Unlocking the Potential of Raspberry Pi Microscopes
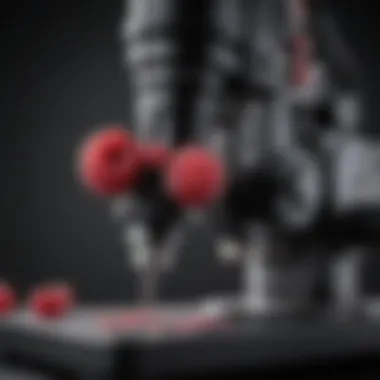
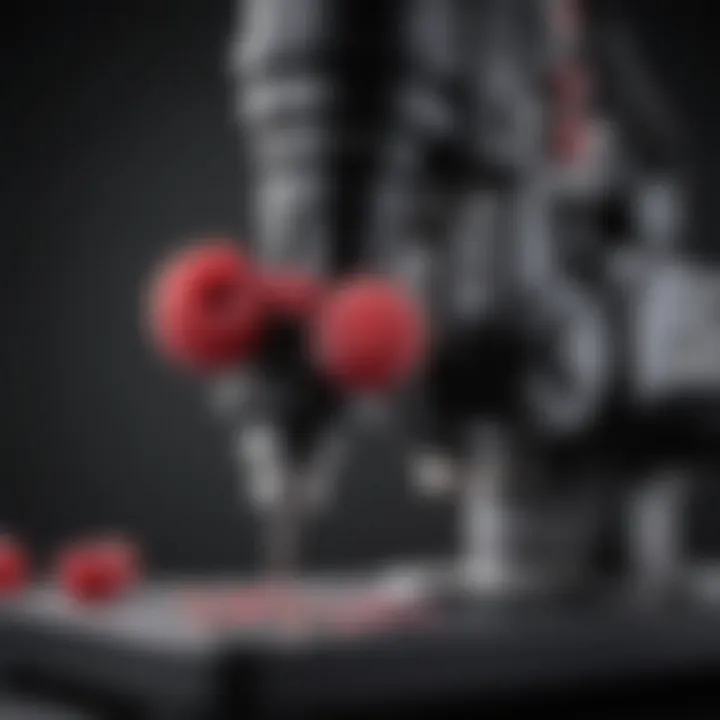
Intro
Raspberry Pi microscopes have become an important aspect of modern research and education. Their accessibility allows students and professionals alike to engage in microscopy without the heavy financial burden often associated with traditional equipment. With the combination of a Raspberry Pi's processing power and various optical components, the result is a versatile and low-cost tool for detailed analysis and observation. In this article, we will explore how to build and utilize these systems, considering the necessary components, software applications, and diverse use cases that showcase their capabilities.
Background and Context
Overview of the Research Topic
The onset of digital microscopy represents a shift in how we visualize the microscopic world. Raspberry Pi microscopes leverage this digital age by combining an easy-to-use microcomputer with optical elements. This innovation allows even modest educational institutions or individual enthusiasts to explore biological specimens, material science, and other fields of study with greater ease and efficiency.
Historical Significance
The historical development of microscopes dates back to the late 16th century, but the integration of digital technology has changed the landscape significantly. Traditional microscopes required expensive equipment and complex optics. The emergence of Raspberry Pi in 2012 opened up new possibilities for hobbyists and educators alike. Utilizing affordable components like USB cameras, lenses, and 3D-printed structures, users can build customized microscopes. This democratization of technology fosters a spirit of experimentation and learning, encouraging more hands-on approaches to science and research.
Key Findings and Discussion
Major Results of the Study
Research on Raspberry Pi microscopes has revealed numerous possibilities for scientific exploration. Users can assemble their own microscopes to achieve magnifications suitable for various applications. The combination of affordable and modular parts allows for tailored experiences, from basic educational functions to intricate research settings. Data gathered indicates the effectiveness of these microscopes in classrooms and labs, where engagement and interest spike due to hands-on projects.
Detailed Analysis of Findings
- Components Required: Building a Raspberry Pi microscope involves selecting components wisely. The following are essential:
- Software Applications: Various software tools facilitate image capture and analysis. Some notable ones include:
- Applications Across Disciplines: The versatility of these microscopes allows utilization in multiple fields:
- Raspberry Pi board (e.g., Raspberry Pi 4 Model B)
- High-resolution camera (like the Raspberry Pi Camera Module)
- Objective lenses and eyepiece lenses
- Sturdy base, preferably built from durable materials or 3D-printed models
- Pi Camera Software: Enables image and video capturing directly from the camera module.
- OpenCV: Useful for image processing and enhancing the output images.
- Biology: Observation of cellular structures.
- Materials Science: Analysis of materials on a microscopic level.
- Education: Foster students' interest in science with hands-on learning.
"The adoption of Raspberry Pi microscopes in educational settings has demonstrated significant improvements in student engagement and understanding in science."
The integration of advanced imaging and processing capabilities open doors for innovative application and research endeavors. It serves as a foundational step for further exploration in microscopy and related fields.
By understanding the significance of Raspberry Pi microscopes, users can immerse themselves in a growing community. As technologies advance and more resources become available, the potential applications for Raspberry Pi-based microscopes are limitless. This article hopes to provide insights that empower users to construct, utilize, and innovate with Raspberry Pi microscopes.
Prelims to Raspberry Pi Microscopes
In recent years, the marriage of traditional microscopy and modern computing platforms has opened up exciting possibilities for researchers and hobbyists alike. At the forefront of this trend is the Raspberry Pi microscope, a tool that revolutionizes how we observe and analyze small subjects. This topic is not just interesting; it holds significant importance for anyone looking to engage with microscopy without the prohibitive costs associated with professional-grade equipment.
Significance of Microscopy
Microscopy is an essential technique in many scientific fields, including biology, material science, and environmental studies. By allowing us to see tiny structures and organisms, it expands our understanding of the world at a microscopic level. This capability becomes vital, especially in educational settings, where students can engage with scientific principles directly. Microscopes can facilitate practical learning by enabling hands-on experiences with specimens that are otherwise invisible to the naked eye.
With Raspberry Pi-based microscopes, education becomes more accessible. They offer a cost-effective solution that does not compromise on quality. The ability to customize the microscope according to specific research needs further enhances its utility. A Raspberry Pi microscope can serve multiple purposes, from examining microbial life to analyzing the properties of materials.
Overview of Raspberry Pi as a Platform
The Raspberry Pi is a credit-card-sized computer that has gained immense popularity among tech enthusiasts, educators, and researchers. Its affordability, versatility, and ease of use make it an attractive choice for projects that require computational power. The small form factor and low energy consumption enable it to be integrated into various applications, including microscopy.
As a platform, the Raspberry Pi supports different operating systems, primarily Linux-based, which can enhance its functionality in microscopy projects. The available GPIO (General Purpose Input/Output) pins allow users to integrate sensors and other electronic components easily. Thus, it is feasible to expand the microscope's capabilities beyond simple image capture, enabling data logging and analysis through additional sensors. This aspect makes the Raspberry Pi an ideal choice for those who want to explore and experiment with new ideas in microscopy.
"Raspberry Pi microscopes exemplify the democratization of science, making advanced tools available to a broader audience."
Overall, the field of Raspberry Pi microscopy bridges the gap between traditional scientific exploration and modern technology, empowering users to conduct their own research and experiments without significant financial investment. It positions itself as a meaningful innovation within the scientific community.
Fundamentals of Microscopy
Understanding the fundamentals of microscopy is essential when constructing a Raspberry Pi microscope. Microscopy serves as a cornerstone for biological research, material analysis, and monitoring environmental changes. The techniques and technologies associated with microscopy not only illuminate the unseen worlds but also allow researchers to make informed conclusions based on observed data. When integrating a Raspberry Pi into microscopy, grasping the foundational principles ensures that users can effectively optimize the options available.
Types of Microscopes
Microscopes can be categorized based on their operational principles and applications. Here are some of the more common types:
- Optical Microscopes: Utilize visible light and standard lenses. They are the most widely used and accessible for basic studies in educational settings.
- Digital Microscopes: Feature built-in cameras, allowing for immediate capture and viewing of images on connected screens. These microscopes often utilize a USB or HDMI connection for easy access to image data.
- Electron Microscopes: Use electron beams instead of light, providing a significantly higher resolution. They are useful for observing small structures such as viruses or cellular components but are generally more costly and complex.
- Fluorescence Microscopes: Employ fluorescent dyes to highlight specific parts of a sample. This type is especially helpful in biological studies, where particular cellular structures can be tagged and observed.
- Confocal Microscopes: Focus light on a single point to eliminate out-of-focus light. This approach allows for 3D visualization of specimens, making it ideal for advanced studies requiring detailed imaging.
Principles of Optical Microscopy

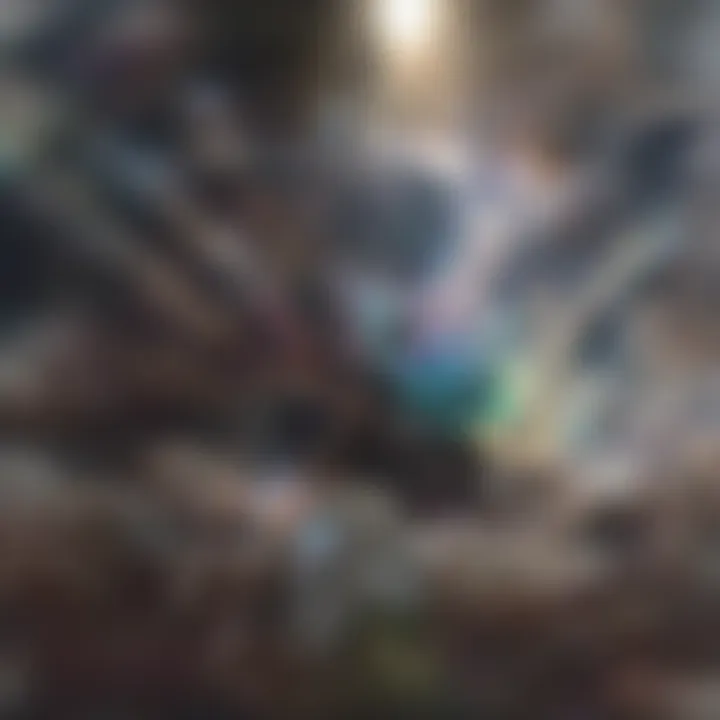
Optical microscopy forms the basis for many Raspberry Pi microscopy projects. The principle relies on visible light being transmitted through or reflected from a sample, which is then magnified by a series of lenses. Key aspects include:
- Light Source: Most optical microscopes use a light source, either transmitted or reflected light, to illuminate the sample. Common options include LED lights or traditional bulb-based illumination.
- Lenses: Convex lenses bend light rays to magnify the viewed object. The quality and type of lenses greatly affect the resulting image quality, leading to a clear and coherent visualization of microscopic structures.
- Resolution: This refers to the ability to distinguish between two closely spaced points. Achieving higher resolution depends on the wavelength of light used and the numerical aperture of the lenses.
- Focus Techniques: Adjusting focus is crucial for achieving clear images. Users may need to manipulate focusing knobs to refine the clarity of the specimen being observed.
In a Raspberry Pi microscope, understanding these principles allows for better decisions regarding component selection, assembly techniques, and software applications for image processing. This knowledge directly informs the overall performance and capability of the end product.
"The development of microscopy has revolutionized our capacity to see and study the microscopic world, allowing breakthroughs across various scientific fields."
By combining the versatility of Raspberry Pi systems with the foundational principles of microscopy, users can create effective and efficient microscopical tools for research and exploration.
Essential Components of a Raspberry Pi Microscope
Building a Raspberry Pi microscope involves several key components that are vital for effective performance. Each part plays a critical role in the functionality, providing the necessary features required for microscopy work. Understanding these components helps in making informed decisions during construction, ensuring the microscope meets specific needs and standards.
Choosing the Right Raspberry Pi Model
Selecting the appropriate Raspberry Pi model is foundational to constructing a reliable microscope. Different models, such as the Raspberry Pi 4, Raspberry Pi 3, and Raspberry Pi Zero, offer varied processing power, memory, and connectivity options. The Raspberry Pi 4 stands out due to its quad-core CPU and options for up to 8GB RAM, which is advantageous for handling complex image data and multiple applications simultaneously.
One must consider the intended use when choosing a model. For basic applications, a Raspberry Pi Zero may suffice. However, for more demanding tasks, a Raspberry Pi 4 provides substantial benefits. Connectivity options are also critical, particularly USB ports for external cameras and other peripherals.
Camera Options and Specifications
Camera quality heavily influences the results achieved with a Raspberry Pi microscope. The choice of camera should align with the desired magnification and level of detail. Popular options include the Raspberry Pi Camera Module V2 and more advanced USB cameras. The Camera Module V2 features an 8-megapixel sensor capable of capturing high-definition images, making it ideal for educational and research purposes.
When selecting a camera, it is essential to consider parameters such as resolution, frame rate, and sensitivity to light. An appropriate camera not only enhances the clarity of images but also improves the overall microscopy experience. Additionally, compatibility with the Raspberry Pi and available software should guide the selection process.
Optical Lenses and Their Impact
Optical lenses are crucial for achieving the desired magnification and clarity in microscopy. The choice of lenses directly affects the quality of the images captured. Common types of optical lenses used in Raspberry Pi microscopes include objective lenses and eyepiece lenses. Objective lenses determine the primary magnification level, while eyepiece lenses facilitate viewing and can add additional magnification.
Different lenses also provide unique fields of view and depth of focus. When selecting lenses, one should consider factors such as focal length and lens quality. High-quality lenses might incur higher costs but can significantly improve image resolution and detail. Moreover, combining different lenses can enable varying levels of zoom and clarity, making the microscope versatile for various applications.
"The lens quality can make or break your microscopy experience; invest wisely."
In summary, understanding the essential components of a Raspberry Pi microscope is key to ensuring effective operation and results. Each element, from the Raspberry Pi model to camera specifications and optical lenses, contributes heavily to the overall functionality and quality of the microscope.
Assembly Process of the Raspberry Pi Microscope
The assembly process of a Raspberry Pi microscope is a significant topic in this article as it transforms components into a functional device. Building the microscope from scratch allows users to understand the mechanics behind it. This process also provides an opportunity to customize the design based on specific needs or scientific applications. The benefits include cost-effectiveness, enhanced understanding of the technology, and the ability to troubleshoot and maintain the device better than pre-assembled models. Considering the assembly process helps ensure that users are prepared for any challenges and fully equipped to utilize the microscope efficiently.
Required Tools and Materials
When preparing to assemble a Raspberry Pi microscope, gathering the right tools and materials is essential. Below is a list of necessary items to ensure a smooth building experience:
- Raspberry Pi Board: Preferably a Raspberry Pi 4 for better performance.
- Camera Module: A compatible camera, such as the Raspberry Pi Camera Module V2, is recommended for better image quality.
- Optical Lenses: Various magnification lenses to adjust the viewing capability of the microscope.
- Structural Frame: This could be made from wood, plastic, or metal, depending on what is available and desired for stability.
- Lighting Source: An LED light panel or lamp to illuminate specimens will enhance the viewing experience.
- USB Cables: Needed for connecting the camera and the Raspberry Pi.
- Screwdriver Set: Essential for assembling the frame and securing components in place.
- Hot Glue Gun: Useful for securing parts without the need for screws in some cases.
- Computer with Internet Access: For software installation and troubleshooting.
Before starting, review all materials to ensure everything required is on hand. This will save time and frustration during the assembly process.
Step-by-Step Assembly Guide
Assembling a Raspberry Pi microscope involves several steps, which require careful attention to detail. Hereโs a straightforward guide to assist through the building process:
- Prepare the Workspace: Ensure a clean, well-lit area to work in. Having ample space will help keep tools and parts organized.
- Build the Frame: Start by constructing the frame that will hold the camera, optical lenses, and the specimen. Securely attach the pieces using screws or hot glue.
- Attach the Lenses: Fix the optical lenses onto the designated area of the frame. Ensure they align correctly with the camera module for optimal viewing.
- Install the Camera: Connect the Raspberry Pi camera to the board. Follow the instructions to ensure proper installation.
- Add Lighting: Position the lighting source to adequately illuminate the specimen area. Adjust the light's angle as necessary.
- Connect the Raspberry Pi: Plug in the power supply and USB cables needed for the camera. Make sure all connections are secure.
- Power On the Unit: Once everything is connected, power on the Raspberry Pi and check that the camera functions correctly by accessing the camera module interface.
- Final Adjustments: Make any necessary adjustments to the focus and lighting before performing the first capture.
The assembly process serves as a practical introduction to functional microscopy. By engaging in this hands-on activity, users enhance their competencies in technology and science.
Software Setup and Image Acquisition
The importance of Software Setup and Image Acquisition in the construction and use of Raspberry Pi microscopes cannot be overstated. These elements form the backbone of functionality for any microscope project. A good understanding of software helps in effectively managing the hardware components and allows the user to capture, process, and analyze images efficiently. Furthermore, appropriate software setups enable enhancements in image quality and usability, which are critical for serious scientific inquiries.
Installing Operating System and Libraries
To begin with, selecting the right operating system is crucial. Raspberry Pi users often opt for Raspbian, a Debian-based OS optimized for the Raspberry Pi's hardware. Installing Raspbian is straightforward. Users can download the latest image from the official Raspberry Pi website and flash it onto a microSD card using software like Balena Etcher. Once the OS is installed, it's essential to update the system using the terminal commands:
After the basic OS installation, additional libraries specific to image processing and camera handling are required. Libraries like PiCamera and OpenCV are extremely beneficial. The PiCamera library provides simple ways to control the camera on the Raspberry Pi while OpenCV offers advanced image processing capabilities.
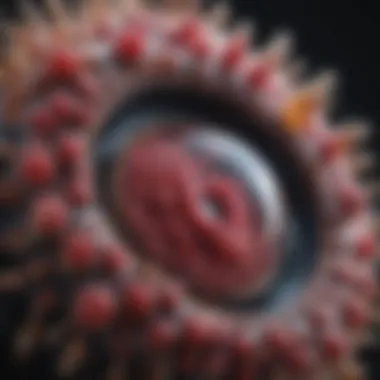
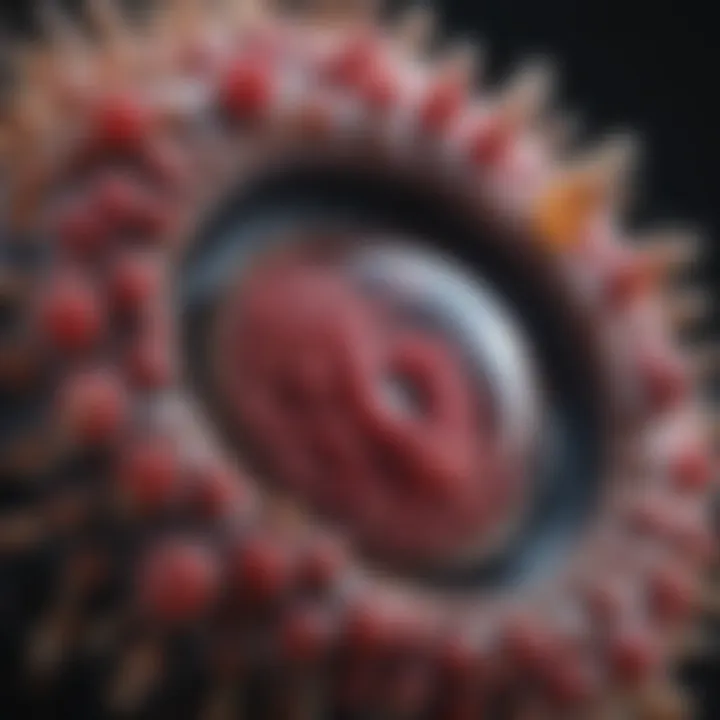
To install these libraries, you can use the following commands:
With the correct libraries installed, the software environment is ready for the next step.
Programming Image Capture Techniques
Once the operating system and libraries are in place, programming image capture techniques is vital for extracting high-quality images from your Raspberry Pi microscope. Python is often the programming language of choice due to its readability and the extensive support community around the Raspberry Pi.
Using the PiCamera module, users can write scripts to capture images based on various settings such as resolution, brightness, and contrast. A sample script to capture images might look like this:
Enhancements can be made by introducing time-lapse functionality or automating captures based on specific triggers, such as light detection. Experimenting with different capture techniques can significantly improve the resulting image quality and functionality of the microscope.
"The blend of hardware and software innovation is at the heart of modern microscopy with Raspberry Pi, enabling low-cost solutions for high-quality scientific investigation."
By understanding these processes, students, researchers, and hobbyists can make significant strides in their projects.
Enhancing Image Quality
Enhancing image quality is a crucial aspect when working with Raspberry Pi microscopes. The effectiveness of this low-cost technology hinges on its ability to produce clear, detailed images. As researchers and enthusiasts strive to obtain finer details in their observations, understanding how to enhance image quality becomes imperative. Significant improvements can be achieved through proper calibration of optical components and the integration of image processing software.
Calibration of Optical Components
Calibration of optical components is essential for ensuring that the microscope operates at its best. When the lenses and sensors are properly aligned, it leads to sharper images, reducing distortion and improving overall visual clarity. Calibration involves adjusting the focus, correcting lens aberrations, and ensuring that the camera sensor accurately captures light from the specimen.
Here are some key steps and considerations in the calibration process:
- Alignment of Lenses: Proper orientation of the optical lenses is vital. Misalignment can lead to blurred images and can degrade the quality significantly. Regular checks can maintain optimal positioning.
- Focus Adjustment: Getting the right focal point is crucial. This often requires fine adjustments to capture images in sharp detail.
- Testing with Calibration Slides: Utilizing standard calibration slides helps in evaluating the quality of the image produced. It allows users to identify areas needing improvement before moving to more complex specimens.
- Regular Maintenance: Keeping the optical components clean and dust-free contributes greatly to maintaining high image quality. Even a small amount of dirt can obscure delicate details.
By systematically addressing these factors, users can significantly enhance the effectiveness of their Raspberry Pi microscopes, ensuring accurate representation of microscopic details.
Use of Image Processing Software
Image processing software plays a pivotal role in further enhancing the quality of images captured by Raspberry Pi microscopes. In many cases, the raw images obtained may not fully represent the details present in the specimen. Image processing techniques can help rectify this, providing clearer visuals that assist in research and analysis.
Several software options are accessible for users, from basic applications to advanced platforms.
Benefits of using image processing software include:
- Noise Reduction: Many images may contain unwanted noise. Software helps to smooth these imperfections, making the features of the specimen more pronounced.
- Contrast Enhancement: Adjusting contrast can help differentiate between various elements in the image. This is especially helpful when working with samples that may appear similar at first glance.
- Measurement Tools: Certain software packages offer in-built tools for measuring distances or areas within the images. This is particularly useful for quantitative studies in fields like biology and materials science.
- Image Stacking: This technique involves combining multiple images taken at different focal points. The result is a single image that has a greater depth of field, further highlighting fine details.
By integrating effective image processing software into their workflow, users can maximize the capabilities of their Raspberry Pi microscopes and achieve superior image quality, paving the way for more insightful analyses in various fields.
Applications in Scientific Research
The integration of Raspberry Pi microscopes in scientific research has opened up numerous avenues for exploration and discovery. These tools provide high adaptability and accessibility, which can significantly enhance research capabilities across various disciplines. Their affordability democratizes access to microscopy, allowing not just established labs but also educational institutions and individual researchers to engage in scientific inquiry. This section delves into specific applications within biology, materials science, and environmental monitoring, emphasizing the innovative uses of Raspberry Pi microscopes.
Biology and Microbiology Studies
Raspberry Pi microscopes have become invaluable in biology and microbiology research. They facilitate the study of cellular structures, microorganisms, and biological processes through high-resolution imaging. One significant advantage is the ease of capturing live samples, which can lead to more dynamic research outcomes.
For instance, in microbiology, researchers can observe bacterial growth patterns and behaviors in real-time. With the right software, they can automate image capture at intervals, allowing for time-lapse studies. This capability can be crucial when examining interactions between different microbial species or observing responses to environmental changes.
Moreover, educational platforms can utilize these microscopes to teach students about microbiology hands-on. This practical engagement cultivates a deeper understanding of complex biological concepts, making research more relatable and accessible.
"The use of Raspberry Pi in biological research not only enhances learning but also broadens research opportunities for those with limited resources."
Materials Science and Analysis
In materials science, Raspberry Pi microscopes serve as tools for analyzing the properties and behaviors of various materials at microscopic levels. They are particularly useful in examining the microstructures of metals, polymers, and composites. Researchers in this field benefit from the ability to assess characteristics such as grain structures, surface defects, and layer thicknesses with precision.
Using Raspberry Pi technology, scientists can document and analyze changes in materials due to stress, temperature variations, or chemical exposure. This capability is crucial for developing new materials or understanding the failure mechanisms in existing ones. Furthermore, the ability to manipulate the imaging process through programming allows for customized experiments tailored to specific research needs.
Environmental Monitoring and Analysis
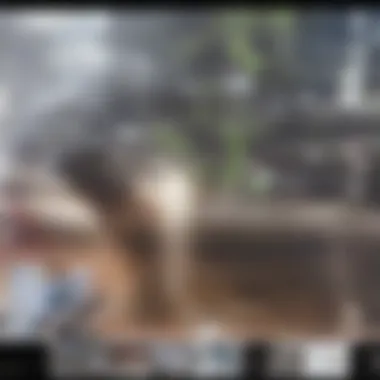
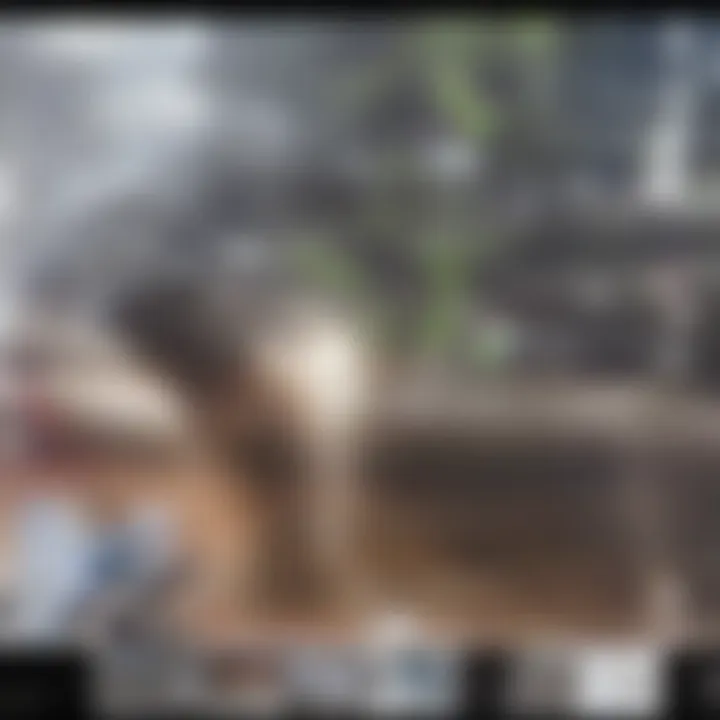
Environmental scientists utilize Raspberry Pi microscopes to conduct insightful analyses of biological and chemical phenomena in ecosystems. These microscopes can assist in monitoring water quality by examining microbial communities or pollutants in various water bodies. Through detailed imaging, researchers can analyze algae blooms or other indicators of ecological health.
Moreover, collaborations between computer scientists and environmental researchers are paving the way for the development of automated monitoring systems. Such systems can continuously capture data, analyze samples, and detect changes in environmental conditions over time. This ongoing observation leads to a robust dataset that researchers can use to draw meaningful conclusions about environmental trends and impacts.
In summary, the applications of Raspberry Pi microscopes in scientific research cover diverse fields, from microbiology to materials science and environmental monitoring. Their integration into research practices not only facilitates innovative studies but also fosters an environment where scientific exploration is within reach for many.
Limitations and Challenges
Understanding the limitations and challenges of using Raspberry Pi microscopes is crucial for both prospective builders and current users. While the Raspberry Pi platform offers an accessible way to create low-cost microscopes, certain factors can restrict their efficacy and usability in various applications. This section discusses the core technical constraints and compares the image quality with that of professional-grade microscopes, providing insight into what users can realistically achieve.
Technical Constraints of the Raspberry Pi
The Raspberry Pi, although versatile, does come with inherent technical constraints. One significant limitation is its processing power. While new models, like the Raspberry Pi 4, boast improved performance, the CPU may still struggle with intensive image processing tasks. When utilizing high-resolution camera modules, the processing demands can lead to latency issues or stuttering during image acquisition.
Another aspect to consider is memory capacity. The Raspberry Pi has limited RAM, which can pose challenges when running multiple applications or using memory-intensive image analysis software. Users may find that optimizing system settings or managing resources is essential for maintaining smooth operation.
Additionally, the Raspberry Pi does not natively support certain features common in professional microscopes. For instance, many advanced microscopes come equipped with specialized software for image enhancement and analysis that Raspberry Pi setups may lack, necessitating additional programming and setup.
Image Quality Comparisons with Professional Microscopes
Image quality is often the first point of reference for those assessing the capabilities of Raspberry Pi microscopes. In general, while DIY microscopes can achieve satisfactory results, they typically cannot match the quality produced by professional microscopes. Key differences include:
- Optical Systems: Professional microscopes utilize high-quality lenses designed for specific applications, allowing for superior clarity and resolution. In contrast, Raspberry Pi setups usually rely on off-the-shelf components that may not offer the same optical precision.
- Lighting Control: Advanced microscopes provide comprehensive lighting systems capable of producing variable intensity and direction, enhancing image quality. Raspberry Pi users may need to experiment with external lighting solutions, which can be less effective.
- Image Processing: Professional systems often come with integrated image processing capabilities, resulting in enhanced performance in terms of contrast, color correction, and detail retrieval. Raspberry Pi users may need extensive coding experience to replicate these features.
"While Raspberry Pi microscopes are defined by their accessibility and adaptability, they are hindered by limitations that prevent them from reaching the image quality of dedicated professional microscopes."
In summary, the Raspberry Pi microscope demonstrates potential for educational and hobbyist applications, though its limitations should not be underestimated. For those engaged in serious research, recognizing these constraints is key to setting appropriate expectations and exploring alternative solutions.
Future Developments in Raspberry Pi Microscopy
The realm of Raspberry Pi microscopy is on the brink of significant advancements. As technology evolves, so too does the potential for these low-cost microscopes to be more potent and accessible. Future developments will likely center around enhancements in camera technology and the integration of artificial intelligence for image analysis. These elements are crucial to improving the overall performance and usability of Raspberry Pi-based microscopes.
Advancements in Camera Technology
The development of camera technology is vital for enhancing the ability of Raspberry Pi microscopes. Recent innovations in camera sensors are leading to improved resolution, dynamic range, and sensitivity. For instance, the introduction of high-resolution sensors allows for capturing finer details in microscopy images.
Moreover, advancements like global shutter technology can reduce motion blur, ensuring that rapid movements are well-captured without distortion. This is particularly useful in biological studies where samples may be dynamic or in motion.
As the prices of high-quality imaging sensors decrease, they become more accessible for integration into Raspberry Pi projects. Researchers can expect to see a wider variety of compatible cameras that offer enhanced functionality.
- Improved resolution will enable capturing tiny details in specimens.
- Better sensitivity can help in low-light scenarios, crucial for fluorescence imaging.
- Enhanced color accuracy will lead to more realistic representations of samples.
In summary, these technological improvements promise to elevate the capabilities of Raspberry Pi microscopes significantly, making them even more viable tools for research and education.
Integrating Artificial Intelligence for Image Analysis
Another promising development in the future of Raspberry Pi microscopy lies in the integration of artificial intelligence. AI algorithms can process vast amounts of data rapidly and provide insights that were previously difficult to obtain.
Using machine learning, Raspberry Pi microscopes can automatically identify and classify specimens based on learned patterns from training datasets. This capability can streamline the analysis process, allowing researchers to focus on interpretation rather than processing raw data.
AI can also enhance image quality through techniques such as super-resolution, where algorithms reconstruct a higher-quality image from several lower-quality ones. This is particularly advantageous for amateur microscopists who may not have access to professional-grade imaging equipment.
Here are several benefits of integrating AI:
- Automated analysis: Reduces the time spent on data processing.
- Enhanced accuracy: Improves reliability in identifying samples.
- Predictive analysis: Provides forecasts based on observed data trends.
End
In the landscape of scientific exploration, Raspberry Pi microscopes represent an innovative convergence of accessibility and precision. They offer educational advantages while facilitating intricate research across a variety of disciplines. The relevance of the topic extends beyond just understanding how to assemble these devices; it speaks to the broader implications of enabling scientific inquiry at all levels. The cost-effectiveness and flexibility of Raspberry Pi technology empower individuals, educators, and researchers, democratizing access to microscopy.
Summary of Key Points Discussed
This article covered several key topics that highlight the importance and utility of Raspberry Pi microscopes:
- Foundation of Microscopy: Understanding the core principles and types of microscopy aids in realizing the capabilities of Raspberry Pi systems.
- Essential Components: Choosing the right Raspberry Pi model, camera, and optical lenses directly affects performance and image quality.
- Assembly Process: A detailed guide facilitates the construction of a functional microscope, emphasizing the importance of meticulous assembly.
- Software Setup: Proper software configuration is critical for image capture and analysis; mastering this can significantly enhance research outcomes.
- Applications: The versatility of these microscopes spans various fields, including biology, materials science, and environmental monitoring, underscoring their relevance in contemporary scientific research.
- Limitations and Future Directions: Knowing the constraints helps in managing expectations while advancements in technology promise novel opportunities for improvement.
Call to Action for Researchers and Hobbyists
For students, researchers, and hobbyists looking to dive into the world of microscopy, engaging with Raspberry Pi microscopes offers a practical platform for exploration. Start your journey with:
- Experimenting: Assemble your microscope and adjust components to see how different setups affect image quality.
- Learning: Utilize online resources and community forums on platforms like Reddit to share experiences and gain insights.
- Sharing Knowledge: Document your findings and methodologies to contribute to the growing body of knowledge, helping others navigate the complexities of microscopy.
- Innovating: Challenge yourself to integrate new technologies, such as artificial intelligence, into your Raspberry Pi setup. Explore how machine learning can enhance image analysis.
Embrace the possibilities that Raspberry Pi microscopes offer. Your discoveries could contribute significantly to your field and inspire others in their scientific pursuits.