Understanding mRNA Production: Mechanisms and Implications
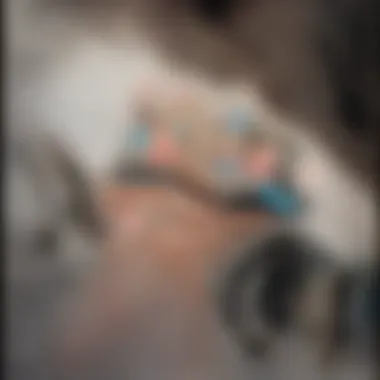
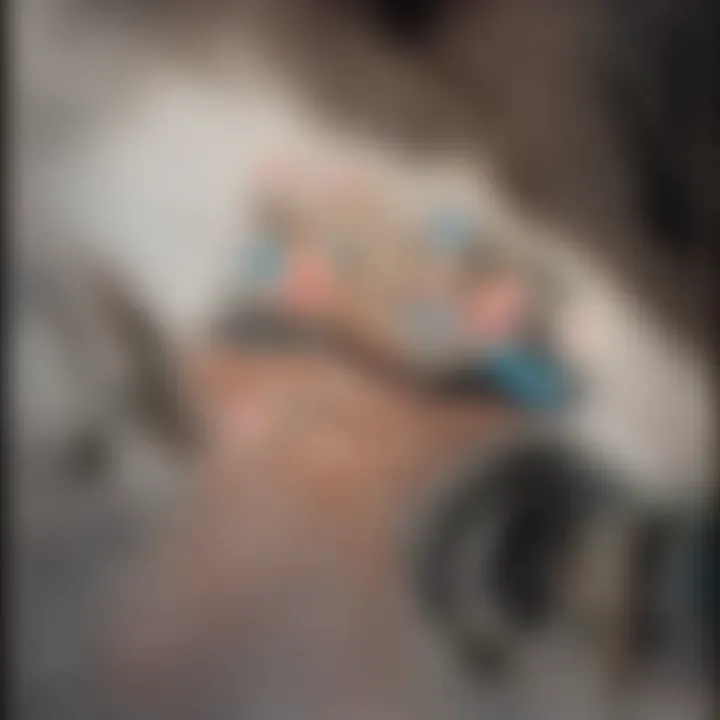
Intro
The production of messenger RNA (mRNA) is a critical biological process that orchestrates the flow of genetic information from DNA to protein synthesis. Understanding mRNA production provides insights into the regulation of gene expression, which is essential in diverse fields like biotechnology and medicine. This article examines various aspects of mRNA production, aiming to elucidate its intricate mechanisms and broader implications for cellular functions.
Background and Context
Overview of the Research Topic
The process of mRNA production involves several key steps, primarily transcription, where an RNA copy of the gene is made from the DNA template. The main enzyme responsible for this process is RNA polymerase, which unwinds the DNA and synthesizes the mRNA strand. This mechanism is driven by a series of regulatory elements including promoters, enhancers, and transcription factors, which modulate the rate and specificity of transcription.
Historical Significance
Historically, the study of mRNA production has evolved significantly. Initially, it was a mystery how genetic information encoded in DNA was translated into functional proteins. The discovery of the role of mRNA by scientists like François Jacob and Jacques Monod paved the way for a better understanding of gene regulation. Their work in the 1960s highlighted the importance of mRNA as an intermediary in protein synthesis, leading to the realization that mRNA is central to cellular function. Over the decades, advancements in molecular biology techniques have facilitated deeper insights into mRNA biogenesis and its regulation.
Key Findings and Discussion
Major Results of the Study
Research on mRNA production has yielded several notable findings. For instance, the identification of various transcription factors has shown that gene expression can be finely tuned by specific protein-DNA interactions. Additionally, studies have revealed the significance of alternative splicing in mRNA maturation, allowing a single gene to produce multiple protein variants, thus increasing the complexity and functionality of the proteome.
Detailed Analysis of Findings
The interplay of different cellular components during mRNA production is complex. Here are key elements involved in the transcription process:
- DNA Template: The gene of interest serves as the template for RNA synthesis.
- RNA Polymerases: These enzymes are crucial for catalyzing the transcription of DNA into mRNA. In eukaryotes, three types exist: RNA polymerase I, II, and III, with RNA polymerase II responsible for mRNA synthesis.
- Transcription Factors: These proteins bind to specific DNA sequences to regulate transcription levels. They can act as activators or repressors, influencing how much mRNA is produced.
Understanding the dynamics of mRNA production is essential for biomedical advancements, especially in developing gene therapies and mRNA vaccines.
The End
Prologue to mRNA
The topic of mRNA production plays a crucial role in modern biology, linking gene expression to the synthesis of proteins that are essential for various cellular functions. As a fundamental molecule in the flow of genetic information, understanding mRNA provides insights into various biological processes, including how cells respond to stimuli and regulate their activity. This knowledge is not only academically important but also has practical applications in biotechnology and medicine, which can transform how we approach treatment and therapeutics.
Defining mRNA
Messenger RNA, or mRNA, is a type of nucleic acid that serves as the intermediary between DNA and proteins. It carries the genetic code from the DNA in the nucleus to the ribosomes in the cytoplasm, where proteins are synthesized. Each mRNA molecule is transcribed from a specific gene, containing codons that specify amino acids, the building blocks of proteins. The mRNA is critical for translating genetic information into functional products.
Historical Context
The study of mRNA began in the mid-20th century, following the discovery of DNA's structure by James Watson and Francis Crick. The term "messenger RNA" was first introduced in the early 1960s when researchers recognized that a specific RNA molecule carried the genetic instructions from the DNA. Understanding this relationship paved the way for further research on cellular processes and revolutionized molecular biology. Over the decades, scientists have unraveled numerous complexities related to mRNA, leading to technologies like CRISPR-Cas9 and mRNA vaccines, which have become particularly relevant in recent times.
Importance of mRNA in Cells
mRNA is a critical component of cellular machinery. Its primary function is to convey genetic information from the DNA to the ribosome, where it directs the synthesis of proteins. The precise regulation of mRNA levels is fundamental for proper cellular functioning, as it ensures that proteins are produced at the right time and in the right amounts. Any malfunction in mRNA processing or translation can lead to cellular dysfunction, affecting everything from metabolism to cell growth. Moreover, mRNA plays a significant role in responding to environmental cues, enabling cells to adapt to changing conditions quickly.
"The dynamic nature of mRNA production directly influences cellular response capabilities, underscoring its importance in cellular biology."
In summary, understanding mRNA involves appreciating its multifaceted roles in cellular processes. From its definition to its historical significance and importance within cells, mRNA is essential for translating genetic blueprints into functional proteins that maintain life.
Transcription: The Process of mRNA Production
Transcription is a fundamental biological process that serves as the initial step in mRNA production. This process is crucial because it converts the genetic information encoded in DNA into a complementary RNA strand, which ultimately leads to protein synthesis. Understanding transcription provides insight into how genes are expressed and how cells respond to various stimuli. The regulation of this process is vital for maintaining homeostasis and ensuring that cellular functions are executed correctly. In the context of mRNA production, transcription serves not just as a mechanism for copying the genetic blueprint, but also as a critical point of regulation that affects gene expression.
Role of DNA in Transcription
DNA plays a central role in the transcription process. The double helix structure of DNA contains the genetic instructions necessary for the development and functioning of living organisms. During transcription, a specific segment of DNA is accessed. The unwinding of DNA takes place at the location of the gene to be transcribed. This unwinding exposes the sequence of nucleotide bases that will guide the synthesis of mRNA. It is important to note that only one strand of the DNA serves as a template during this process. This template strand is known as the antisense strand while the other strand is the coding strand. The choice of the template strand is critical and can influence the resulting RNA product.
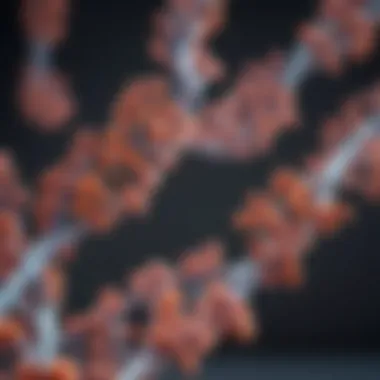
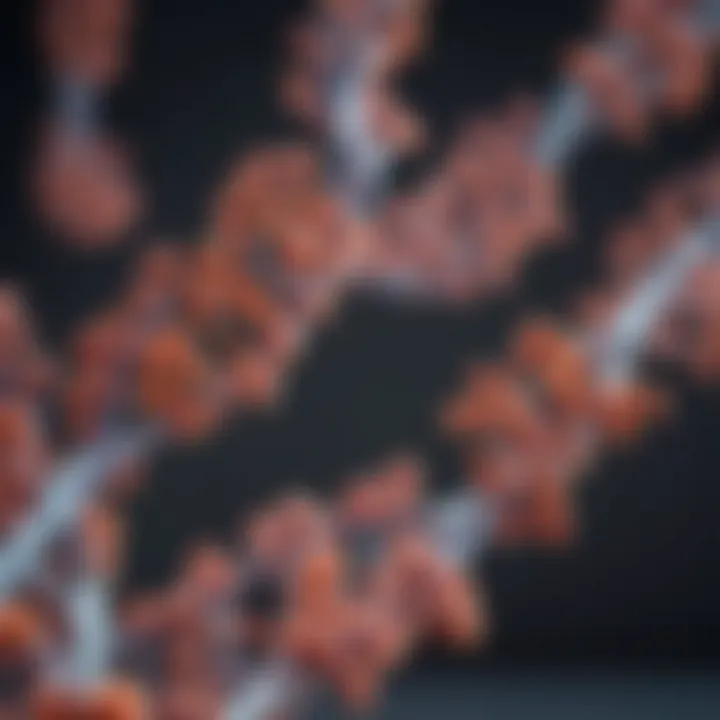
Initiation of Transcription
The initiation phase involves several key steps necessary for transcription to commence. The first step is the binding of transcription factors to specific regions of the DNA called promoters. These transcription factors facilitate the recruitment of RNA polymerase, the enzyme that synthesizes RNA. Once RNA polymerase is properly positioned at the promoter, the DNA unwinds further, creating an open complex. This complex is essential as it allows RNA polymerase to begin synthesizing mRNA by pairing complementary RNA nucleotides with the template DNA strand. This phase is tightly regulated as it ensures that transcription occurs only under suitable conditions, in appropriate cell types, and at the right stages of development.
Elongation Phase
Once initiation is complete, the elongation phase begins. During elongation, RNA polymerase moves along the DNA strand, adding nucleotides to the growing mRNA molecule. Each nucleotide is added in a sequence complementary to the template DNA strand, following base-pairing rules. As the enzyme progresses, it continues to unwind the DNA ahead of it while rewinding the DNA behind, maintaining the overall integrity of the DNA molecule. The speed and accuracy of RNA polymerase during this phase can influence the efficiency of mRNA production. Errors during elongation can lead to mutations in RNA, which can have downstream effects on protein synthesis and cellular function.
Termination of Transcription
Termination marks the end of the transcription process. This phase is triggered by specific signals within the DNA sequence, often corresponding to terminator regions. When RNA polymerase encounters these signals, it releases the newly synthesized mRNA strand and detaches from the DNA. The termination process is as crucial as initiation. It ensures that transcription stops after the complete gene has been copied. After termination, further processing of the mRNA occurs, preparing it for translation into proteins. This includes modifications like the addition of a 5' cap and a poly-A tail, which are vital for mRNA stability and translation efficiency.
"Transcription is not merely about copying DNA; it is a complex orchestration involving various factors that ensure accuracy and regulation of gene expression."
In summary, transcription is a pivotal process in mRNA production, characterized by several distinct phases: initiation, elongation, and termination. Each phase involves specific mechanisms and components that facilitate the correct expression of genes. The fidelity and regulation of transcription have profound implications for cellular function and biological responses.
Key Components in mRNA Production
Key components of mRNA production are essential for understanding how genes are transcribed into messenger RNA. The production of mRNA does not occur in isolation. It relies on a complex interplay between multiple factors that ensure proper transcription, regulation, and processing. Each of these components plays a critical role in determining the efficiency and accuracy of mRNA synthesis. Understanding these key elements is necessary for students, researchers, and professionals who aim to explore the nuances of genetic expression and its applications in biotechnology and medicine.
RNA Polymerases
RNA polymerases are the primary enzymes that catalyze the synthesis of RNA from a DNA template during transcription. In eukaryotic cells, there are three main types of RNA polymerases: RNA Polymerase I, II, and III. Each type is responsible for transcribing specific classes of genes.
- RNA Polymerase I primarily synthesizes ribosomal RNA (rRNA), except for 5S rRNA.
- RNA Polymerase II transcribes protein-coding genes into mRNA, making it the most critical for mRNA production.
- RNA Polymerase III is responsible for the transcription of small RNAs, including transfer RNA (tRNA) and 5S rRNA.
The importance of RNA polymerases cannot be overstated. They initiate transcription by recognizing promoter regions of the DNA and unwinding the double helix. Following this, they synthesize RNA strands by adding ribonucleotides complementary to the DNA template. The enzyme’s fidelity in selecting the correct nucleotide influences the quality of mRNA produced. Misincorporation of nucleotides can lead to non-functional proteins, which may affect cellular functions.
Transcription Factors
Transcription factors are proteins that assist in the initiation of transcription by binding to specific DNA sequences. Their interaction with RNA polymerases is critical for gene expression regulation. Transcription factors can be categorized into two major types:
- General Transcription Factors (GTFs): These factors are required for the transcription of all protein-coding genes. They help RNA Polymerase II to recognize promoter regions and start transcription.
- Specific Transcription Factors: These factors are involved in the regulation of specific genes by enhancing or repressing the transcription process. They respond to developmental cues, environmental signals, and cellular conditions.
The dynamic nature of transcription factors allows cells to adapt quickly to changes. For example, in response to stress, specific transcription factors can activate genes that help in overcoming the challenge. This specificity makes transcription factors vital in many biological processes, including differentiation and response to stimuli.
Promoters and Enhancers
Promoters and enhancers are DNA sequences that play crucial roles in the regulation of transcription. A promoter is a region located at the beginning of a gene and serves as the binding site for RNA polymerase and transcription factors. Promoters determine the frequency of transcription initiation and are often categorized into two types:
- Core Promoter: Contains essential elements required for basic transcription.
- Proximal Promoter Elements: These are located close to the core promoter and help fine-tune transcription levels.
Enhancers are distant regulatory elements that can significantly increase transcription levels. They can be located upstream or downstream of the gene they regulate. Once bound by specific transcription factors, enhancers facilitate the assembly of the transcription machinery at the promoter.
The interplay between promoters and enhancers exemplifies the complexity of gene regulation. Their combined efforts can greatly amplify gene expression in response to various signals.
Silencers and Insulators
Silencers and insulators also contribute to the regulation of mRNA production, but in different ways compared to enhancers. Silencers are sequences that inhibit transcription when bound by repressor proteins. They prevent RNA polymerase from transcribing genes, essentially silencing them. This regulation is essential for processes like developmental timing and cell differentiation.
Insulators, on the other hand, act as boundaries that prevent gene activation signals from spreading to adjacent genes. They can protect genes from the effects of nearby enhancers or silencers. This function is crucial in maintaining distinct gene expression patterns within the genome.
In summary, understanding the roles of these key components is critical for grasping the intricacies of mRNA production. Together, these elements orchestrate a finely-tuned transcription process that underpins gene expression.
Regulation of mRNA Production
The regulation of mRNA production is an essential aspect of gene expression. Understanding how this regulation occurs provides insights into cellular processes and responses to various stimuli. The mechanisms that control mRNA production influence how much protein a cell produces and at what times. This regulation is vital for maintaining homeostasis in biological systems and can have far-reaching implications in health and disease.
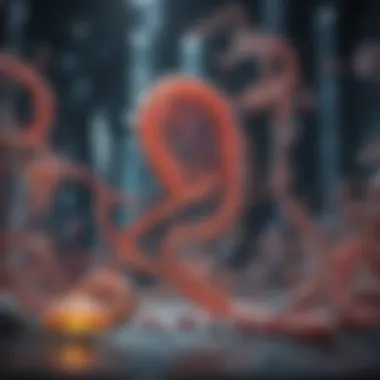
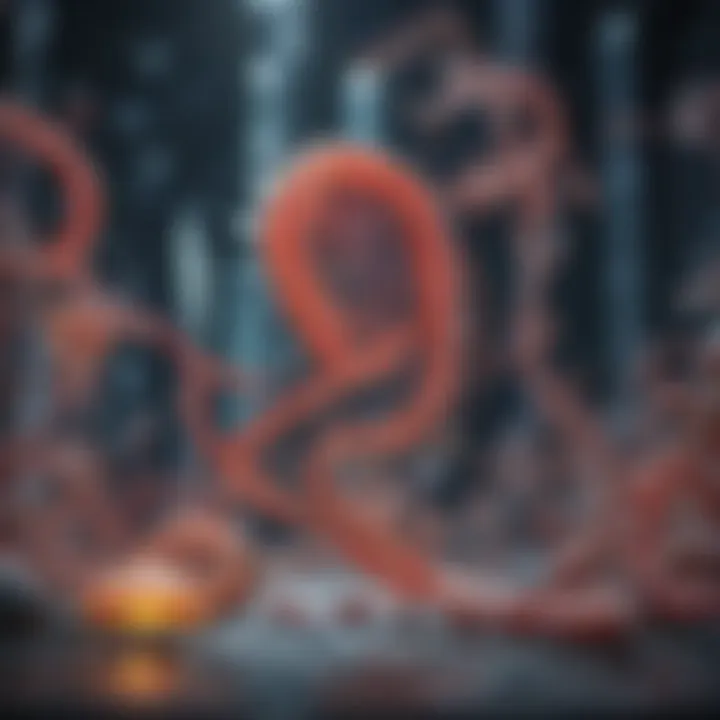
Gene Expression Regulation
Gene expression regulation involves multiple layers of control that dictate when and how genes are expressed as mRNA. Several factors contribute to this regulation. The role of transcription factors cannot be overstated. They act as activators or repressors by binding to specific sequences in the DNA. Their presence can significantly influence mRNA synthesis by promoting or hindering the recruitment of RNA polymerases to the promoter regions.
Moreover, epigenetic modifications like DNA methylation and histone modification are critical. These changes can alter the accessibility of DNA, thus affecting the transcriptional machinery's ability to initiate mRNA production. Environmental factors such as stress, nutrient availability, and signaling molecules also modulate gene expression. Hence, regulation is a delicate balance between internal and external signals.
Post-Transcriptional Modifications
Post-transcriptional modifications are processes that occur after the primary mRNA transcript is synthesized. These modifications include capping, polyadenylation, and splicing. Each of these steps plays a crucial role in the stability, localization, and translation efficiency of the mRNA.
- Capping: The addition of a 5’ cap protects mRNA from degradation and facilitates ribosome binding during translation.
- Polyadenylation: This process involves adding a poly(A) tail to the 3’ end of the mRNA, which also enhances stability and aids in transport from the nucleus to the cytoplasm.
- Splicing: This involves the removal of introns and the joining of exons to form a mature mRNA.
These modifications not only ensure mRNA stability but also influence the half-life of the mRNA in the cytoplasm, ultimately affecting protein synthesis.
Alternative Splicing
Alternative splicing is a key process that allows a single gene to produce multiple mRNA variants. This mechanism can lead to the synthesis of different proteins, thus increasing the functional diversity of the proteome without the need for additional genes.
During splicing, specific sequences known as splice sites guide the removal of certain exons or introns, depending on the cell's needs. This process can generate different isoforms of proteins that have diverse functions or regulatory roles. The choice of splice sites can be influenced by cis-acting elements located on the pre-mRNA as well as trans-acting factors.
"Alternative splicing is a sophisticated way cells adapt to changes in their environment by producing different protein variants from the same genetic sequence."
mRNA and Protein Synthesis
The relationship between mRNA and protein synthesis is pivotal in cellular biology. Messenger RNA, or mRNA, acts as a crucial intermediary between the genetic information encoded in DNA and the synthesis of proteins. This process is fundamental because proteins play essential roles in virtually all biological processes. Thus, understanding how mRNA facilitates protein synthesis is a key aspect of molecular biology.
Translation: From mRNA to Protein
Translation is the process where ribosomes decode mRNA to synthesize proteins. This begins when mRNA, having been transcribed from DNA, binds to a ribosome. This is a sophisticated machinery composed of ribosomal RNA (rRNA) and proteins. The ribosome then reads the mRNA sequence in groups of three nucleotides, called codons. Each codon corresponds to a specific amino acid.
During translation, the ribosome moves along the mRNA strand, facilitating the binding of transfer RNA (tRNA) molecules. Each tRNA carries an amino acid and has an anticodon that pairs with a complementary codon on the mRNA. This interaction ensures the correct amino acid sequence is assembled, creating a polypeptide chain that will fold into a functional protein.
Role of Ribosomes
Ribosomes are often referred to as the sites of protein synthesis. They serve as the machinery where mRNA is translated into a protein. Ribosomes are composed of two subunits, the large and small subunit. These subunits come together during translation and separate afterward to initiate another round of protein synthesis.
The large subunit contains binding sites for both tRNA and mRNA. It catalyzes the formation of peptide bonds between adjacent amino acids, elongating the polypeptide chain. The small subunit is primarily responsible for decoding the mRNA sequence. The efficiency of ribosomes is critical for cellular function, and any disruption in their activity can lead to significant biological consequences.
tRNA: The Adapter Molecule
Transfer RNA, or tRNA, plays an essential role as the adapter molecule in protein synthesis. Each tRNA molecule has a specific structure that enables it to carry a single amino acid. The crucial feature of tRNA is its anticodon, which is a sequence of three nucleotides complementary to a specific codon on the mRNA.
Once the tRNA molecule recognizes and binds to the corresponding codon on the mRNA, it brings its amino acid to the growing polypeptide chain. This specific pairing is vital to maintaining the accuracy of protein synthesis. The availability and functionality of tRNA are also key factors influencing the overall efficiency of the translation process.
"Without mRNA, the entire process of protein synthesis would cease to exist, disrupting countless biological functions."
Factors Influencing mRNA Production
The production of mRNA is not merely a straightforward process; it is significantly influenced by various external and internal factors. Understanding these influences is crucial for comprehending how genes are expressed within cells. Factors such as environmental conditions, cellular stress responses, and hormonal influences play a vital role in determining the levels and functionality of mRNA. By examining these elements, researchers gain deeper insights into gene regulation and the overall cellular response to different stimuli.
Environmental Impacts
Environmental factors can profoundly affect mRNA production. Changes in temperature, light, and nutrient availability can lead to significant variations in gene expression. For instance, exposure to UV radiation can induce stress responses in cells, which may alter the transcription rate of specific genes. Furthermore, in plants, light conditions are crucial for regulating the expression of photosynthesis-related genes.
"Environmental influences can trigger profound shifts in mRNA levels, potentially altering cellular functions and overall health."
The capability of organisms to adapt to environmental changes often hinges on their ability to modulate mRNA synthesis. This adaptability is essential for survival and function across various ecosystems.
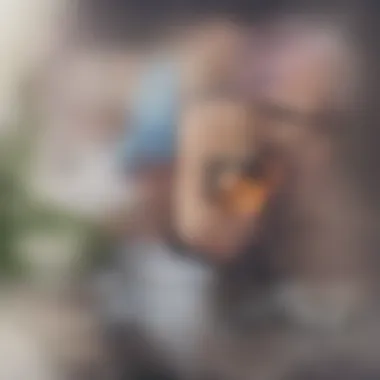
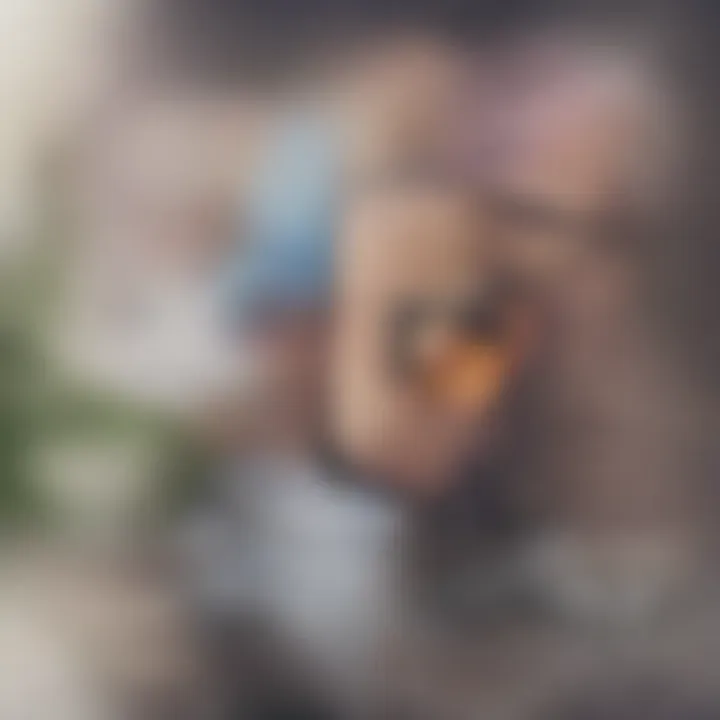
Cellular Stress Responses
Cells respond to stress, such as oxidative stress or nutrient deprivation, in complex ways that involve mRNA production. Under stress conditions, specific signaling pathways are activated, which can lead to the up-regulation or down-regulation of mRNA levels. For example, heat shock proteins are produced in response to elevated temperatures, and their encoding mRNAs are synthesized at increased rates.
The relationship between stress and mRNA is significant in therapeutic contexts, especially regarding diseases like cancer. Understanding how stress responses influence mRNA production can provide insights into potential treatment strategies, as altered expression may correlate with disease progression.
Hormones and mRNA Expression
Hormones are key regulators of mRNA expression, influencing various physiological processes. For example, cortisol can enhance the transcription of specific genes involved in stress responses. Similarly, insulin plays a crucial role in regulating mRNA levels related to metabolism.
In a broader context, hormonal signaling can induce cascades that lead to the activation or repression of gene expression via specific transcription factors. Consequently, understanding hormonal influences is vital not only for basic biological research but also for medical applications, such as developing hormone-based therapies.
Recognizing the interplay between hormones and mRNA production provides insights into the intricate regulation of cellular processes, offering avenues for future research and applications in health and disease.
Current Research and Applications
The field of mRNA research is rapidly advancing, revealing significant implications for various sectors including biotechnology and medicine. This progress arises from a deeper understanding of the mechanisms of mRNA production, as well as the evolving techniques used to manipulate and apply mRNA for practical purposes. The ability to engineer mRNA offers extensive possibilities that extend beyond basic cellular biology.
mRNA in Biotechnology
mRNA technology has become a cornerstone in modern biotechnology. Researchers are exploring its applications in vaccine development, particularly highlighted by the recent surge in messenger RNA vaccines for infectious diseases. These vaccines, such as those developed by Pfizer-BioNTech and Moderna, demonstrate how mRNA can be used to instruct cells to produce antigens, thereby eliciting an immune response.
Moreover, mRNA platforms are versatile. They not only aim at combating viruses but also hold promise in cancer treatment. By encoding tumor-associated antigens, personalized mRNA vaccines can be tailored to individual patients. This approach leverages the unique characteristics of each tumor, aiming to improve patient outcomes and reduce side effects associated with traditional therapies.
Therapeutic Uses of mRNA
The therapeutic potential of mRNA extends into diverse areas beyond vaccines. Current research focuses on utilizing mRNA to produce proteins that may be deficient in certain diseases. For example, in genetic disorders where proteins are missing or dysfunctional, administering mRNA that encodes the correct version of these proteins could provide a treatment avenue.
In addition to genetic disorders, mRNA is being studied as a treatment strategy for cardiovascular diseases. By delivering mRNA that encodes growth factors, researchers aim to promote regeneration in heart tissue after injury. This intersection of mRNA therapeutic applications represents a significant departure from traditional methods, paving the way for novel treatment paradigms.
Future Trends in mRNA Research
Looking ahead, mRNA research is poised for significant evolution. As more is learned about its properties, researchers aim to enhance its stability and efficacy. Modifications to the structure of mRNA may improve its delivery to target tissues, making treatments more effective.
Furthermore, the exploration of mRNA’s role in regulating gene expression is gaining momentum. The next phase may involve merging mRNA technology with CRISPR gene editing, resulting in innovative strategies for tackling complex diseases.
Research collaborations across institutions and industries are expected to accelerate discoveries and application breakthroughs. The burgeoning interest and investment in mRNA technology indicate a promising future, one where mRNA will likely play a pivotal role in shaping therapeutic landscapes.
"The ability to harness mRNA for tailored therapeutics signals a new age in medicine, with the potential to address previously unmet medical needs effectively."
In summary, ongoing developments in mRNA research encapsulate its profound influence in biotechnology and medicine. As this field unfolds, it promises enhancements in health, personalized medicine, and innovative treatment methodologies.
Finale
The conclusion of this article serves to encapsulate the complex and pivotal role mRNA plays within biological systems. As we explored the intricacies of mRNA production, we have observed how crucial this molecule is, not just in the realm of cellular processes but also in broader applications such as biotechnology and medicine. The informed understanding developed throughout the sections highlights several specific elements of significance.
First and foremost, the importance of mRNA as an intermediary between DNA and protein synthesis cannot be overstated. This central role allows mRNA to act as a messenger, conveying critical information from the genetic blueprint stored in DNA to the ribosomes where proteins are synthesized.
Moreover, the regulatory mechanisms governing mRNA production have profound implications. They determine the timing, location, and quantity of protein synthesis, thereby influencing cellular function and responsiveness to environmental cues. This regulatory capacity points to a layer of sophistication in cellular operations, enabling adaptation and fine-tuning of responses to internal and external stimuli.
The insights gained from understanding mRNA production extend beyond basic biology. Everyday applications in medical therapies, particularly those that utilize mRNA technology for vaccination and treatment strategies, underscore its relevance in contemporary science.
In summary, the conclusion chapter emphasizes that as mRNA continues to be at the forefront of biotechnological innovations, further investigation into its production and regulation could lead to significant advancements in medicine and research.
Summary of Key Points
- mRNA acts as a messenger, bridging the gap between DNA and protein synthesis.
- The production and regulation of mRNA are crucial for cellular function and adaptability.
- Current applications in mRNA technology impact fields such as medicine and vaccine development.
- Future research in mRNA has the potential to unlock further therapeutic possibilities.
Implications for Future Research
The exploration of mRNA production opens avenues for extensive future research. There are various implications emerging from this field.
- Therapeutic Developments: With advancements in mRNA technology, there exists a potential to revolutionize vaccines and treatments for various diseases. Understanding its production could lead to more efficient designs of mRNA therapies.
- Regulatory Pathways: Investigating the complex regulatory mechanisms governing mRNA could provide insights into gene expression profiles. Such knowledge can inform strategies for manipulating gene expression in clinical settings.
- Environmental Influences: Research into how environmental factors impact mRNA production may contribute to understanding diseases related to cellular response mechanisms.
- Alternative Applications: The ongoing fascination with mRNA derivatives might promote diversification of applications in fields such as genetic engineering and synthetic biology.