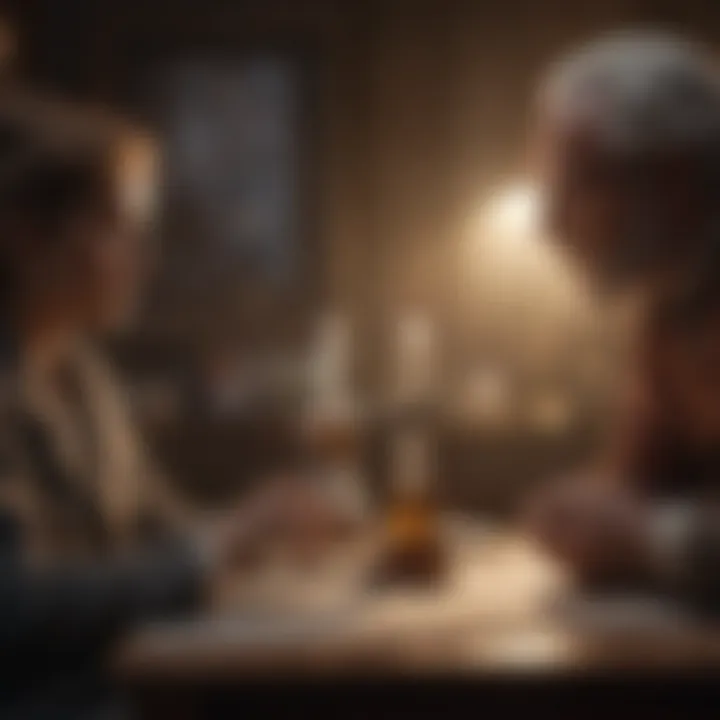
Application in Earth Sciences
Environmental Research
Environmental research stands as a hallmark of interdisciplinary work, where biology, chemistry, and geology converge to address pressing global challenges. This research not only emphasizes sustainability but also delves into the intricate relationships between human activity and natural ecosystems. The immediate relevance of environmental outcomes fuels passion in this field, appealing to a wide range of stakeholders. However, translating scientific findings into actionable policies can often face political and social hurdles, complicating the path from research to real-world impact.
Geological Studies
Geological studies offer a unique vantage point on Earth's history and dynamics through the lens of the scientific method. By studying rock formations and fossil records, scientists draw connections between past events and current conditions, providing insights into climate change and resource management. The key characteristic of geological studies is their long-term perspective, fostering an understanding that transcends the immediate confines of human time. However, the vast scales of geological time can make it challenging to relate findings directly to pressing contemporary issues.
Challenges in Scientific Research
Scientific research, while essential in furthering our understanding of the universe, is fraught with numerous challenges. These hurdles can significantly affect the outcomes and reliability of research. By looking into challenges such as funding limitations, ethical considerations, and their implications, we can engage with the nuances that make the scientific process both intricate and crucial.
Funding and Resource Limitations
One of the foundational challenges that scientists often face is securing adequate funding. Without financial backing, conducting experiments and gathering data becomes a Herculean task.
In an era where resource allocation in academia and industry is under strict scrutiny, researchers frequently grapple with limitations that can hinder progress. This has several implications:
- Inaccessibility of Equipment: Advanced instruments and tools that are vital for exploration may remain out of reach, forcing scientists to use outdated technology.
- Restricted Personnel: A shortage of funds can limit the number of researchers and assistants on a project, which in turn, can slow progress and reduce the quality of the work done.
- Impact on Research Scope: Researchers might be compelled to limit their inquiries to low-cost options, often sacrificing depth and comprehensive understanding in favor of feasibility.
Ethical Considerations
Scientific integrity is paramount, yet the ethical challenges presented by modern research cannot be overlooked. These considerations guide researchers in ensuring that their studies do not result in harm. Two critical areas within this realm are human and animal research ethics, as well as the overarching concept of scientific integrity.
Human and Animal Research Ethics
The ethics surrounding research involving humans and animals forms a cornerstone of responsible scientific inquiry. This aspect addresses critical issues regarding consent, welfare, and rights. Some key characteristics include:
- Informed Consent: Participants must be fully aware of the study details, understanding potential risks and benefits before agreeing to participate.
- Welfare Considerations: Ensuring minimal suffering for animal subjects is a non-negotiable aspect.
- Oversight Mechanisms: Regulatory bodies and ethics committees play an essential role in assessing proposals, ensuring that research standards uphold moral principles.
The unique feature here is that ethical research often enhances public trust in science. However, it can sometimes extend project timelines, as obtaining approvals and following protocols might result in bureaucratic delays.
Scientific Integrity
Scientific integrity encompasses the norms and standards that researchers are expected to uphold. These values are not just words on a paper, they ensure the credibility of research. Some core points include:
- Transparency: Clear sharing of methods and results fosters an open environment where findings can be scrutinized and validated by others.
- Accountability: Researchers must take responsibility for their work, especially when it comes to data integrity.
- Honesty: Misrepresentation of data or using fabricated results not only misleads but can have far-reaching implications for public policy and perception.
The unique aspect here lies in the way these principles protect the scientific enterprise from fraud. Though adherence to integrity standards can initially seem burdensome, the long-term trust and progress it fosters within the scientific community make it worth the effort.
"A culture of ethical research not just protects participants, it enriches science itself."
Thus, by examining these challenges ā be it resources or ethical dilemmas ā it becomes evident that progress in scientific inquiry requires navigating a maze of limitations. But, without addressing these obstacles, the pursuit of knowledge could be significantly stymied.
Future Directions in Scientific Inquiry
Scientific inquiry is a living, breathing organism, evolving with each passing day. As we stand at the precipice of a new era in research and development, understanding the future directions of the scientific method is paramount for fostering innovation. More than just a collection of techniques, these directions integrate emerging technologies and interdisciplinary collaborations, both paving the path toward revolutionary breakthroughs in various fields.
Emerging Technologies
In the realm of scientific inquiry, technology is akin to a double-edged sword. On one hand, it enhances our capabilities; on the other, it introduces complexities we must navigate. Emerging technologies, including artificial intelligence, machine learning, and big data analytics, reshape how researchers gather data and conduct experiments. Instead of hammering away at traditional methods alone, scientists now have tools that can analyze vast amounts of information in a fraction of the time.
For example, artificial intelligence can sift through millions of scientific papers, identifying trends and connections that might evade even the most astute researchers. This capability not only streamlines the research process but also sparks new ideas, leveling the playing field for smaller teams without extensive resources. Additionally, automation in laboratories increases efficiency, allowing scientists to focus on critical analysis instead of mundane tasks.
Interdisciplinary Collaboration
Benefits of Cross-Disciplinary Research
Collaboration across disciplines can be viewed as the spice in the stew of scientific inquiry. When two or more areas of expertise come together, they create a rich tapestry of knowledge that can lead to innovative solutions. In this modern era, scholars and practitioners no longer silo themselves. Instead, they bring together diverse perspectives, often producing results that are more robust than single-discipline studies could achieve alone.
In this regard, cross-disciplinary research is seen as a most beneficial choice because it often leads to out-of-the-box solutions. For example, a partnership between biologists and computer scientists can result in improved algorithms for predicting biological responses, enhancing drug discovery efficiency.
Unique features of cross-disciplinary collaboration include the interchange of methodologies and perspectives, which can refine existing theories and introduce brand new narratives into scientific conversations. However, such collaborations necessitate effective communication, as jargon can sometimes create barriers instead of bridges.
Examples of Successful Collaborations
Successful collaborations often serve as the hallmark of innovation in scientific research. One of the standout examples is the Apollo program, where physicists, engineers, and other experts combined their talents to land a man on the moon. This monumental achievement signified not merely a technological triumph but underscored how converging diverse expertise can achieve extraordinary feats.
Similarly, the Human Genome Project showcased cooperation among biologists, computer scientists, and ethicists. This blend of skills produced a comprehensive map of human DNA, yielding insights into disease mechanisms and forging the way for personalized medicine.
What makes these collaborative efforts striking is their ability to address complex problems that one discipline alone struggles to unravel. Yet, such partnerships also come with their own set of challenges, particularly in aligning goals and methodologies, which can lead to tension if not managed properly.
In a world that's increasingly interconnected, understanding these emerging trends in scientific inquiry is not just educational ā itās a necessity for shaping the innovations of tomorrow. Crossing boundaries, both disciplinary and technological, allows scientific inquiry to not just advance; it allows it to flourish.