Understanding Histone Methylase: Mechanisms in Gene Regulation
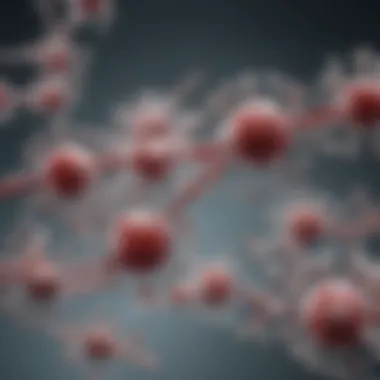
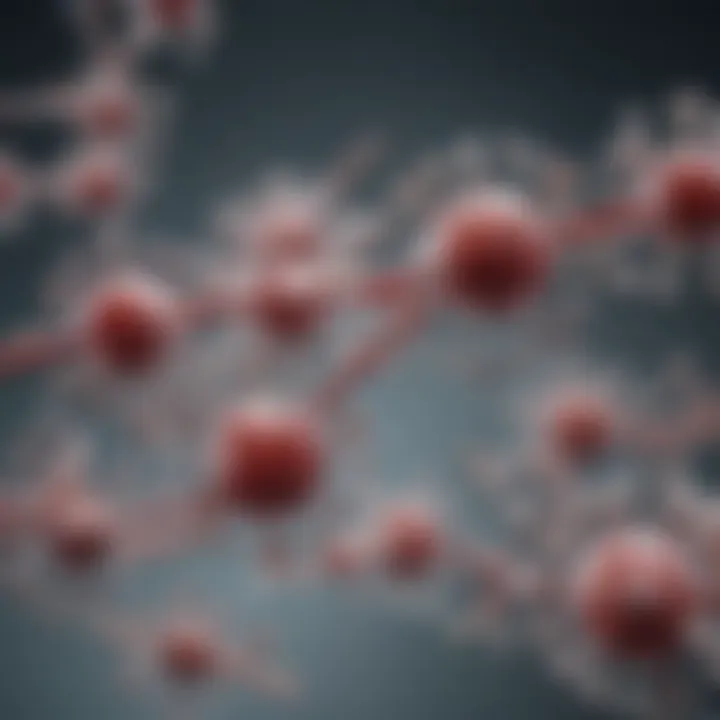
Background and Context
Histone methylases play a crucial role in the regulation of gene expression. These enzymes add methyl groups to specific lysine and arginine residues on histone proteins. This modification can profoundly alter the way DNA is packaged within the cell nucleus, impacting transcriptional activity. The complexity of gene regulation involves various mechanisms, with histone methylation being a key aspect.
Overview of the research topic
The advent of epigenetics has led to an increased focus on histone modifications, including methylation. Histone methylases influence chromatin structure, which is essential for cellular processes like DNA replication, repair, and gene transcription. Dysregulation of these enzymes is linked to numerous diseases, including cancer. Researchers are now investigating the therapeutic potential of targeting histone methylases to correct abnormal gene expression.
Historical significance
Historically, the study of histone methylation gained momentum in the early 2000s, following the discovery that histones were not merely structural components of chromatin but active participants in gene regulation. The identification of specific histone methylases advanced our understanding of how methylation influences the chromatin landscape, paving the way for further investigations into their roles in health and disease.
Key Findings and Discussion
Major results of the study
Recent studies have categorized histone methylases into different families based on their structure and function. Significant findings have demonstrated how specific methylation patterns correlate with gene expression levels. For example, trimethylation of histone at lysine 4 (K4me3) is often associated with active transcription, while trimethylation at lysine 27 (K27me3) usually indicates transcriptional repression.
Detailed analysis of findings
The activity of histone methylases is governed by various factors, including the availability of substrates and cofactors. Moreover, its implications extend beyond gene regulation. Histone methylation can influence cell differentiation, development, and responses to environmental signals. Understanding these processes can lead to better insights into diseases where gene regulation is faulty. For instance, in certain cancers, abnormal histone methylation patterns can turn on oncogenes or silence tumor suppressor genes.
"Histone methylation is not just a marker but an integral player in the dynamics of gene expression."
As researchers continue to unravel the mechanisms of histone methylases, the need for targeted therapies becomes increasingly evident. By modulating the action of these enzymes, it may be possible to reverse abnormal gene expression found in many diseases.
Preface to Histone Methylases
Histone methylases play a pivotal role in the intricate regulation of gene expression by modifying histones, the proteins around which DNA is tightly coiled. This modification is essential for various biological processes, influencing the accessibility of genetic material for transcription. Understanding histone methylases is critical for elucidating mechanisms underlying cellular function, differentiation, and response to environmental signals. The study of these enzymes offers insights into both normal physiological processes and the aberrations associated with various diseases.
Definition of Histone Methylases
Histone methylases are enzymes that attach methyl groups to specific amino acid residues within histone proteins, primarily lysine and arginine. The action of these enzymes can lead to either activation or repression of gene expression, depending on the location and nature of the methylation. These modifications can serve as a signal for other proteins to bind, influencing the structure of chromatin. More compact chromatin is generally associated with gene silencing, while less condensed chromatin is linked with gene activation. The general understanding of histone methylases thus encompasses both their structural role in chromatin and their functional consequences in gene regulation.
Historical Context and Discovery
The discovery of histone methylases was part of a broader exploration into the field of epigenetics, emerging from the need to understand how genetic information can be regulated without altering the underlying DNA sequence. The first hints of the existence of histone modifications surfaced in the 1960s and 1970s. However, it was not until the late 1990s that specific methylases were characterized.
In particular, the identification of the SET domain as a hallmark of many methylases marked a significant advancement. This led to a deeper understanding of how these enzymes contributed to the functional diversity of histone modifications. By identifying various histone methylases, such as the well-studied SET1 and EZ, researchers revealed the complexity of these enzymes and their ability to influence diverse biological outcomes. The ongoing research continues to uncover novel histone methylases and their specific roles, which are essential for a comprehensive understanding of gene regulation.
Mechanism of Action
Histone methylases play a fundamental role in the intricate dance of gene regulation. Their mechanisms of action are essential for understanding how gene expression is finely tuned. Methylation affects chromatin structure and function, ultimately influencing transcriptional outcomes. Dissecting these mechanisms sheds light on cellular behavior and the intricate biochemistry that underlies life itself.
Methylation Process
The methylation process carried out by histone methylases involves the transfer of a methyl group from a donor molecule, typically S-adenosylmethionine (SAM), to specific lysine or arginine residues on histone proteins. This process is not merely a chemical modification; it has profound implications for chromatin dynamics. The addition of a methyl group can lead to various functional outcomes, including changes in nucleosome structure and recruitment of chromatin remodeling complexes.
The steps involved in the methylation process include:
- Binding of the enzyme: Histone methylases must identify and bind to their specific histone substrates. This specificity ensures the correct modifications at the right genetic locations.
- Transfer of the methyl group: After binding, the methyl group from SAM is transferred to the histone residue, resulting in a methylated histone.
- Conformational changes in chromatin: Once methylated, these histones can alter the compactness of chromatin, facilitating or hindering recruitment of transcription factors, depending on the type of modification.
This process is regulated tightly, as improper methylation can lead to aberrant gene expression patterns, which may have downstream effects on cell function and behavior.
Types of Methylation Modifications
Histone methylation is nuanced, characterized by different types of modifications, primarily distinguished by the number of methyl groups added. The key types include:
- Monomethylation: Involves the addition of a single methyl group. This modification is often associated with transcriptional activation but can be context-dependent.
- Dimethylation: Two methyl groups are added to the same residue. This modification can promote a more stable activation state in some scenarios but can also signal repression in others.
- Trimethylation: The addition of three methyl groups completely alters chromatin accessibility and often leads to silencing of gene expression.
Different combinations of these modifications can dictate the functional outcomes of gene expression. For example, trimethylation of lysine 4 on histone (K4me3) is often linked with active promoters, whereas trimethylation of lysine 27 on histone (K27me3) is more closely associated with gene repression. The intricate interplay of these variations highlights the complexity of epigenetic regulation and the critical role histone methylases play in this process.
Understanding the mechanisms of histone methylases is crucial for grasping how gene expression is controlled and modulated in various cellular contexts.
Thus, the methylation process and the types of modifications are vital for comprehending how methylases direct gene regulation, ultimately impacting both healthy physiological processes and disease states.
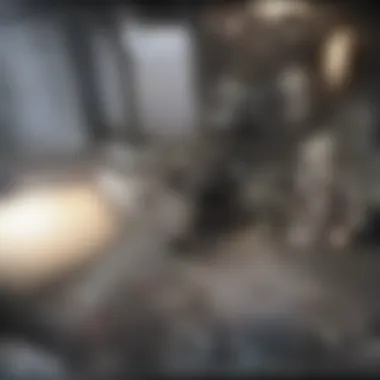
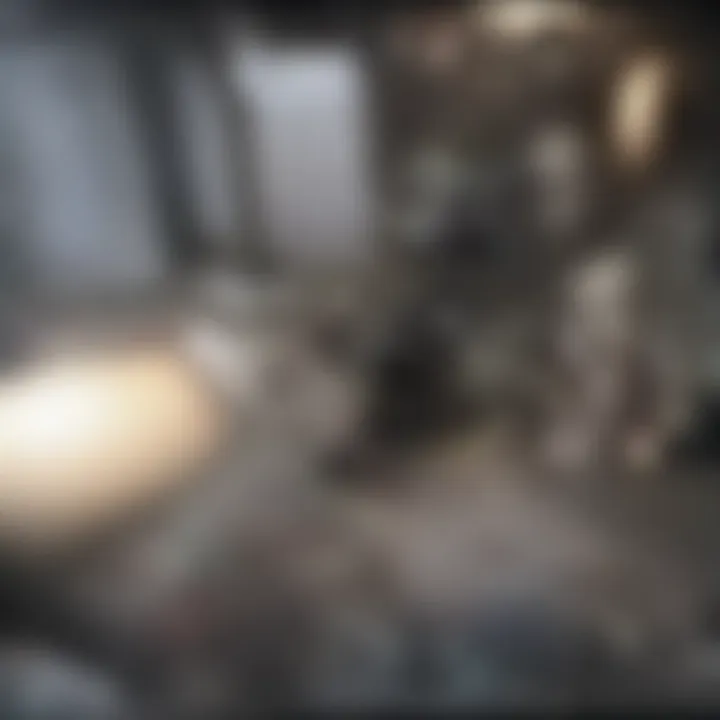
Classification of Histone Methylases
The classification of histone methylases is crucial for understanding their diverse roles in cellular processes and gene regulation. Recognizing the distinct types of these enzymes allows researchers to elucidate their specific functions and interactions with other epigenetic factors. Furthermore, this classification system enables scientists to identify potential therapeutic targets in various diseases, such as cancers and neurodegenerative disorders. By examining the differences and similarities between the classifications, one can appreciate the complexities of histone methylation and its broader implications in genetics.
Set Domain Containing Methylases
Set domain containing methylases represent a prominent group in the classification of histone methylases. These enzymes are characterized by the presence of a conserved SET domain, which plays a pivotal role in the transfer of methyl groups to specific lysine residues on histones. One well-known example includes the Enhancer of Zeste Homolog 2, or EZ, which is associated with gene repression and plays a vital role in development and differentiation.
These methylases are involved in various biological processes, including:
- Gene silencing: They facilitate transcriptional repression by modifying histones, ultimately leading to the formation of heterochromatin.
- Developmental regulation: Set domain containing methylases are crucial during embryonic development, influencing cell fate decisions and organogenesis.
- Response to signals: These enzymes can respond to external stimuli, responding quickly to environmental changes.
Importantly, the dysregulation of Set domain containing methylases has been implicated in several diseases, including cancer. Their role in maintaining gene expression patterns makes them prime targets for therapeutic interventions.
Non-Set Domain Methylases
Non-set domain methylases reflect another category within histone methylases that are vital for gene expression regulation. Unlike their Set domain counterparts, these enzymes lack the SET domain but still possess the ability to catalyze methylation. Some well-studied examples include the protein Arginine Methyltransferase 1, known as PRMT1. Non-set domain methylases typically exhibit different substrate specificities and preferences, often targeting arginine residues directly rather than lysine residues on histones.
The contributions of non-set domain methylases to cellular function include:
- Regulation of gene transcription: They can activate or repress specific gene targets, based on the methylation status of histones.
- Influence on protein-protein interactions: These methylases modify histones in a manner that can alter chromatin structure, subsequently affecting the recruitment of transcription factors.
- Interplay with other post-translational modifications: Often, non-set domain methylases interact synergistically with acetylation and phosphorylation, forming a complex signaling network.
Overall, understanding the characteristics and functions of non-set domain methylases adds significant depth to the exploration of histone methylation and its impact on gene regulation.
Role in Gene Regulation
The role of histone methylases in gene regulation is a crucial aspect of understanding how genes are expressed or silenced within cells. These enzymes influence various biological processes by modifying histone proteins through the addition of methyl groups. This modification changes how tightly or loosely DNA is packaged around histones, directly impacting gene accessibility. As a result, histone methylases can dramatically affect cellular function, phenotype, and response to environmental stimuli.
In the realm of gene regulation, histone methylases play two primary roles: activation and repression of gene expression. Understanding these dual functions sheds light on how genes are selectively turned on or off, which is vital for cellular differentiation and function. Their precise regulation is fundamental to many biological processes, including development, immune responses, and cellular homeostasis. Moreover, dysregulation of these enzymes can lead to various diseases, highlighting their significance.
Key aspects that define the importance of histone methylases in gene regulation include:
- Molecular Complexity: Histone methylation involves multiple methyl groups and various states (monomethylation, dimethylation, trimethylation), resulting in diverse outcomes for gene regulation.
- Context Dependence: The function of histone methylases is context-dependent; the same methylation mark can have opposite effects depending on the cellular or environmental context.
- Integration with Other Signals: Histone methylation does not act alone. It interacts with other epigenetic modifications and transcription factors, creating a complex regulatory network.
Understanding the role of histone methylases provides insights into fundamental biological mechanisms and potential therapeutic avenues for diseases linked with improper gene regulation.
Activation of Gene Expression
Histone methylation is associated with gene activation primarily through specific methylation marks on histones and . For instance, trimethylation at lysine 4 of histone (K4me3) is generally a hallmark of actively transcribed genes. This process of activation involves the recruiting of transcriptional machinery, which includes RNA polymerase II and other essential cofactors.
When histones are methylated, the structure of chromatin becomes less condensed, allowing transcription factors better access to DNA. This accessibility is paramount for the initiation of transcription. Additionally, certain proteins known as coactivators can bind to methylated histones, further stabilizing the transcription complex and promoting gene expression.
Essential points regarding activation include:
- Specific Methylation Patterns: Different histone methylation marks can signify active transcription, providing a unique code that distinguishes active genes from silenced ones.
- Synergistic Effects: Activation is often supported by the interplay of different epigenetic events, including histone acetylation, adding complexity to the regulation.
"Understanding histone methylation helps decipher the syntax of gene expression, revealing how cells respond differently under various circumstances."
Repression of Gene Expression
Conversely, histone methylation can also lead to gene repression, with particular emphasis on methylation at lysine 27 of histone (K27me3). This mark is often associated with silenced or lowly expressed genes. Repressive histone methylation attracts proteins that can compact chromatin, thus making DNA less accessible for transcription. Such changes can inhibit the binding of transcription factors and RNA Polymerase II, leading to reduced or halted gene expression.
Not all genes will be repressed in the same manner. This variation depends on the location and context of methylation marks. Factors that dictate the repressive effect include:
- Histone Variants: Different histones can affect how repression is implemented; for example, the presence of specific histone variants may enhance or negate the repressive effect.
- Complex Interactions: Repressed genes do not operate in isolation and can be affected by nearby active genes through mechanisms like transcriptional interference.
In summary, the dual role of histone methylases in both activating and repressing gene expression illustrates the complexity of gene regulation. Their importance cannot be overstated, as they present potential points of intervention for understanding diseases driven by epigenetic changes.
Histone Methylation and Epigenetics
Histone methylation stands as a crucial mechanism in the realm of epigenetics, influencing gene expression and cellular function significantly. This part of the article addresses how histone methylation contributes to the structural dynamics of chromatin and the implications this modification holds for various biological processes. Understanding histone methylation unveils the complex layers regulating gene activity beyond genetic sequences alone.
Histone methylation refers to the addition of methyl groups to the amino acids of histone proteins. This modification alters how DNA is packaged in the nucleus, subsequently affecting gene accessibility for transcription. When histones are methylated, the derepression or repression of gene expression can occur, making it a vital player in gene regulation. This importance is particularly evident in both development and cellular response to environmental stimuli.
Epigenetic Modifications Explained
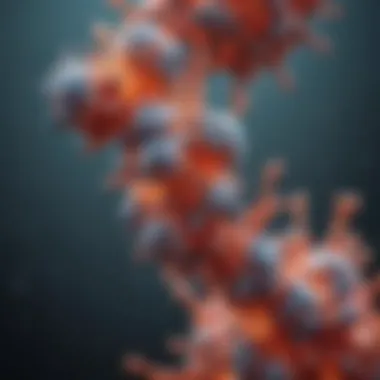
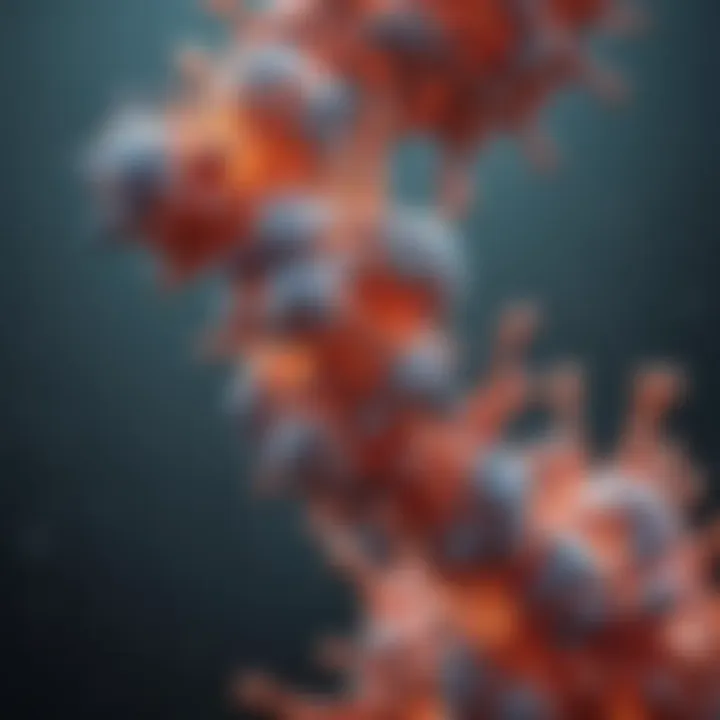
Epigenetic modifications are critical for the expression patterns observed in different cell types. These modifications include methylation, acetylation, phosphorylation, and ubiquitylation, all contributing to the regulation of gene expression without changing the underlying DNA sequence. The study of histone methylation falls under this broader umbrella of epigenetic regulation, emphasizing how chemical changes can dictate cellular identity and function.
Among the various modifications, histone methylation is unique due to the specific roles it can play regarding gene activation and repression. For instance, the trimethylation of lysine 4 on histone is generally correlated with active gene transcription, whereas trimethylation of lysine 27 on the same histone is associated with gene silencing. This duality illustrates the tuning ability of histone methylation in gene regulation and highlights its importance in health and disease.
Interaction with Other Epigenetic Marks
Histone methylation does not act in isolation; it interacts intricately with other epigenetic marks resulting in a complex regulatory network. For example, histone acetylation is often coupled with methylation, leading to synergistic or antagonistic effects on gene expression. The interplay between these marks serves as a mechanism to finely tune gene expression.
In addition to acetylation, histone methylation interacts with DNA methylation. DNA methylation, the addition of methyl groups to cytosine residues in the DNA, can complement histone modifications, creating a stable framework for transcriptional repression. When both histones and DNA are methylated, the chromatin becomes more compacted, effectively silencing the genes in that region.
"The combined effects of histone methylation and other epigenetic modifications underscore the complexity of gene regulation, opening avenues for understanding the processes of development and disease."
Through the lens of epigenetics, histone methylases offer valuable insights into the principles of heredity that extend beyond genetic makeup. By studying these modifications, researchers can unravel the links between epigenetic changes and health outcomes, leading to potential therapeutic interventions. Understanding this interplay is essential for grasping how cells can inherit traits not solely driven by DNA sequence changes, but also by reversible modifications impacting gene expression.
Histone Methylases in Development
Histone methylases play a crucial role in development by modulating gene expression patterns that influence cellular behavior and fate. By adding methyl groups to histones, these enzymes help regulate chromatin structure and function. This precise control is essential during the various stages of development, where differentiation and cellular identity must be established and maintained.
Understanding histone methylases in this context is important for several reasons. First, it illuminates how epigenetic mechanisms shape embryo formation and organogenesis. Second, it highlights potential consequences of dysregulation in these enzymes, which could lead to developmental disorders. Ultimately, studying histone methylases gives insights into both normal and abnormal developmental trajectories.
Role in Embryonic Development
During embryonic development, histone methylases are integral for managing the timing of gene expression. Different stages of development require specific genes to be turned on or off. Histone methylation patterns help dictate when these genes should be active. For instance, certain methylases are activated to promote genes necessary for the formation of vital structures like limbs or organs. Conversely, other methylases might silence genes that are no longer needed.
Several key histone methylases have been studied in the context of early development. For example, the EZ enzyme, part of the Polycomb repressive complex, is known to facilitate gene silencing that is crucial for proper embryonic stem cell maintenance and differentiation into various cell types. If the functions of these methylases are disrupted, significant developmental anomalies can occur, underscoring their importance.
Impact on Differentiation Processes
Histone methylases also play a pivotal role in differentiation processes. As stem cells continue to divide and develop, they must transition into specialized cell types. Methylation patterns can guide this transition by modifying histones associated with genes responsible for specific functions. Depending on the signals received by the cell, particular methylases will either activate or repress genes that drive differentiation.
For example, in the process of neuronal differentiation, certain histone methylases might activate genes that promote neuron formation while silencing those associated with other lineages. This fine-tuning is not only fundamental for healthy development but also for maintaining the balance of cell populations within tissues. Disruption in these pathways due to faulty methylase activity can lead to problems such as impaired cellular identity or failure to respond appropriately to signaling cues.
In summary, histone methylases have a profound impact on both embryonic development and differentiation processes. Their ability to control gene expression through epigenetic modification highlights their critical role in shaping cellular identity and functionality.
Histone Methylases in Disease
Histone methylases play a crucial role in the regulation of gene expression, which has significant implications for various diseases. Dysregulation of histone methylation can lead to altered chromatin states, impacting cellular functions and contributing to disease pathogenesis. Understanding the involvement of these enzymes in disease processes helps in identifying potential therapeutic targets.
Associations with Cancers
Histone methylases have been extensively studied in relation to cancer. They can contribute to oncogenesis either through overexpression or mutations. For example, the overexpression of various histone methylases, such as EZ (Enhancer of Zeste Homolog 2), has been linked to numerous cancers, including breast, prostate, and lymphoma. This enzyme is part of the Polycomb Repressive Complex 2 (PRC2) and is responsible for trimethylating histone at lysine 27 (K27me3), which leads to gene silencing.
The inhibition of EZ is being explored as a cancer therapy. Several small molecules that inhibit EZ have entered clinical trials. Notably, studies have shown that targeting EZ can reverse the silencing of tumor suppressor genes, thus restoring their expression and inhibiting cancer cell proliferation. Moreover, SETDB1, another histone methylase, has been noted for its role in triggering cellular transformation, solidifying its relevance as a tumor-associated factor.
The implications of histone methylases in cancer extend to understanding the mechanisms behind metastasis as well. Certain methylases may affect gene expression patterns that drive the invasive characteristics of tumor cells, revealing further avenues for therapeutic intervention.
Role in Neurological Disorders
In addition to their involvement in cancer, histone methylases are significant in the context of neurological disorders. Methylation modifications on histones can influence the expression of genes critical for neuronal function and repair. Disorders such as schizophrenia, Alzheimer’s disease, and autism spectrum disorders have been linked to aberrant histone methylation patterns.
For instance, the dysregulation of G9a, a histone methylase, has been implicated in Alzheimer's disease through its role in the repression of neuroprotective genes. The reduction of G9a activity in animal models has demonstrated enhancements in cognitive functions, suggesting that targeting this enzyme could provide a novel approach to treating cognitive decline.
Another example is the involvement of Nsd2, a histone methylase that mediates the trimethylation of K36. Its expression levels have been associated with increased susceptibility to autism, highlighting how histone methylation affects brain development and function.
"The understanding of histone methylases in the context of neurological disorders opens a window to developing targeted therapies that can affect epigenetic regulation, potentially leading to better management strategies."
In summary, histone methylases provide valuable insights into disease mechanisms including cancers and neurological disorders. Their role as modifiers of gene expression is essential not only for normal cellular function but also as potential therapeutic targets. Continued research on these enzymes promises to enhance our understanding of their complex functions and their implications across various disease states.
Therapeutic Targeting of Histone Methylases
The study of histone methylases extends beyond basic biological functions to therapeutic implications in various diseases. Targeting histone methylases is becoming increasingly relevant in the development of strategies to treat cancers and other disorders linked to epigenetic modifications. These enzymes are crucial in regulating gene expression through their ability to add methyl groups to histones. By modulating their activity, researchers can influence chromatin structure, impacting how genes are expressed or silenced.
Development of Inhibitors
Creating inhibitors for histone methylases is a focal point for researchers aiming to harness their regulatory properties for therapeutic benefits. Inhibitors can help restore normal gene expression patterns in cells where histone methylation is dysregulated. Multiple approaches are being explored to create these inhibitors. One promising strategy involves small molecules that specifically inhibit the enzymatic activity of certain histone methylases, like EZ or DOT1L.
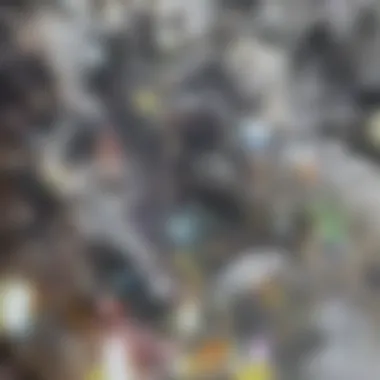
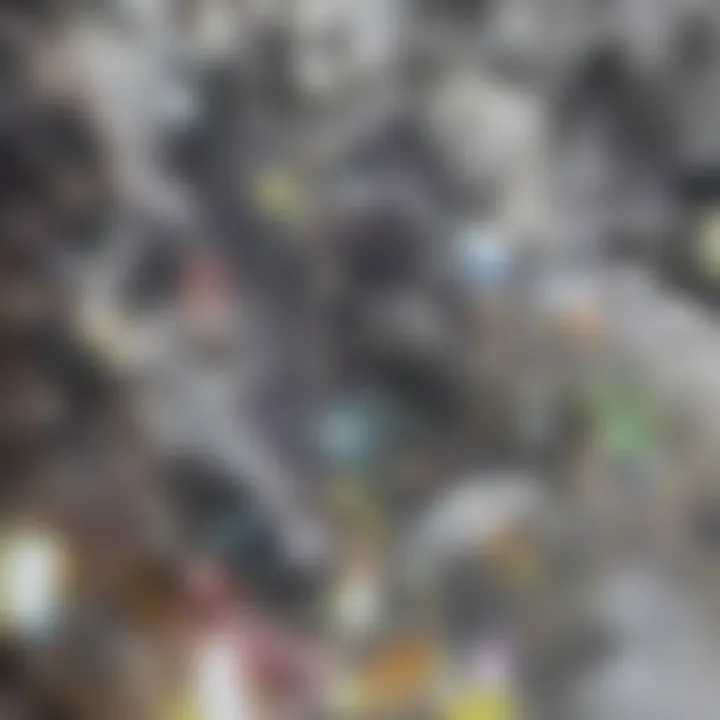
- Specificity: These inhibitors must be designed to target specific methylases to avoid off-target effects that could dysregulate other essential pathways.
- Screening Technologies: Innovative screening technologies, such as high-throughput screening, facilitate the identification of effective compounds.
- Synthetic Biology Tools: Techniques from synthetic biology can also aid in designing better inhibitors that are tailored for specific contexts of cellular or tissue type.
"Targeting histone methylases offers a new avenue for precision medicine, allowing tailored therapies based on individual epigenomic landscapes."
Clinical Applications and Trials
The progression from laboratory findings to clinical applications is a meticulous journey. Several clinical trials have been initiated to evaluate potential inhibitors of histone methylases in treating malignancies like leukemia and solid tumors.
- GSK126: An inhibitor of EZ that has shown promise in clinical settings, especially in lymphomas with EZ mutations.
- EPZ-6438: Another compound currently being tested, focusing on its efficacy in hematologic cancers.
The outcomes of these trials will greatly inform the future of epigenetic therapies and their role in cancer treatment. There is also ongoing research into using combinations of methylase inhibitors with other therapeutic agents to enhance treatment efficacy. Excellent progress has been made, but research continues to refine these methods and establish clear guidelines for clinical use.
In summary, targeting histone methylases represents a rapidly evolving area with the potential to change therapeutic paradigms for various diseases. The development of inhibitors and their progress through clinical trials could lead to innovative treatments that leverage the understanding of epigenetics in disease management.
Experimental Techniques to Study Histone Methylases
Studying histone methylases is essential for understanding their roles in gene regulation and the broader implications for epigenomics. Various experimental techniques enable researchers to elucidate the functions and characteristics of these enzymes. Each method offers distinct advantages and considerations.
Employing the right techniques can validate the role of histone methylases in specific biological contexts. Accuracy in measurements, reproducibility of results, and the capability to discern between different methylation states are key factors in the analysis. Moreover, exploration of these techniques provides insights that could lead to effective therapeutic targets.
Chromatin Immunoprecipitation Sequencing (ChIP-Seq)
Chromatin Immunoprecipitation Sequencing, commonly known as ChIP-Seq, is a powerful technique used to study protein-DNA interactions, particularly involving histone modifications. This method provides a means to identify the binding sites of histone methylases across the genome.
The process begins with cross-linking proteins to DNA, followed by fragmentation and immunoprecipitation using specific antibodies against target histone marks. Upon purification of the DNA, next-generation sequencing reveals the regions associated with histone methylation.
Some benefits of ChIP-Seq include:
- High resolution: It allows mapping of histone modifications with base-pair resolution.
- Genome-wide analysis: Researchers can study methylase activity across thousands of genes simultaneously.
- Insights into gene regulation: Patterns of methylation directly correlate to gene expression changes.
However, some considerations exist:
- Antibody specificity: Choosing the right antibody is critical for reducing background noise.
- Cross-linking efficiency: Inefficient cross-linking can lead to low signal and loss of precision in results.
Mass Spectrometry for Methylation Analysis
Mass spectrometry (MS) is another vital technique for studying histone methylation. It quantifies specific histone marks and characterizes the types of methylation present on histones. By directly analyzing histone proteins, researchers can obtain detailed information about methylation states at the amino acid level.
The workflow typically involves:
- Protein Extraction: Isolate histones from cellular samples.
- Proteolytic Digestion: Fragment histones into peptides using enzymes like trypsin.
- Ionization: Peptides are ionized and entered into the mass spectrometer.
- Detection: The mass-to-charge ratio (m/z) is measured to identify and quantify methylation patterns.
Advantages of mass spectrometry include:
- Sensitivity: It can detect low-abundance modifications.
- Detailed structural information: MS provides insights into different types of methylation and mixed states.
Nevertheless, the technique has some limitations:
- Complexity of analysis: Data interpretation may require specialized knowledge and software.
- Sample preparation: The quality of the sample preservation can significantly affect results.
Overall, both ChIP-Seq and mass spectrometry are essential tools in the study of histone methylases, offering complementary information that enhances our understanding of their biological roles.
Future Directions in Histone Methylase Research
The exploration of future directions in histone methylase research is crucial to not only deepen our understanding of the mechanisms behind gene regulation but also to open pathways for potential therapeutic interventions. As studies continue to unveil the numerous roles that histone methylases play in various biological processes, there is an increasing need to focus on novel aspects that could transform current knowledge into practical applications.
Exploration of Novel Methylase Substrates
One significant avenue for future research involves the investigation of novel substrates for histone methylases. Traditional studies have largely focused on well-characterized substrates. However, expanding this scope can reveal previously unknown interactions and modifications that may have profound implications for gene expression regulations and cellular function. Researchers are encouraged to employ high-throughput screening techniques and advanced mass spectrometry methods to identify new substrates effectively. This could also lead to discovering atypical roles of existing methylases or uncovering other molecular targets that contribute to cellular processes.
- Benefits: Understanding new substrates helps in delineating the broader impact of histone methylation on gene regulation.
- Considerations: These investigations must consider the contextual nature of histone modifications, as one substrate's effect may significantly vary depending on the cellular environment.
Integration with Genomic Technologies
Another promising direction is the integration of histone methylase studies with genomic technologies such as CRISPR-Cas9 and single-cell sequencing. By using CRISPR to gene-edit histone methylases, researchers can observe direct impacts on methylation patterns and gene expression in real-time. This precise manipulation allows for the assessment of the causative relationships rather than merely correlational studies.
Moreover, employing single-cell sequencing technologies can reveal heterogeneity in histone modification patterns that exists within cell populations. This provides crucial insights that can enhance our understanding of disease mechanisms, especially in heterogeneous diseases such as cancers.
- Benefits: Integration with technological advancements enhances the resolution of investigations and provides more comprehensive data.
- Considerations: As technology continues to evolve, ensuring that methodologies remain robust and reproducible is vital for validating findings.
"The future research agenda should encompass innovative strategies, aligning advanced technologies with histone methylase functionalities for transformative insights into gene regulation."