Understanding RNA Integrity Number in RNA-Seq

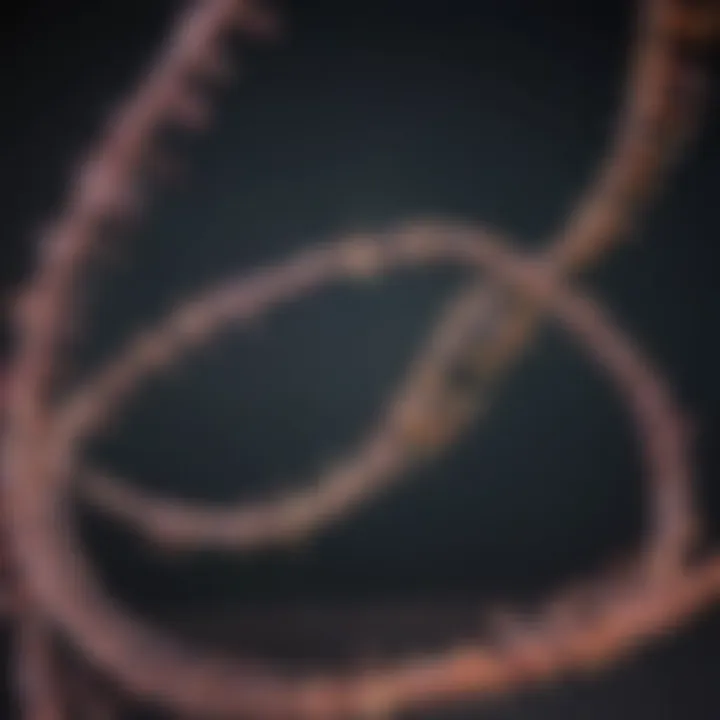
Intro
RNA sequencing, often called RNA-Seq, is a game changer in molecular biology. It opens the door to a treasure trove of information regarding gene expression, alternative splicing, and much more. But to make the most of this powerful technique, one must pay close attention to RNA quality, and that's where the RNA Integrity Number, or RIN, comes into play. RIN serves as a crucial benchmark for assessing the integrity of RNA samples prior to sequencing, ensuring that the resulting data are reliable and informative.
Understanding RIN isn’t just about a number; it’s about grasping its implications and applications in RNA-Seq. To truly appreciate its significance, one mustn't overlook the essentials surrounding this concept.
Background and Context
Overview of the research topic
In the fast-paced world of genomic research, the ability to accurately quantify RNA gives scientists an edge in understanding biological processes. RIN was developed as a straightforward metric to assess the integrity of total RNA. Average values range from 1 to 10, where 10 indicates pristine, intact RNA. The genesis of this measure is a response to the growing need for reliable indicators amid an abundance of RNA extraction techniques and protocols that can compromise sample quality. RIN relies on automated capillary electrophoresis, measuring the sizes of RNA fragments and their respective intensities.
Historical significance
RIN was introduced not long after the emergence of RNA-Seq as a mainstream technique. Historically, RNA quality assessment methods were largely qualitative and sometimes subjective. Techniques such as gel electrophoresis certainly played their part, but they often fell short in providing concrete metrics. The advent of RIN marks a shift toward standardized criteria in RNA quality assessment. Its role has only solidified over time as high-throughput sequencing technologies rapidly evolved.
The introduction of RIN has been particularly impactful in the research community focusing on gene expression studies. In a world where results can pivot on the smallest variations, ensuring RNA integrity can be the thin line between groundbreaking findings and inconclusive data. Therefore, grasping RIN amidst the broader context of RNA-Seq not only enriches research methodology but enhances the reliability of the scientific conclusions drawn from these studies.
Preface to RNA-Seq and its Significance
RNA sequencing, or RNA-Seq, has become an essential tool in molecular biology, allowing researchers to probe into the depths of gene expression studies, transcriptomics, and beyond. Its significance lies not merely in its ability to sequence RNA but in the insights it provides into the intricate workings of cells. Understanding how RNA interacts, its stability, and the overall quality can vastly influence experimental outcomes, making the RNA Integrity Number (RIN) a vital consideration in RNA-Seq methodologies.
Overview of RNA-Seq Technology
RNA-Seq is a high-throughput sequencing technique that offers a snapshot of the transcriptome. Unlike traditional methods, RNA-Seq analyzes the entire range of RNA molecules in a sample, from protein-coding transcripts to non-coding RNAs. This comprehensive view is made possible by converting RNA into complementary DNA (cDNA) before sequencing, allowing for detailed mapping and quantification.
Researchers can utilize RNA-Seq to:
- Identify novel transcripts: This encompasses discovering new genes or variants.
- Quantify gene expression levels: RNA-Seq offers dynamic ranges of expression, enabling insights into gene regulation under various conditions.
- Detect alternative splicing: Understanding how a single gene can give rise to multiple products is crucial for grasping complex biological systems.
By embracing RNA-Seq technology, scientists gain a nuanced understanding of cellular responses that can lead to discoveries in fields ranging from cancer research to developmental biology.
Significance of High-Quality RNA in Research
The adage “garbage in, garbage out” rings especially true in the realm of RNA-Seq. The quality of RNA extracted from samples directly influences the accuracy and reliability of the resultant data. RNA is notoriously fragile and can degrade quickly due to environmental stressors or improper handling.
For those working tirelessly in laboratories, the implications of using low-quality RNA can hardly be overstated. Among the most critical considerations:
- Inaccurate gene expression profiles: Low-quality RNA may yield unreliable results, leading to erroneous biological interpretations.
- Biological confounding: Variations within RNA quality can obscure true biological signals, complicating analyses and skewing results.
- Increased experimental costs: The need to rerun experiments due to low-quality RNA introduces redundancy, wasting both time and resources.
In essence, maintaining high RNA quality is not just a technical requirement but a cornerstone for gaining meaningful insights into biological processes.
Defining RNA Integrity Number (RIN)
In the realm of molecular biology, understanding the RNA Integrity Number—or RIN—is pivotal for ensuring that RNA sequencing (RNA-Seq) yields reliable results. RIN serves as a gauge for the integrity and quality of RNA extracted from biological samples. When diving into RNA-Seq experiments, researchers must grasp why RIN matters. Poorly characterized RNA can skew outcomes, leading to erroneous interpretations and potentially flawed conclusions.
One can view RIN not merely as a number but as a key indicator reflecting the suitability of RNA for downstream applications. Issues like degradation can drastically affect the fidelity of data obtained through sequencing. Therefore, comprehending RIN helps safeguard the accuracy and reproducibility of scientific findings in various research fields, including genomics and transcriptomics.
Conceptual Framework of RIN
RIN is a calculated metric that stems from the electrical patterns observed during gel electrophoresis of RNA. This number ranges from 1 to 10, where 1 indicates completely degraded RNA and 10 reflects pristine, highly intact RNA. In many ways, RIN can be likened to a health check-up for RNA: if your samples sport a low RIN, it's akin to getting a bad report from the doctor.
The calculation of RIN incorporates factors such as the presence of ribosomal RNA bands, their relative intensity, and the overall RNA degradation footprint. As RNA degrades, certain patterns emerge—primarily a decrease in the ribosomal RNA (rRNA) bands. The more intact the rRNA, the higher the RIN value will be. This quantitative analysis supports decisions throughout experimental protocols and can dictate sample suitability for specific applications.
RIN as a Measure of RNA Quality
Using RIN as a measure of RNA quality presents clear advantages. A higher RIN indicates that the RNA sample is of better quality, which is crucial for any downstream applications, including cDNA synthesis and sequencing. In practical terms, a sample with a RIN of 8 or above typically signifies that the RNA is likely fit for high-quality sequencing outcomes.
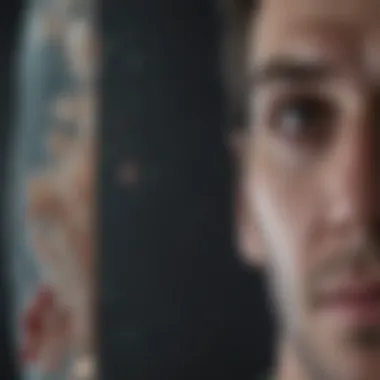
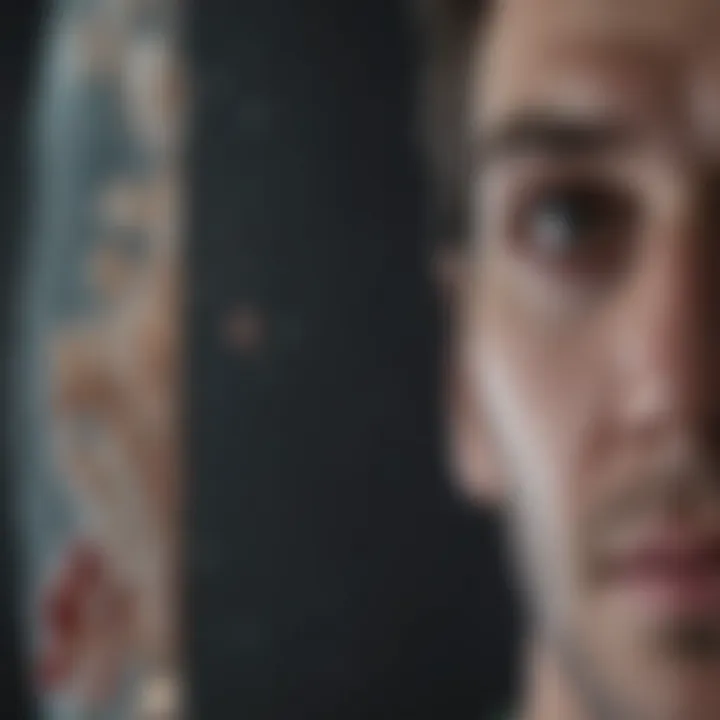
However, one must be cautioned against becoming overly reliant on RIN alone. While a high RIN might suggest good integrity, it doesn't always correlate directly with biological relevance. For example, a degradation level might not be reflected in a straight numerical measurement if the RNA still harbors significant biological information. Therefore, combining RIN with other quality metrics provides a more comprehensive perspective.
"RNA integrity is not just a number; it's a critical dialogue between molecular quality and experimental fidelity."
Ultimately, understanding RIN—along with its implications and limitations—enriches researchers’ appreciation of RNA quality metrics. This clarity bolsters experimental design and interpretation, laying the groundwork for accurate research results that can lead to robust scientific discourse.
Methodologies for RIN Assessment
Assessing the RNA Integrity Number (RIN) is crucial for ensuring the fidelity of RNA sequencing (RNA-Seq) experiments. Various methodologies are employed in this assessment, each with its unique strengths and limitations. The choice of technique can have significant implications for the quality of downstream analyses, making it imperative to consider several factors, such as the nature of the sample, the desired accuracy, and available resources. By understanding the various methodologies available, researchers can make informed decisions that ultimately enhance the integrity of their research findings.
Electrophoretic Techniques for RIN Measurement
Electrophoresis has long stood as a cornerstone in molecular biology, especially for RNA analysis. The technique leverages an electric field to separate RNA molecules based on size and charge. This separation allows a clear visual representation of RNA quality, which is key when calculating RIN. High-quality RNA tends to show distinct bands with minimal smearing, while degraded RNA often appears as a diffuse smear.
- Advantages:
- Visual Clarity: The bands provide immediate insight into the RNA's integrity.
- Cost-effectiveness: Generally, these techniques require less expensive equipment compared to some modern techniques.
However, the interpretation of results can be subjective and heavily relies on the operator's expertise. This subjectivity may introduce variability across different assays, which can impact the overall reliability of RIN assessments.
Spectrophotometric Methods
Spectrophotometry is another widely used technique for determining RNA concentration and purity, indirectly inferring its quality. The method involves measuring the absorbance of RNA at specific wavelengths, particularly at 260 nm and 280 nm. The ratio of absorbance at these two wavelengths can indicate the presence of contaminants and, by extension, RNA degradation.
- Utility:
- Speed: This technique is relatively quick, often yielding results in just a few minutes.
- Simplicity: It doesn’t require complex sample preparation or in-depth technical skills.
That said, while it is excellent for assessing concentration and quality in terms of contaminants, it provides limited information about the structural integrity of the RNA, which can be a drawback in certain applications.
Next-Generation Sequencing Approaches
Next-Generation Sequencing (NGS) provides a deeper look into RNA quality and integrity. In this approach, the actual sequencing process can give insights into RIN, as sequencing reads can reveal the presence of intact RNA transcripts versus degraded fragments. Recent advancements enable researchers to assess not only the quality but also to obtain a more comprehensive view of transcript abundance and diversity.
- Key Benefits:
- High Sensitivity: NGS can detect even minute quantities of RNA, providing a detailed picture.
- Multiplexing Capacity: It allows for the simultaneous assessment of multiple samples, thereby increasing efficiency.
Nonetheless, NGS can be more resource-intensive and time-consuming compared to traditional methods. Thus, the decision to use NGS for RIN assessment will depend on the specific research context and available funding.
The choice of methodology for RIN assessment impacts not only the accuracy of results but also the overall validity of RNA-Seq studies.
In concluding this section, it is essential to understand that each methodology has its place in research, with corresponding benefits and considerations. Awareness of these techniques enables researchers to optimize their RNA preparation workflows to achieve high-quality results, ultimately enriching the scientific community's insights into gene expression and functionality.
Impact of RIN on RNA-Seq Outcomes
In the realm of RNA sequencing (RNA-Seq), the RNA Integrity Number (RIN) plays a crucial role that cannot be overlooked. RIN serves not just as a marker of RNA quality but also significantly influences the results and accuracy of RNA-Seq experiments. High RIN values generally indicate intact and high-quality RNA, which is essential for producing reliable sequencing data. Conversely, low RIN scores can lead to fragmented RNA, increasing the likelihood of erroneous results that can skew biological interpretations.
Understanding the implications of RIN on RNA-Seq outcomes is significant for several reasons. First off, it helps researchers select the right samples for sequencing, thus minimizing the chances of obtaining erroneous or inconclusive data. Additionally, a clear comprehension of RIN’s role facilitates the optimization of analytical methods, ensuring that downstream applications are based on dependable biological data. This consideration becomes critical especially when working with precious clinical samples or rare subjects, wherein each data point carries considerable weight in the overall conclusions.
Correlation between RIN and Sequencing Quality
RIN and sequencing quality share a symbiotic relationship that profoundly dictates the success of RNA-Seq experiments. Simply put, a high RIN score is often synonymous with high-quality sequencing outputs. Studies showcase that RNA with a RIN score greater than seven tends to yield better sequencing results, exhibiting higher read counts and greater transcriptome coverage. On the other hand, samples with RIN scores below this threshold frequently suffer from low-quality sequencing reads, leading to incomplete or misleading datasets.
Several aspects contribute to this correlation:
- Fragmentation Level: High RIN correlates with less fragmentation of the RNA molecules, enhancing the fidelity of the sequencing process.
- Bias Reduction: Higher quality RNA diminishes biases in expression levels, allowing for a more accurate reflection of the transcriptome.
- Reduced PCR Errors: High-quality RNA minimizes the risk of polymerase errors that could result from amplification processes during library preparation.
"A thorough understanding of RIN helps avoid pitfalls that lead to misleading biological interpretations, cementing its importance in RNA-Seq outcomes."
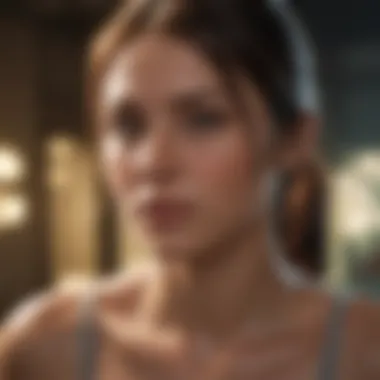
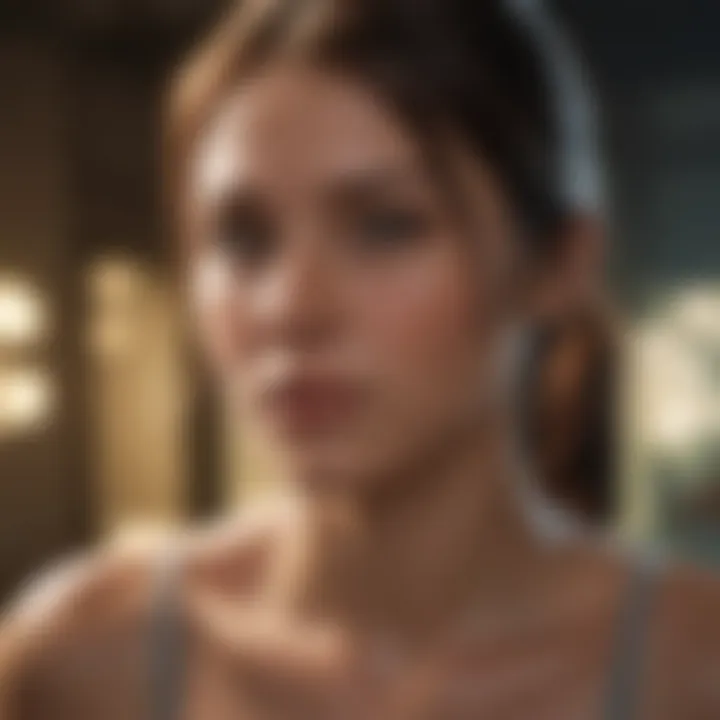
Influence of RIN on Biological Interpretation
The implications of RIN extend beyond the technical aspects of sequencing quality; it has profound effects on biological interpretation as well. Incorrect interpretations arising from low-quality RNA data can have significant ramifications in research fields ranging from genomics to clinical studies. When RNA is degraded, it can lead to skewed gene expression profiles, misleading researchers regarding the biological state of the samples.
Here are a few ways in which RIN affects biological interpretation:
- Gene Expression Analysis: Low RIN values may alter the expressions of genes, causing false positives or negatives. This discrepancy can lead to incorrect conclusions about disease mechanisms or biological pathways.
- Regulatory Mechanisms: Degraded RNA might misrepresent the regulatory aspects of the genome, leading to flawed assumptions about cellular responses or gene networks.
- Comparative Studies: In comparative RNA-Seq studies, inconsistencies in sample quality driven by RIN can complicate analyses, thereby impeding valid conclusions based on group differences or temporal changes.
Recognizing the strong connection between RIN and RNA-Seq results not only emphasizes the need for robust sample handling and preservation protocols but also serves as a reminder of the importance of thoroughly assessing RNA quality prior to analysis. In summary, the RIN is not just a number; it carries significant implications for the reliability and validity of RNA-Seq data, impacting the trajectory of molecular biology research.
Best Practices for RIN Optimization
Optimizing RNA Integrity Number (RIN) is crucial for ensuring high-quality RNA in RNA-Seq experiments. High RIN values indicate intact RNA, which is fundamental for reliable sequencing results. If the RNA samples are compromised, the entire experimental workflow can be flawed, leading to skewed data and misinterpretation of findings. Therefore, understanding and applying best practices for RIN optimization can profoundly influence the success of molecular biology studies.
Sample Collection and Storage
The journey of RNA integrity begins at sample collection. Proper technique and equipment are imperative. When collecting samples, it’s vital to use appropriate tools, such as RNase-free containers and pipettes. This helps prevent contamination, which can significantly affect the RIN.
- Speed is Key: Time is of the essence. The quicker the harvested sample is processed, the lower the chances of RNA degradation. For instance, using liquid nitrogen for snap-freezing samples immediately after collection is effective in maintaining integrity.
- Storage Conditions Matter: RNA is notoriously unstable. To ensure the longevity of RNA samples, they should be stored at -80°C. While some might suggest short-term storage at -20°C, it is not recommended due to potential degradation from freeze-thaw cycles.
- Use Compatible Buffers: During the initial stages of RNA extraction, using buffers that protect RNA, such as those containing guanidine thiocyanate, can enhance sample quality.
Avoiding Contamination and Degradation
Once samples are collected and stored, the next hurdle is maintaining that quality throughout handling and experimentation. Degradation of RNA can stem from various sources, including environmental factors, improper handling, and the presence of RNases.
- Maintain Sterility: Always use RNase-free reagents and consumables. Working in a clean environment minimizes the risk of introducing enzymes that degrade RNA. Regular cleaning of workspaces with RNase decontamination solutions is a good practice.
- Minimize Exposure to Heat and Light: RNA integrity can deteriorate with prolonged exposure to heat and UV light. It's advisable to keep samples on ice when possible and store them in opaque containers or wrapped in aluminum foil.
- Implement Best Handling Practices: Ensure that personal protective equipment, like gloves, is used when handling RNA samples. Changing gloves frequently helps to avoid contamination from the user's skin or clothing.
"RIN is more than a number; it reflects the story of your sample's quality, from collection to analysis. Treat it with care, and it will share its secrets well."
By adhering to these best practices, researchers can significantly improve the quality of their RNA samples, thereby enhancing the reliability of their RNA-Seq outcomes. Focusing on these aspects not only minimizes the risk of degradation and contamination but also bolsters the overall integrity of the research process.
Troubleshooting RIN-Related Issues
Troubleshooting RIN-related issues is a crucial aspect of ensuring high-quality RNA-Sequencing outcomes. If the RNA harvested from a sample is of poor integrity, it could greatly impact the reliability of the entire sequencing process. Understanding how to troubleshoot common problems could potentially save time, resources, and alleviate the stresses that arise from unexpected results. Evaluation of RIN should not only happen at the outset but during each stage of RNA handling. This includes sample collection, storage, and preparation.
Identifying Common Pitfalls
When working with RNA, several pitfalls may lead to suboptimal RIN values. Here are some common errors to watch out for:
- Poor Sample Collection: One of the first steps in RNA handling is often where things go awry. Using incorrect techniques or tools during the sampling process can lead to contamination or degradation.
- Inadequate Storage Conditions: RNA is notoriously fragile. Store samples in improper temperatures or in unsuitable conditions, and you can kiss your RIN good-bye.
- Extended Handling Times: Every minute counts when it comes to RNA integrity. Allowing samples to sit out—whether at room temperature or on ice—can lead to RNA degradation.
- Use of Incompatible Reagents: Some chemicals used during RNA extraction can end up damaging RNA if they're not properly formulated. Knowing what plays well with others is essential.
Each of these pitfalls contributes directly to a potential decrease in RIN, making it critical to pay attention at each stage.
Solutions and Recommendations
To effectively address the issues outlined above, consider the following solutions:
- Train Personnel Thoroughly: Make sure everyone involved in RNA handling knows the best practices for sample collection and processing. A little education goes a long way in minimizing human error.
- Implement Strict Storage Protocols: Devise a foolproof protocol for sample storage that includes specific temperature controls and guidelines on how long samples can be left at room temperature.
- Plan Efficient Workflows: Create an organized workflow that minimizes the time RNA spends outside of controlled conditions. Always have your reagents ready and follow a checklist.
- Invest in Quality Control Measures: Regularly assess RIN values post-collection and during analysis to catch any issues early. Using reliable methods of stabilization can also help retain RNA quality during processing.
Effective troubleshooting is not just about fixing problems; it's about preventing them from happening in the first place.
By addressing pitfalls proactively and adopting recommended practices, one can significantly improve the likelihood of obtaining samples with high RIN values, positively impacting downstream RNA-Seq results.
RIN Variation Across Different Sample Types
RIN, or RNA Integrity Number, is a crucial metric in evaluating RNA quality across various sample types. The significance of RIN variation becomes clear when we consider that the biological source of RNA often plays a pivotal role in its integrity. The variability in RIN values can inform researchers regarding how effectively RNA samples can be utilized in downstream applications, particularly RNA sequencing. Understanding how RIN behaves in different biological contexts helps research professionals optimize their experiments for accuracy.
RIN in Plant vs. Animal Samples
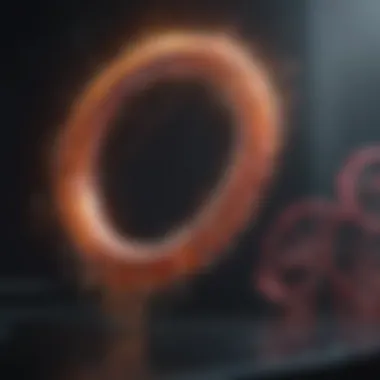
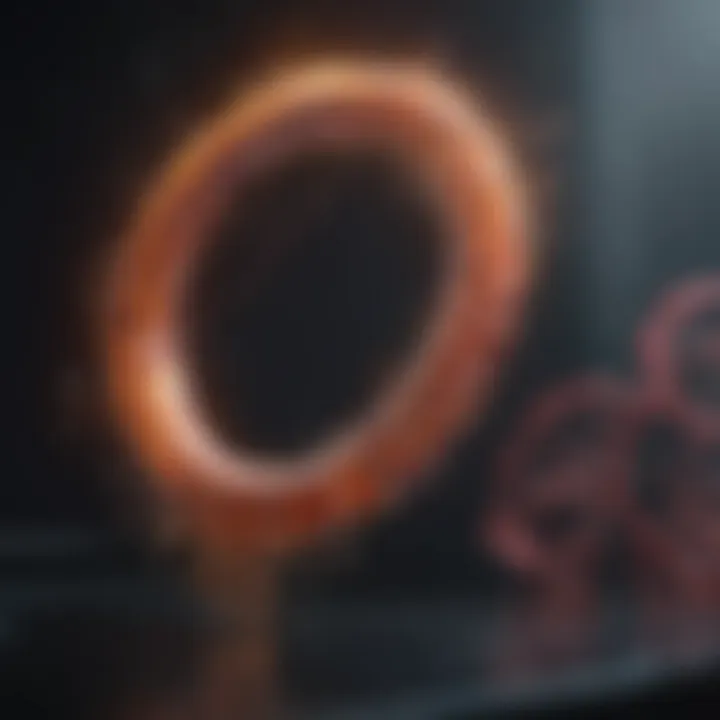
When it comes to assessing RIN in plant and animal samples, researchers encounter a notable difference. Plant RNA tends to exhibit a higher degree of variability due to the presence of complex cell walls and other structural barriers, which can hinder RNA extraction processes. In contrast, animal RNA usually presents a more straightforward extraction landscape due to its cellular architecture. As a result, RIN values of plant samples can sometimes be more unpredictable, highlighting the importance of method standardization and optimization.
- Key Factors Affecting RIN in Plants:
- Cell wall structure
- RNA extraction methods
- Degradation due to environmental stressors
Animal samples, including tissues, often yield more consistent RIN values. Still, it’s worth noting that factors such as sample storage time and temperature during collection may lead to fluctuations. This brings into focus the necessity for tailored protocols depending on the sample's origin.
Variability in Clinical Samples
Clinical samples exhibit their own set of challenges when it comes to RIN variability. For example, RNA harvested from blood or peripheral blood mononuclear cells can display lower RIN values compared to biopsies of solid tumors. This discrepancy is often attributed to the innate variability of RNA stability in different biological environments, as well as the handling parameters before and during sample processing.
When working with clinical samples, several considerations need attention to ensure high-quality RIN values:
- Sample Collection: Must be executed with precision to avoid contamination and degradation.
- Storage Conditions: Optimal temperature and time must be adhered to, as improper management can severely affect RNA quality.
- Patient Factors: Individual differences such as age, health status, and even medication can influence RNA integrity, making clinical studies particularly complex.
Effective quality control mechanisms are essential in clinical research to maintain high RIN levels across diverse sample types.
In summary, both plant and animal samples, alongside clinical samples, demonstrate unique variability in RIN values. This variation necessitates an adaptive approach to RNA extraction and quality assessment, ensuring that researchers can derive meaningful insights from their RNA-Seq studies. Recognizing these differences allows for better planning and improved methodologies tailored to specific types of biological material.
RIN and Different Experimental Conditions
The RNA Integrity Number (RIN) is not just a static measure; it dynamically interacts with various experimental conditions. As researchers delve into RNA-Seq, understanding how these conditions influence RIN becomes essential. Temperature, time, and chemical treatments can all affect RNA quality, thus impacting the overall integrity of RIN assessments. By comprehending these intricate relationships, one can better prepare samples, optimize protocols, and ensure the reliability of RNA sequencing results.
Impact of Temperature and Time
Temperature and time are two critical factors that can drastically alter RNA quality. When you think about how RNA degrades, it’s often due to the activities of ribonucleases, enzymes that break down RNA molecules. These enzymes are sensitive to environmental conditions, primarily temperature.
- High Temperatures: Exposing RNA to elevated temperatures, for instance, can accelerate the degradation process. Experiences in the lab have shown that a mere increase in the room temperature, particularly above 25°C, often leads to marked declines in RIN scores. If treated casually, RNA samples might slide into poor RNA integrity territory.
- Low Temperatures: On the flip side, keeping RNA samples on ice or at ultra-cold temperatures can dramatically preserve their integrity. It's paramount to remember that time spent at less-than-ideal temperatures counts. If RNA is allowed to stand at ambient conditions for too long—even for just a few hours—it might not yield the high RIN results one hopes for in downstream applications.
"Maintaining RNA samples at consistent low temperatures can be the difference between success and failure in sequencing endeavors."
Effects of Chemical Treatments
Chemical treatments during RNA extraction and purification can yield both positive and negative effects on RIN. While these treatments can enhance RNA quality by removing contaminants, improper use can lead to complications.
- Common Reagents: Many labs use phenol-chloroform extraction to clean up RNA samples. This method can be effective, but if not executed correctly, the chemicals can cause hydrolysis, thus damaging RNA integrity. Are there residual chemicals left behind? If so, they could potentially impact future assays.
- pH Levels: The pH of solutions used in the extraction process also plays a significant role. RNA is most stable in a slightly alkaline environment but can become unstable in highly acidic conditions. This is where one needs to tread carefully.
- Additives: Some researchers introduce stabilizing agents like RNAlater or other commercial buffers to improve RIN scores. However, the effectiveness of these solutions can vary, and one must validate their usage within specific experimental frameworks.
Understanding these chemical influences on RNA integrity could mean the difference between inconclusive results and groundbreaking discoveries. Emerging technologies are focusing on improving chemical treatments, paving the way for more reliable RIN assessments.
In sum, whether through temperature fluctuations or the interaction of chemical reagents, the experimental conditions surrounding the handling of RNA centrally impact RIN values. Being cognizant of these influences gives scientists the tools to maximize the quality of their RNA samples, ultimately enhancing the reliability and richness of data retrieved from RNA-Seq studies.
Future Directions in RIN Studies
The future of RNA Integrity Number (RIN) research is a promising horizon, where emerging methodologies are set to enhance our understanding of RNA quality. The insights gained from RIN studies are not only pivotal for improving RNA-Seq outcomes but also have implications for various biomedical fields. Considering the rapid advancements in RNA analysis techniques, it is essential to explore these new avenues with a critical eye toward their practical benefits and applicability in real-world contexts.
Emerging Techniques for RNA Assessment
As technology progresses, new methods for RNA assessment are gaining traction. For instance, quantitative PCR (qPCR) coupled with RIN analysis allows researchers to not just measure RNA integrity, but also to assess its functional viability. This is increasingly important as scientists strive to differentiate between merely intact RNA and RNA that can effectively participate in biological processes.
Another intriguing approach involves the application of microfluidic devices that can analyze RNA samples at a surprisingly small scale. These devices offer rapid processing times, which can be invaluable in high-throughput settings. Microfluidics likely improves accuracy by reducing sample handling errors, making them beneficial in both clinical and research laboratories.
"New techniques not only refine our understanding of RIN but also expand our toolkit for molecular analysis."
Next-Generation Sequencing (NGS) continues to evolve. Techniques such as RNA-Seq now incorporate RIN assessment techniques during library preparation stages. This integration provides real-time feedback on RNA integrity, allowing for immediate adjustments before running the sequences. This way, researchers are reducing the risk of poor-quality results, which often stem from compromised RNA.
Integrating RIN into Multi-Omics Approaches
The integration of RIN into multi-omics frameworks is another compelling direction for future studies. As researchers begin to intertwine transcriptomics, proteomics, and metabolomics, establishing a standardized RNA integrity benchmark becomes crucial. By doing so, scientists can facilitate deeper biological insights that are reproducible across different omics levels.
For example, when examining the correlation of various biomarkers in diseases such as cancer, including RIN as a variable ensures that RNA quality does not skew the interpretation of results. This layer of integrity assessment is vital, considering the complexities involved in omics data integration.
Also, incorporating RIN into the design of experiments will enhance data interpretation across studies. This can lead to the establishment of a comprehensive quality control protocol that would enhance contributions in personalized medicine, where tailoring treatment options according to individual genetic profiles is paramount.
As multi-omics approaches gain traction, the relationship between RIN and other molecular parameters could provide a broader understanding of cellular functions and their responses to therapeutic interventions. Researchers should consider this integration as part of their experimental design to pave the way for impactful scientific discoveries.