Understanding Gradient Gel Electrophoresis Techniques
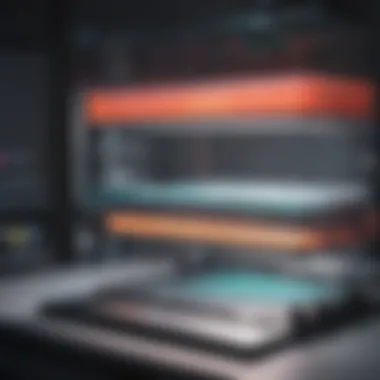
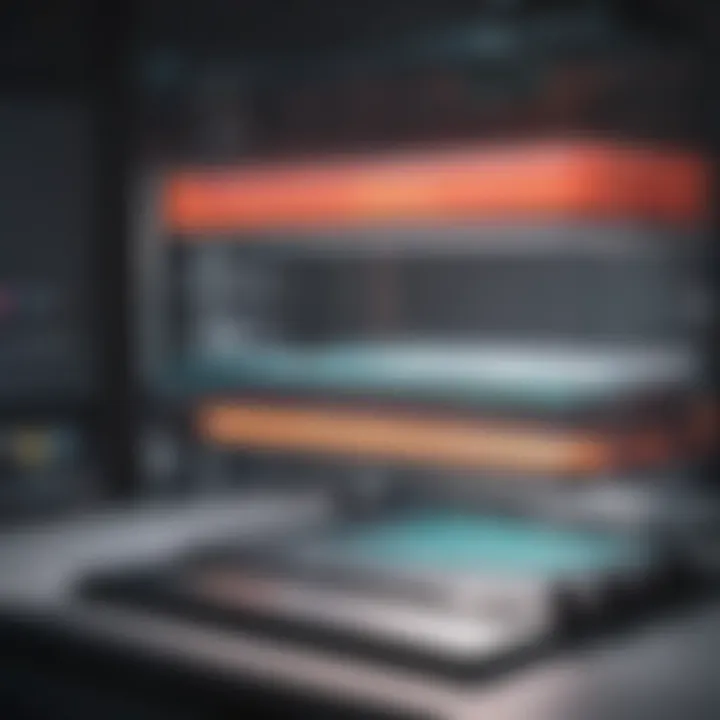
Intro
Gradient gel electrophoresis is a pivotal technique extensively utilized in the fields of molecular biology and biochemistry. It plays a critical role in the analysis of various biomolecules, especially proteins. This method allows for the separation of biomolecules based on their size and charge, providing valuable insights into their functions and interactions. Understanding the principles, applications, and practical considerations of gradient gel electrophoresis can significantly enhance research outcomes in life sciences.
Background and Context
Overview of the research topic
Gradient gel electrophoresis is integral in studying proteins, nucleic acids, and other biomolecules. This technique utilizes a gradient of polyacrylamide or agarose in the gel, allowing different molecular weights to migrate at varying speeds. As a result, a comprehensive separation of components occurs, enabling researchers to analyze complex biological samples efficiently. The ability to dissect mixtures into their individual components has profound implications in proteomics, genomics, and diagnostics.
Historical significance
The evolution of gel electrophoresis can be traced back to the mid-20th century. Scientists began experimenting with polyacrylamide gels, leading to the development of various techniques. In the 1960s, researchers like A.J. Hillyard started employing gradient gels, recognizing their enhanced resolution capabilities. Since then, advancements in buffering systems and imaging technologies further refined this method, making it indispensable in modern biochemical research.
Key Findings and Discussion
Major results of the study
Recent studies emphasize the utility of gradient gel electrophoresis in numerous applications:
- Characterization of protein subunits and complexes
- Analysis of post-translational modifications
- Quality control in biologics production
- Cloning and sequencing of nucleic acids
These findings underscore the importance of this technique in generating precise data essential for advancing scientific knowledge.
Detailed analysis of findings
In the practice of gradient gel electrophoresis, numerous parameters influence the results. The concentration of the gel, running buffer composition, voltage applied, and temperature can all affect separation efficacy. Understanding and optimizing these factors is crucial for achieving accurate and reproducible results.
Additionally, common challenges during experimentation include band smearing, poor resolution, and inconsistent migration. Implementing meticulous gel preparation and optimizing electrophoresis conditions can mitigate these issues.
"Gradient gel electrophoresis stands as a cornerstone in biochemical analysis, crucial for the accurate interpretation of molecular data."
With increased demand for precision in scientific studies, gradient gel electrophoresis will continue to evolve, adapting to the needs of researchers worldwide.
By dissecting these elements, the article not only aims to present a comprehensive picture of gradient gel electrophoresis but also encourages researchers to apply this technique thoughtfully in their work.
Preface to Gradient Gel Electrophoresis
Gradient gel electrophoresis serves as a pivotal technique in molecular biology, with significant applicability in protein and nucleic acid analysis. This section introduces the topic, emphasizing its relevance in scientific research and diagnostics. Gradient gel electrophoresis allows for the separation of biomolecules based on size and charge, contributing to the identification and characterization of proteins, DNA, and RNA. Understanding the fundamentals of this technique is essential for students and professionals in the life sciences.
Definition and Overview
Gradient gel electrophoresis is a refined version of standard gel electrophoresis. In this method, a gradient of polymer concentration is established within the gel. This gradient results in varying pore sizes throughout the gel matrix, enabling more effective separation of molecules. The larger pores found at the top of the gel allow bigger molecules to migrate freely, while smaller pores at the bottom hinder their movement. Consequently, this creates a more defined separation based on the size and charge of biomolecules. In essence, gradient gel electrophoresis enhances the resolution of bands when analyzing complex mixtures. Researchers often use it to examine proteins or nucleic acids, especially when sample purity is critical.
Historical Context
The evolution of gradient gel electrophoresis dates back to the mid-1970s. It was developed as a response to the growing need for better resolution methods in protein analysis. Early versions utilized a single concentration of agarose or polyacrylamide, which limited the ability to separate proteins with small differences in size. The introduction of gradient gels marked a significant breakthrough in electrophoretic techniques.
Over the years, advancements in equipment and gel formulations have refined the methodology. Today, gradient gel electrophoresis is a staple in laboratories worldwide, drawing from its historical roots while also integrating modern technologies. The continuous improvement in imaging and detection techniques further enhances its applicability, making it indispensable in contemporary biochemical research.
Principles of Gel Electrophoresis
The principles of gel electrophoresis form the cornerstone of the technique, encapsulating the fundamental interactions between molecules and the gel medium. Understanding these principles is crucial for anyone focusing on molecular biology, as they dictate how biomolecules behave under an electric field. This section delves into key elements, underlying benefits, and important considerations related to these principles in the context of gradient gel electrophoresis.
Fundamental Concepts
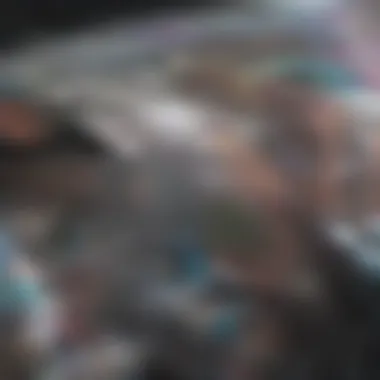
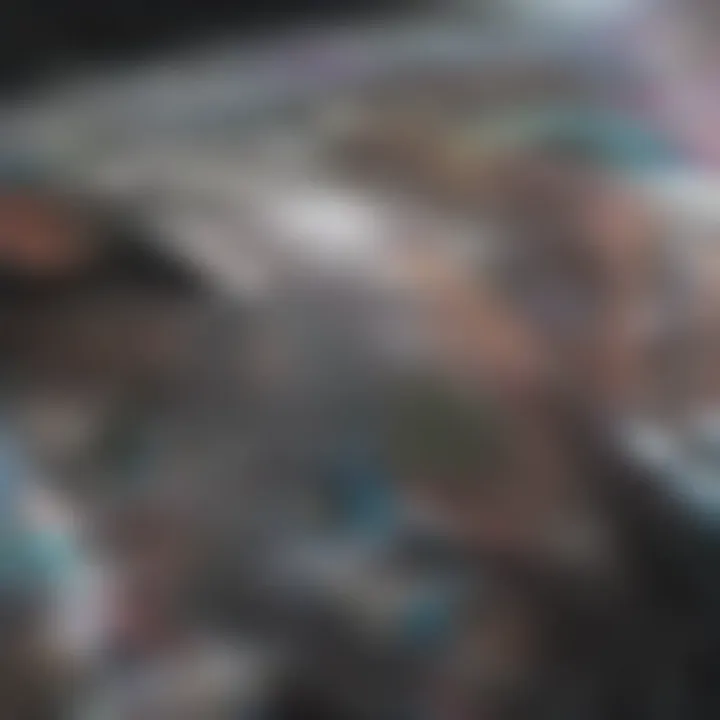
At its core, gel electrophoresis employs an electric field to separate charged molecules based on size and charge. The gel matrix acts as a sieve, where smaller molecules navigate through the pores more easily than larger ones. The formation of a gradient in the gel adds complexity, allowing for more refined separation.
A gradient gel is made of varying concentrations of agarose or polyacrylamide. As a result, molecules move through different densities, which affects their migration speed. The technique is particularly useful for proteins because it provides a clearer distinction between closely sized molecules.
When a sample containing proteins is loaded into the well of a gel and an electric current is applied, the proteins migrate towards the anode, usually positively charged, due to their intrinsic charge. Understanding the size and charge of the molecules in your sample is critical, as these factors directly influence how far and how fast they move through the gel.
Role of Charge and Size
The intrinsic properties of the molecules under investigation, namely their charge and size, play a pivotal role in gel electrophoresis. Proteins, for example, acquire a net charge based on their amino acid composition and the pH of the buffer solution. A protein’s isoelectric point (pI) will dictate its movement in the electric field. At a pH below pI, the protein has a positive charge and migrates towards the negative electrode. Conversely, at a pH above pI, it is negatively charged, moving towards the positive electrode.
Moreover, size contributes to the separation process. Smaller proteins can traverse the gel matrix more efficiently than larger ones. The relationship between size, charge, and gel composition determines the efficiency and resolution of separation. Therefore, careful consideration must also be given to the chemical properties of the gel used, as this will affect the results.
Types of Gels Used
Different types of gels can be employed in gel electrophoresis, each with its unique properties and applications. The two primary types are:
- Agarose Gel: Best suited for larger nucleic acids, agarose gels provide a relatively loose matrix suitable for DNA and RNA separation. The concentration of agarose can be adjusted to optimize the separation based on the size of the target molecules.
- Polyacrylamide Gel: Typically used for protein electrophoresis due to its ability to provide tighter control over pore size. It allows for the resolution of smaller differences between protein sizes. Polyacrylamide gels can be further modified to create gradient gels, enhancing their separation capabilities.
The choice of gel type significantly impacts the resolution and efficiency of separation, making it vital to select the appropriate medium based on the specific needs of the experiment.
In summary, the principles of gel electrophoresis revolve around understanding the charge and size of molecules, the gel medium used, and their interactions within an electric field. Mastery of these principles enables researchers to optimize their experimental setups, leading to better results and deeper insights in molecular biology.
Gradient Formation in Gels
Gradient formation in gels is a cornerstone of the gradient gel electrophoresis technique. Its significance becomes evident when considering how gradients influence the separation quality of biomolecules. This method enables differentiation based on both size and charge, enhancing the ability to analyze complex samples effectively. Understanding gradient formation not only aids in optimizing experimental conditions but also helps in interpreting results with precision. The careful construction of gradients can lead to substantial improvements in resolution and clarity in data obtained from electrophoresis.
Concept of Gradient Gels
Gradient gels are designed to have a variation in concentration. This variation often occurs in the acrylamide or agarose gel used. The gradient allows for a continuous transition of pore size, which is crucial for the separation of molecules. As a sample passes through the gel, different-sized proteins or nucleic acids experience varying resistance depending on their size. Larger molecules will move slowly through regions of denser gel, while smaller molecules pass through more easily. This principle of size-exclusion enables a refined separation mechanism. Importantly, the establishment of gradient gels can provide information about the heterogeneity in a sample, allowing for a deeper insight into the sample’s composition.
Methods for Creating Gradients
Creating gradients in gels involves several techniques. Some of the widely used methods include:
- Layering Method: This technique involves stacking multiple layers of gels with different concentrations. Each layer is sequentially poured, forming clear boundaries between them. However, this approach can lead to mixing at the interfaces, which may negatively affect resolution.
- Continuous Gradient: In this method, a single solution of varying concentration is polymerized to form a continuous gradient throughout the gel. This can often be achieved with specific devices that facilitate the mixing of different concentrations as they are dispensed. Examples include gradient makers that enhance uniformity.
- Freezing Technique: This approach utilizes the temperature to control the setting of gradients. Different temperatures can trigger varying polymerization rates. This method can also yield a stable and precise gradient but requires careful equipment control.
- Using Additives: Certain chemicals can be added to gels to create non-linear gradients. For instance, using sucrose in an agarose gel can affect gradient formation by altering viscosity, thus adjusting migration speed for different molecules.
The effectiveness of gradient gel electrophoresis largely depends on how well the gradient is created and maintained throughout the experiment.
Experimental Setup
In gradient gel electrophoresis, the experimental setup plays a crucial role in achieving accurate and reproducible results. This section carefully outlines the necessary equipment and the gel preparation protocol, which are essential for conducting successful electrophoresis. Having the right setup contributes significantly to data quality and facilitates efficient analysis of biomolecules. A well-organized experimental environment ensures that the electrophoresis procedure proceeds smoothly and allows for better optimization of separation conditions.
Equipment Required
To perform gradient gel electrophoresis, several key pieces of equipment are necessary. The list below provides an overview of the essential tools:
- Gel Casting Apparatus: This is used to create the gel itself. The apparatus must allow for adjustable thickness to accommodate varying gradient levels.
- Electrophoresis Unit: Comprised of two electrode chambers, these units help in applying the electric current. The unit should be compatible with the gel dimensions.
- Power Supply: A stable power supply is needed to provide consistent voltage and current throughout the experiment.
- Cooling System: Since heat can affect the separation process, a cooling system may be essential, especially for long runs.
- Pipettes and Tips: Accurate pipetting is crucial for loading samples onto the gel without causing degradation or distortion of results.
- Reagents and Chemicals: This includes agarose or polyacrylamide for the gel matrix, buffer solutions, and loading dyes.
- Imaging System: After electrophoresis, a system for visualizing the bands (e.g., UV transilluminators) is necessary for result interpretation.
Each piece of equipment should be regularly calibrated and maintained to ensure optimal performance. The choice of equipment might vary based on laboratory protocols and specific research requirements.
Gel Preparation Protocol
The preparation of gradient gels is a pivotal aspect of gradient gel electrophoresis. A well-prepared gel determines the efficacy of biomolecule separation. Below is a standard protocol for gel preparation:
- Choose Gel Composition: Select either agarose or polyacrylamide based on the molecule size. Agarose is often used for larger nucleic acids, while polyacrylamide is typically better for proteins.
- Prepare Gradient: Create a concentration gradient by mixing two or more stock solutions. For instance, when using polyacrylamide, prepare a solution of varying percentages to form a continuous gradient.
- Casting the Gel: Pour the mixed solution into the casting tray. Make sure to assemble the plates properly to avoid leakage during polymerization. Insert a comb to create wells for sample loading.
- Polymerization: Allow the gel to polymerize at room temperature or under mild conditions. Ensure the gel solidifies completely before removing the comb.
- Equilibrate the Gel: Before use, rinse the gel with running buffer to enhance ionic strength and minimize diffusion of the sample during loading.
- Load Samples: Carefully load the samples into the wells using a pipette. Avoid introducing bubbles which can interfere with migration paths.
The above steps may require fine-tuning based on specific experimental demands. A precise gel preparation enhances separational resolution and overall experimental outcomes.
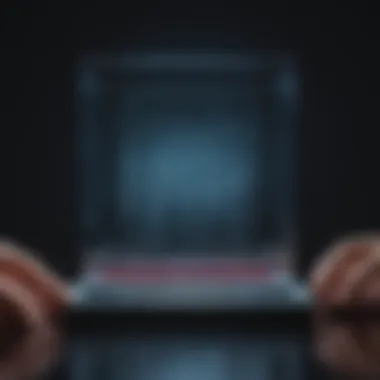
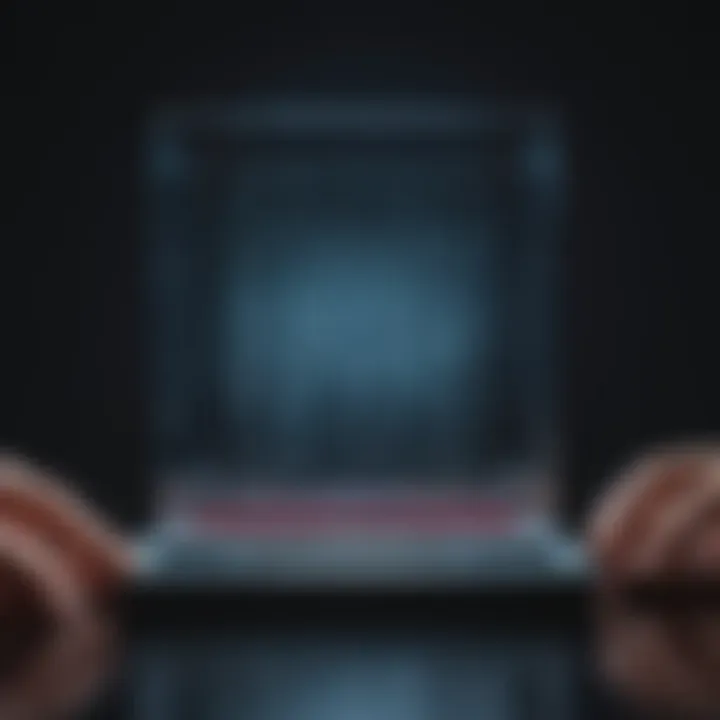
"Gradient gels provide a unique advantage by allowing for simultaneous separation of different molecule sizes within a single run, leading to much more efficient analyses."
In summary, a thorough understanding of the equipment required and gel preparation protocol forms the backbone of effective gradient gel electrophoresis. Proper setup enhances the quality of results, ultimately contributing to advancements in molecular biology research.
Running the Electrophoresis
Running the electrophoresis is a critical phase in the gradient gel electrophoresis process. Here, the actual separation of biomolecules occurs. This section will outline key elements that influence the effectiveness of separation and the detection of the target molecules. It provides insights into this essential technique that is widely used in various scientific fields, such as biochemistry and molecular biology. The precision of this step significantly impacts the overall results and interpretations.
Parameters Influencing Separation
Several parameters play a significant role in the effectiveness of separation during electrophoresis. Understanding these factors is essential for optimizing results. Key parameters include:
- Voltage: The applied voltage determines the electric field strength. Higher voltages accelerate the movement of biomolecules but can lead to overheating, affecting separation quality.
- Gel Concentration: The concentration of the gel affects the porosity, influencing how easily molecules can migrate through. A higher concentration gel is better for smaller molecules, while lower concentrations favor larger ones.
- Running Buffer: The composition of the running buffer is also vital. It must provide the necessary ions for conductivity, ensuring that the electric current can flow efficiently.
- Temperature Control: Temperature affects gel viscosity and molecule mobility. Controlled temperature is essential to prevent heat-induced artifacts that could corrupt the data.
- Time of Run: The duration of the run affects separation resolution. Longer runs can lead to better separation but may also cause diffusion of bands, which can obscure results.
By carefully managing these parameters, researchers can greatly enhance the clarity and accuracy of their results.
Detection Methods
Detection methods are crucial for identifying and analyzing the separated biomolecules post-electrophoresis. Without effective detection, the results can be meaningless. Typically used detection methods include:
- Staining Techniques: Commonly used stains include Coomassie Brilliant Blue for proteins and ethidium bromide for nucleic acids. These stains make bands visible under UV light or specific wavelengths.
- Western Blotting: This is a more advanced technique that allows for the detection of specific proteins after separation. It combines gel electrophoresis with antibody-based detection, enhancing specificity.
- Fluorescent Labels: These are often used for nucleic acid analysis and provide high sensitivity. Labels attached to biomolecules fluoresce under specific conditions, allowing for easy tracking.
- Mass Spectrometry: Though not used directly with gel electrophoresis, it can analyze separated compounds' mass-to-charge ratios, offering detailed information on the molecular makeup of samples.
Effective detection methods not only enhance the accuracy of analyses but also expand the potential applications of gradient gel electrophoresis in research and clinical diagnostics.
Applications of Gradient Gel Electrophoresis
Gradient gel electrophoresis plays a crucial role in molecular biology and biochemistry. This technique allows scientists to separate biomolecules based on size, charge, and other physical properties. As a result, it finds extensive applications across various domains, including protein analysis, nucleic acid studies, and clinical diagnostics. Understanding these applications is vital for appreciating the broader implications of gradient gel electrophoresis.
Protein Analysis
In protein analysis, gradient gel electrophoresis stands out as an essential method for resolving complex mixtures. By employing a gradient of acrylamide, this technique enables the separation of proteins with similar molecular weights. The concentration gradient of the gel enhances resolution and provides clearer banding patterns. Researchers often utilize this method to determine protein purity, identify post-translational modifications, and characterize protein interactions. Additionally, it plays a role in evaluating protein expression levels under different conditions.
The ability to visualize proteins after electrophoresis is paramount. Staining techniques, like Coomassie Brilliant Blue or silver staining, allow scientists to observe band intensity and correlate it with protein quantity. Analyzing these bands helps in identifying specific proteins that contribute to cellular processes or disease states. Furthermore, researchers can utilize techniques such as Western blotting following gradient gel electrophoresis to further validate findings. This step adds an extra layer of specificity in protein analysis.
Nucleic Acid Studies
Gradient gel electrophoresis is also pivotal in the study of nucleic acids, particularly DNA and RNA. It allows scientists to separate nucleic acid fragments based on size, which is fundamental for various applications, including restriction fragment length polymorphism (RFLP) analysis and the verification of PCR products. The gradient gel provides finer separation than standard gel electrophoresis, making it advantageous for analyzing complex mixtures of nucleotides.
Another notable application in nucleic acid studies is in the examination of the integrity of RNA samples. This is crucial for reverse transcription and subsequent analysis. Detecting degradation in RNA can influence the outcome of experiments and lead to unreliable results.
Clinical Diagnostics
In clinical diagnostics, gradient gel electrophoresis serves an essential function in identifying and monitoring diseases, notably in hematology and oncology. For example, it is valuable in detecting abnormal hemoglobin variants in blood samples, aiding in the diagnosis of conditions such as sickle cell anemia.
Moreover, this technique is critical for analyzing biomolecules involved in cancer. Gradient gel electrophoresis helps identify specific proteins or gene expression profiles linked to tumor development or progression. This information can contribute to personalized medicine approaches, allowing for tailored treatment plans based on individual biomolecular characteristics.
The integration of gradient gel electrophoresis in clinical applications underscores its relevance in disease management and research.
In summary, the multifaceted applications of gradient gel electrophoresis highlight its importance across diverse fields. By facilitating in-depth analysis of proteins and nucleic acids, it plays a significant role in advancing research and diagnostics, ultimately leading to better health outcomes.
Interpreting Results
Interpreting the results obtained from gradient gel electrophoresis is a crucial phase in the overall process. This step determines how well the electrophoresis was executed and what biological information can be derived from the experiment. Proper analysis of band patterns can reveal the presence, size, and quantity of biomolecules such as proteins or nucleic acids. Thus, a thorough understanding of how to interpret these results is essential for researchers and professionals in molecular biology.
Analyzing band patterns allows scientists to compare samples effectively. Variations in band intensity can signify differences in concentration among biomolecules. Moreover, the distance migrated by a band correlates with the size of the molecule; smaller molecules tend to travel farther than larger ones. However, analyzing band patterns is not simply a matter of observation. Researchers must take care to consider factors such as gel concentration, voltage applied, and the nature of the sample loaded. These factors can influence the clarity and resolution of bands.
Analyzing band patterns can also provide insights into post-translational modifications or the presence of isoforms. For instance, you might observe multiple bands when analyzing a single protein if different charge variants or modifications exist. Observing these nuances requires keen attention and a strong foundational knowledge of the underlying biochemical principles.
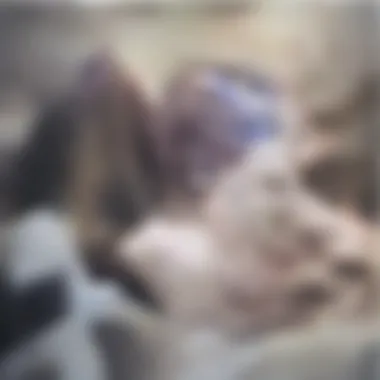
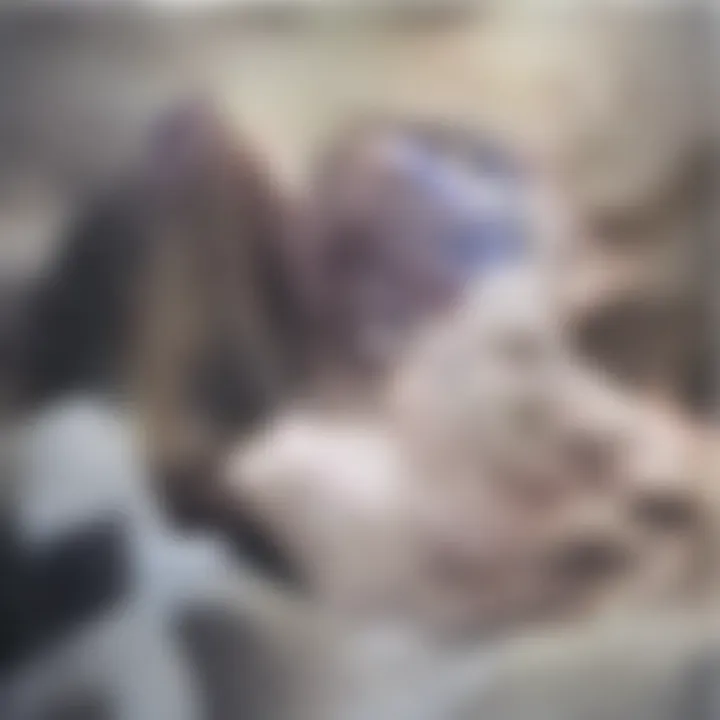
"Understanding the results from electrophoresis goes beyond visual inspection. It involves a careful analysis of patterns, an essential task in the quantitative assessment of biomolecules."
Analyzing Band Patterns
When it comes to analyzing band patterns, identifying the molecular weight markers is important. These markers serve as a reference point for estimating the size of the biomolecules in question. By comparing sample bands to those of known sizes, you can calculate molecular weights accurately. Keep in mind that the quality of the gel can impact visibility; poorly prepared gels might obscure bands, leading to misconceptions about molecular sizes.
- Visual inspection often is not enough; software tools are available that can enhance accuracy. These programs can quantify band intensity and even apply statistical analysis to generate meaningful data about your samples.
- Patterns can suggest the presence of specific post-translational modifications, necessitating further investigation through additional analytical techniques.
Quantification Techniques
Quantification techniques are vital for obtaining precise measurements of biomolecule concentrations from the bands observed in the gel. Techniques may vary from simple visual assessments to advanced imaging systems.
- Densitometry: This is one common method for quantifying bands. By scanning gel images and plotting the density of each band against a calibration curve, you can obtain a quantitative analysis of the biomolecules present.
- Image Analysis Software: More sophisticated approaches involve software that can automatically quantify results, offering added precision that manual methods may lack. These programs can automate corrections for background noise and enhance visibility, leading to more reliable data.
- Comparative Techniques: Utilizing standards or controls alongside samples permits a straightforward relative quantification approach. By comparing experimental results against known standards, researchers can evaluate changes in concentration or expression levels effectively.
- Densitometry software assists in analyzing band intensity, providing numerical data that can be useful for making comparisons based on experimental conditions.
- It is essential, however, to ensure that bands fall within the linear range of the detection system to avoid skewed results.
- ImageJ and Quantity One are examples of such imaging software widely used in laboratories.
In summary, interpreting results forms the backbone of understanding and applying gradient gel electrophoresis. Proper analysis of band patterns and effective quantification techniques lead to valuable insights in various molecular biology applications.
Challenges and Limitations
Understanding the challenges and limitations of gradient gel electrophoresis is crucial for researchers applying this technique. The ability to identify and address these issues can significantly impact the quality of experimental results. Knowledge of these challenges ensures that researchers can optimize their protocols and interpret data with confidence.
Common Experimental Issues
Several experimental issues can arise during gradient gel electrophoresis. Some common problems include:
- Inconsistent Gel Composition: Variability in the concentration of acrylamide can lead to artifacts and inconsistent results across experiments.
- Temperature Fluctuations: The temperature during the run can affect the mobility of biomolecules, making reproducibility difficult.
- Electrode Inefficiency: Poor electrode placement or degradation over time can lead to uneven electric fields, compromising separation quality.
- Sample Overloading: Overloading samples can lead to band distortion, which compromises the clarity of the results and makes interpretation difficult.
- Buffer Issues: Incorrect buffer pH or ionic strength can adversely impact the resolution and effectiveness of separation.
These issues underscore the importance of meticulous protocol optimization and control measures.
Addressing Limitations
To mitigate the challenges associated with gradient gel electrophoresis, researchers should adopt several strategies:
- Standardization of Gel Preparation: Implement consistent protocols for gel preparation to reduce variability in acrylamide concentration. Regular calibration of equipment can also enhance reproducibility.
- Temperature Control: A temperature-controlled electrophoresis chamber can significantly reduce variability caused by temperature fluctuations.
- Regular Maintenance: Routine inspection and maintenance of electrodes can help maintain an effective electric field. This can improve separation performance and consistency.
- Careful Sample Loading: Optimizing sample volumes is critical. Researchers should establish guidelines for sample loading based on the specific concentration and size of the biomolecules analyzed.
- Buffer Optimization: Regularly check and adjust buffer composition to ensure optimal conditions for the run. This guarantees that pH and ionic strength are maintained.
Implementing these strategies not only addresses the common issues faced during gradient gel electrophoresis but also enhances the overall reliability and accuracy of the results obtained. As the field progresses with advancements in technology, these challenges will likely evolve, and ongoing research will be essential to find new solutions.
Future Directions in Gradient Gel Electrophoresis
Gradient gel electrophoresis plays a critical role in molecular biology and biochemistry. As the field evolves, there are notable future directions that promise significant advancements. These directions encompass technological innovations, optimization of methodologies, and expansion of applications. Understanding these trends can influence how researchers utilize this technique in various contexts, enhancing its effectiveness and potential impact.
Technological Advances
Technological progress is a cornerstone in the evolution of gradient gel electrophoresis. Innovations in imaging and detection methods improve both resolution and sensitivity. For example, the integration of advanced fluorescent imaging technologies allows for better visualization of protein bands. This can lead to more precise quantification and improved analysis.
Automation is another area gaining traction. Automated systems for gel preparation and electrophoresis running reduce human error and increase reproducibility. These systems can quickly set up multiple experiments simultaneously, which is especially beneficial in high-throughput environments.
Recent developments in microfluidics create opportunities for smaller, more efficient gel systems. Microfluidic devices can process minuscule samples and provide rapid results, catering to environments where sample quantity is limited. This can enhance the feasibility of gradient gel electrophoresis in clinical and diagnostic applications, where timely results are often crucial.
Potential New Applications
The future also holds promise for new applications of gradient gel electrophoresis across various scientific fields. In pharmacogenomics, for example, this technique could be adapted for analyzing the molecular profiles of individuals in personalized medicine. Gradient gels can help differentiate between subtle variations in protein expression unique to each patient.
In environmental science, the method may evolve to assess biomolecules in complex mixtures from different ecosystems. This can aid in understanding the impacts of pollutants on microbial communities, ultimately contributing to ecological conservation efforts.
Moreover, gradient gel electrophoresis can find applications in the realm of bioengineering. With advances in synthetic biology, it can help analyze proteins developed through engineered pathways, contributing to the creation of novel therapies or biofuels.
In summary, the future of gradient gel electrophoresis is expansive. Through technological advances and new applications, researchers can harness this technique more effectively to address complex challenges in diverse fields.
Overall, keeping pace with these developments not only enhances the understanding of gradient gel electrophoresis but also elevates its role in advancing the life sciences.