Comprehensive Overview of the PDB Database
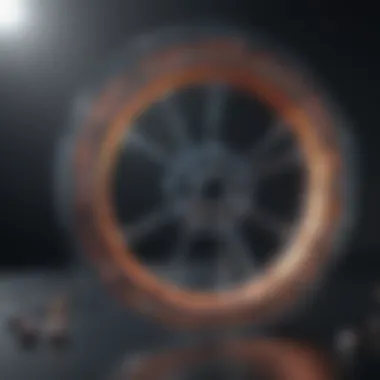
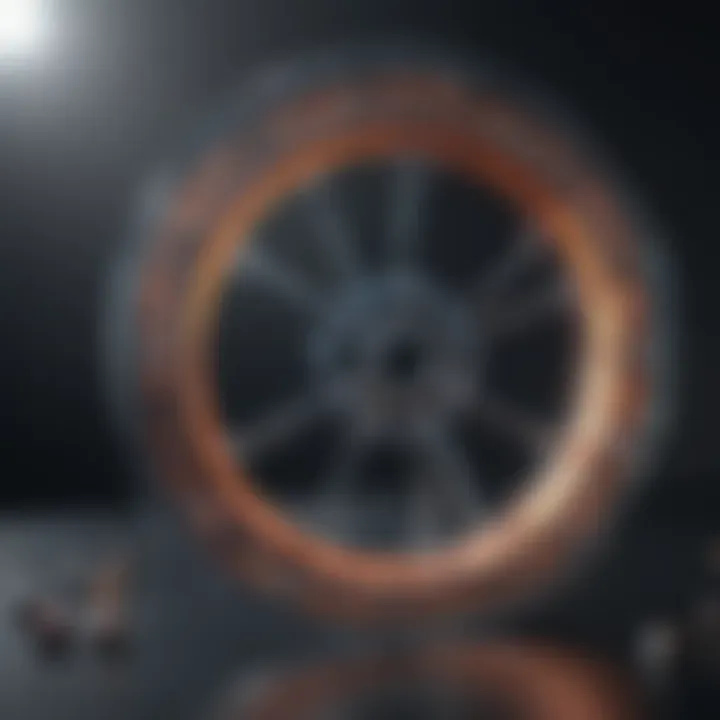
Intro
The Protein Data Bank (PDB) is a crucial resource in the field of structural biology, serving as a repository for three-dimensional structural data of biological macromolecules. Understanding how proteins and nucleic acids interact at a molecular level is vital for many scientific fields, including biochemistry and pharmacology. This article aims to provide a detailed exploration of the PDB, discussing its structure, significance, and the substantial impact it has had on scientific research and advancements.
Background and Context
Overview of the research topic
The PDB was established to address the growing need for accessible structural data on proteins and nucleic acids. It consolidates experimental data and provides a framework for sharing this information globally among researchers. This vast database is not simply a collection of data; it's a cornerstone for understanding life sciences and developing new therapeutic agents.
Historical significance
Founded in 1971, the PDB began as a small archive with only 7 entries, but it has grown exponentially over the decades. Its continual evolution parallels advances in imaging technologies like X-ray crystallography and nuclear magnetic resonance (NMR) spectroscopy. These techniques provide the detailed information needed for accurate structural representations. The importance of the PDB extends beyond mere data collection; it reflects the dynamic relationship between technological innovations and scientific discoveries in biology.
Key Findings and Discussion
Major results of the study
The PDB is essential for various applications, notably in drug design and development. Researchers can study protein-ligand interactions by analyzing structural data available in the PDB.
- Drug design: The structural information can guide scientists in designing molecules that effectively target specific sites in proteins.
- Protein engineering: Engineers rely on PDB data to modify protein structures to improve their functions or create novel enzymes.
- Computational biology: Many computational techniques, such as molecular modeling and simulations, require structural data from the PDB for validation and accuracy of results.
Detailed analysis of findings
Through detailed analysis of data from the PDB, researchers can elucidate intricate biological mechanisms. The importance of PDB in advancing our understanding of disease mechanisms cannot be overstated. For instance, by comparing the structures of healthy and diseased proteins, critical insights into the pathology can emerge.
Moreover, the ease of access to PDB data facilitates collaborative research across disciplines. The database fosters an environment conducive to innovation and discovery, as data is shared among biologists, chemists, and pharmacologists.
"The PDB serves as a bridge connecting diverse fields of study, highlighting its critical role in the advancement of biomedicine and biotechnology."
Prelims to the PDB Database
The Protein Data Bank (PDB) serves as an indispensable resource for the scientific community, particularly in the field of structural biology. Understanding its foundations and relevance is crucial to appreciating how it enhances research and advancements in various biotechnological applications. The PDB provides a comprehensive repository of three-dimensional structural data for proteins, nucleic acids, and complex assemblies, all derived from experimental techniques. This section will delve into its historical context and clarify what the PDB specifically entails.
Historical Background
The origins of the PDB can be traced back to the early 1970s when the first crystal structure of a protein, myoglobin, was resolved. This landmark achievement showcased the value of structural biology and necessitated a centralized database for storing such vital information. In 1971, the PDB was officially established, initially hosted at the Brookhaven National Laboratory in the United States. Over the years, technological advancements and the increasing number of structures determined have prompted several updates and transitions. Today, the PDB is maintained by a consortium of institutions, including the Research Collaboratory for Structural Bioinformatics. Its evolution has mirrored advancements in crystallography, NMR spectroscopy, and other structural determination methods, making it more influential within the scientific community.
What is the PDB?
The PDB is an archive that systematically organizes three-dimensional structures of biological macromolecules. It contains a wealth of information provided by researchers from all over the globe, thus fostering collaboration and exchange within the field. Each structure in the PDB is represented by a unique identifier, known as the PDB ID, which facilitates easy referencing. The database not only houses coordinate data but also includes annotations regarding ligand interactions, binding sites, and functional insights. It plays a pivotal role in various scientific disciplines, including drug design, molecular biology, and biochemistry.
Researchers utilize the PDB to visualize and analyze structures, leading to informed decisions in their projects. It is not merely a collection of data; it is a dynamic resource that enhances scientific inquiry. Furthermore, as accessibility and usability have improved over time, the PDB continues to be a cornerstone for understanding the molecular underpinnings of life.
Structure and Organization of the PDB Database
The structure and organization of the Protein Data Bank (PDB) database are fundamental to its utility and effectiveness in the realm of structural biology. A coherent data structure allows researchers to efficiently query and retrieve information regarding protein and nucleic acid structures. This section will delve into the intricacies of how the data is organized, the various formats it utilizes, and the implications of these structures on scientific research.
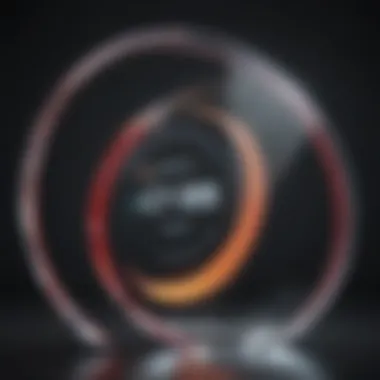
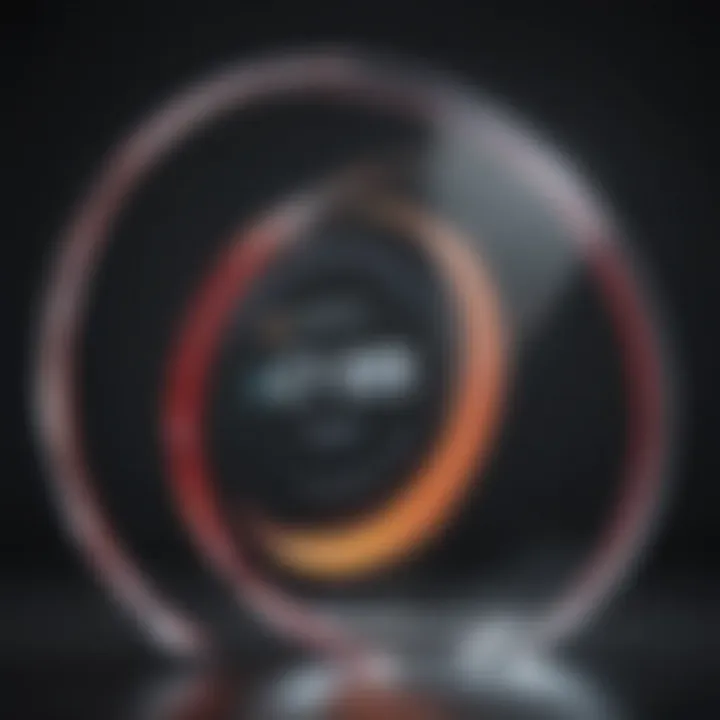
Data Structure
The PDB is designed to manage and present a vast quantity of structural information. This is achieved through a carefully outlined data structure that defines how each entry appears in the database. The data structure encompasses several components, such as metadata associated with each structural entry, including the protein's name, its source organism, experimental method used for structure determination, and structural resolution.
Each entry is identified by a unique four-character identifier. This identifier is essential for referencing and retrieving data efficiently. The structure of the PDB file comprises a series of standardized records or sections, which contain the coordinates of atoms, connectivity information relating to residues, and additional details about the structure. This systematic organization ensures that all necessary information is readily accessible, facilitating analyses ranging from basic educational use to advanced research applications.
Furthermore, the organization of data within the PDB allows for a seamless integration with various computational tools. This offers researchers an opportunity to visualize structures in three dimensions or perform quantitative analyses through programming languages like Python and R. Overall, a well-defined data structure is pivotal for enhancing usability and access.
File Formats
The PDB database employs several file formats, which play a significant role in how data is stored and shared. The most recognized format is the PDB file format (.pdb). This text file format is straightforward, consisting of longitudinal records that list atomic coordinates along with necessary details about each structure. The readability of this format is one of its primary advantages, making it accessible even to those who may not possess advanced computational skills.
Another format utilized by the PDB is the mmCIF (macromolecular Crystallographic Information File). This format represents a more modern approach, allowing a richer set of data fields while also maintaining a structured hierarchy. mmCIF is specifically designed to accommodate larger data sets, which have become prevalent as structures become more complex and detailed.
Moreover, there are additional derived formats like the PDBx/mmCIF format, which extends the capabilities of the mmCIF format for better integration with other databases and software. These various formats offer distinct benefits related to data transfer, storage efficiency, and compatibility with different computational tools.
In terms of practical use, researchers can choose the format that best serves their needs. Good practices dictate that when sharing data or accessing the database, users should be aware of these formats to ensure proper usage and application.
In summary, the structure and organization of the PDB database is crucial. It influences not only how information is stored but also how effectively it can be utilized in research and education.
Data Collection and Submission
Data collection and submission form the backbone of the Protein Data Bank (PDB). These processes ensure that data derived from various techniques is accurately recorded and made available for research. The importance of this topic cannot be understated; the quality of the database depends heavily on how data is collected, verified, and submitted by researchers from all over the world. Good data practices enhance the reliability and usability of the database for scientists, educators, and students alike.
Techniques for Structure Determination
Understanding different techniques is crucial for appreciating what the PDB offers. Each method has its distinct characteristics, advantages, and disadvantages, influencing the structural data generated.
X-ray Crystallography
X-ray crystallography stands out as a primary technique for determining the 3D structures of biomolecules. It is widely recognized for its high resolution, making it possible to visualize structures at an atomic level. This high resolution is one of its most significant contributions to structural biology. The method involves crystallizing a protein and then bombarding it with X-rays, which creates a diffraction pattern that can be analyzed to determine the structure.
One key feature of X-ray crystallography is its ability to provide detailed information about the arrangement of atoms within a crystal. This level of detail makes it a preferred method for many researchers aiming to elucidate intricate molecular interactions. However, there are drawbacks. Not all proteins can be easily crystallized, which can limit the applicability of this technique.
Nuclear Magnetic Resonance
Nuclear Magnetic Resonance (NMR) spectroscopy offers an alternative to X-ray crystallography, especially for proteins in solution. This technique allows for the study of proteins in a more natural state. A key characteristic of NMR is its ability to provide dynamic information about molecular structures. This means researchers can observe how proteins change shape or interact over time, which is essential for understanding their functions.
While NMR is beneficial for smaller proteins, it has limitations regarding the size of the biomolecules it can analyze effectively. Larger complexes can present challenges due to signal limitations and increased complexity in the data analysis process.
Electron Microscopy
Electron microscopy is another significant technique for structural determination. It allows for imaging of biomolecules at high resolutions, making it possible to visualize large complexes and assemblies. A critical aspect of electron microscopy is its capability to analyze non-crystalline samples, addressing a limitation present in X-ray crystallography. This is particularly advantageous for large proteins that do not form crystals easily.
However, the technique also has some shortcomings. The sample preparation can be intricate and time-consuming. Additionally, interpreting the resulting images often requires sophisticated computational algorithms to reconstruct the 3D structure accurately.
Submission Process for Researchers
The submission process for researchers is designed to ensure that data enters the PDB efficiently and safely. It involves several steps, including verifying the data's quality and its significance to the biological community.
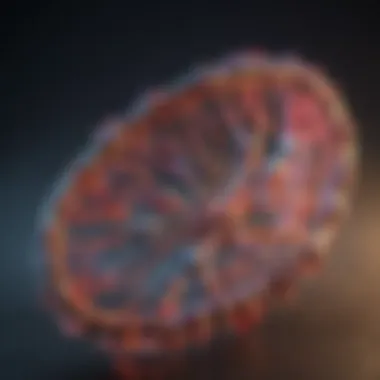
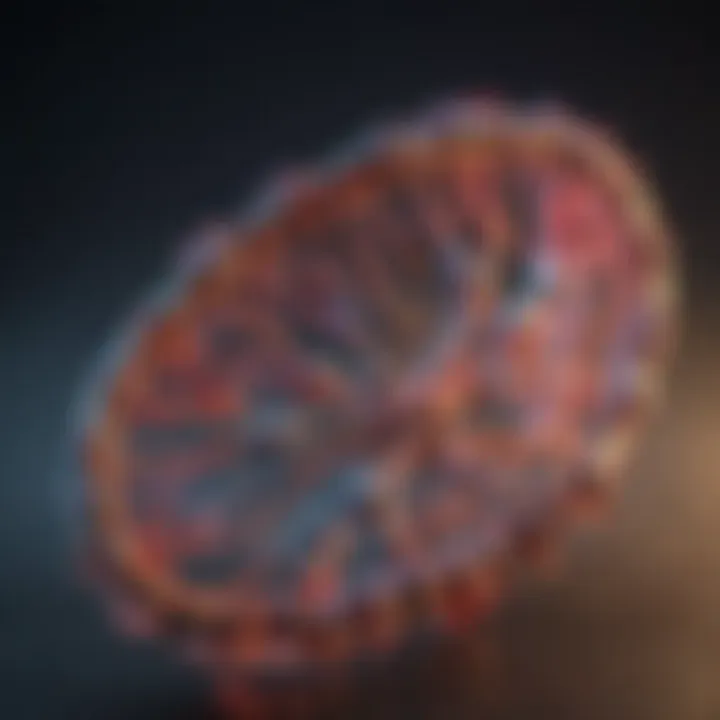
Researchers must follow specific guidelines while submitting their structures. They often begin by preparing their data in compatible file formats. Then, each submission undergoes a thorough review process before becoming publicly available. This scrutiny ensures that only high-quality data contributes to the integrity of the PDB database.
In summary, both data collection and submission are vital aspects of the PDB. They ensure that essential structural information is available for a wide range of applications in scientific research.
Importance of PDB Data Accessibility
Data accessibility is a cornerstone of the Protein Data Bank's function and significance in the scientific community. The PDB serves as a comprehensive repository of experimentally determined structures of biological macromolecules. This valuable resource supports ongoing research by enabling easy access to necessary structural data. By making this information readily available, the PDB enhances collaboration among researchers and institutions, leading to accelerated scientific discovery.
Facilitating Research Collaborations
The PDB provides a platform that promotes collaborations across various scientific disciplines. Researchers who aim to study protein functions or develop new drugs can rapidly access relevant structural data. These structures can reveal crucial insights into molecular mechanisms and interactions, thus fostering interdisciplinary work.
Moreover, many scientific projects rely on external partnerships, often involving universities, pharmaceutical companies, and governmental agencies. The PDB's open access nature encourages sharing of discoveries and findings, which is critical in areas like drug discovery, where understanding protein structures can lead to marking breakthroughs in therapeutic interventions.
"Open access to the PDB data allows researchers to leverage existing knowledge, reducing duplication of efforts and enhancing the pace of advancements in science."
Furthermore, the ability to visualize protein structures using PDB data allows teams to collaborate more effectively. By discussing and evaluating common structures, researchers can share findings globally. The PDB not only simplifies the research process, it empowers collaborative networks that are vital for innovation in structural biology.
Support for Educational Initiatives
The PDB plays a significant role in education by providing valuable resources for students and educators. By offering access to a vast collection of protein structures, educators can incorporate real-world examples into their curriculum, bridging the gap between theory and practice.
In classrooms, students can learn about protein structures through hands-on experience. They can visualize relationships between structures and functions, enhancing their understanding of complex biological concepts. The availability of tools such as visualization software developed around PDB data allows learners to explore molecular structures interactively.
Educational institutions also utilize PDB data in research projects, allowing aspiring scientists to contribute to real-world science. Such initiatives can prepare students for future careers in biotechnology, pharmacology, and bioinformatics. Thus, the PDB not only supports formal education but also nurtures the next generation of scientists.
In summary, the accessibility of PDB data is essential for promoting research collaborations and enhancing educational initiatives. By providing a single point of access for a wealth of structural data, the PDB empowers the scientific community, making it easier to advance our understanding of biology and contribute to the development of new technologies.
Applications of the PDB Database
The Protein Data Bank (PDB) serves as a cornerstone of structural biology, providing invaluable insights into protein structures and functions. Its applications span various fields, notably drug design, protein engineering, and computational biology. Each of these areas benefits significantly from the rich, structured data hosted by the PDB, facilitating innovative research and advancements in science. Understanding the applications within these domains is critical for researchers and professionals alike, as it underscores the database's relevance and enhances its role in ongoing scientific endeavors.
Drug Design and Development
The PDB acts as a crucial resource in the pharmaceutical industry, especially in the drug design and development phase. Structures of target proteins, obtained from the PDB, inform researchers about potential binding sites for new drugs. By analyzing these structures, medicinal chemists can create compounds that interact effectively with specific proteins, enhancing therapeutic efficacy while minimizing side effects.
Furthermore, the influence of the PDB extends beyond mere structure access. Sophisticated tools allow researchers to perform virtual screening against protein structures, streamlining the identification of promising drug candidates.
In recent years, structure-based drug design has gained momentum, enabling scientists to optimize lead compounds through iterative cycles of design, synthesis, and testing. This has resulted in the accelerated development of new medications, addressing various diseases ranging from cancer to infectious conditions.
"The PDBβs role in drug design is not just foundational; it is transformative, enabling rapid advancements in medical treatments."
Protein Engineering
Protein engineering represents another significant application of PDB data. With structural information at hand, researchers can manipulate protein characteristics to develop novel proteins with enhanced functionalities. This has implications in multiple industries, such as biotechnology and agriculture.
Through techniques like site-directed mutagenesis, scientists can alter specific amino acids to improve protein stability, activity, or specificity. The data from the PDB supports these modifications by providing a detailed understanding of protein conformation and interactions.
Moreover, the integration of PDB data with machine learning techniques is opening avenues for predictive modeling. Researchers can now anticipate how changes at the molecular level affect overall protein performance, bringing about advancements in enzyme design and bio-catalysis.
Computational Biology
In the realm of computational biology, the PDB plays a pivotal role in simulations and modeling studies. The atomic coordinates provided in PDB entries allow researchers to perform molecular dynamics simulations, studying how proteins move and evolve over time. This detailed dynamic understanding can reveal critical insights into protein function and interactions.
Understanding protein-ligand interactions, folding pathways, and stability are critical for comprehensive research.
Additionally, the availability of large datasets from the PDB supports bioinformatics approaches that can unveil correlations between structure and function across diverse protein families. Such analyses are essential for evolutionary studies and understanding disease mechanisms at the molecular level.
The integration of PDB data with computational tools fosters an environment ripe for discovery and innovation, driving forward advancements that shape the future of life sciences.
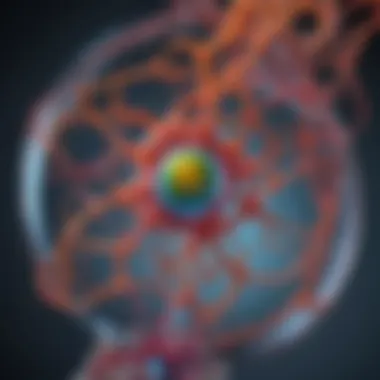
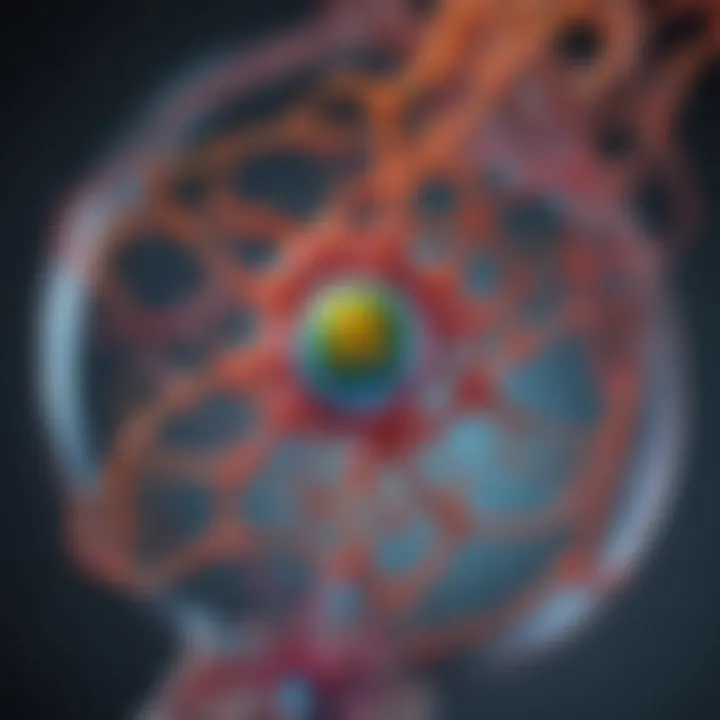
Notable Case Studies Utilizing PDB Data
The Protein Data Bank (PDB) serves as a foundational resource for a plethora of significant scientific studies. Its impact is seen across various disciplines, particularly in drug discovery and functional analysis of proteins. This section explores notable case studies that exemplify the vital role of PDB data in modern research.
Innovative Drug Discoveries
Drug discovery has undergone monumental changes with the advent of structural biology. The PDB provides a crucial repository of 3D structures of biological macromolecules which aid in understanding the interactions between drugs and their target proteins. For instance, the development of HIV protease inhibitors, such as Ritonavir, showcases how PDB data can inform drug design. By analyzing the structure of HIV protease available in PDB, researchers have been able to identify binding sites and optimize compounds accordingly.
In another example, the discovery of new antibiotics has been enhanced through PDB data. The structural analysis of bacterial ribosomes has led to the development of novel antibiotics that specifically target these structures, minimizing the chance for resistance to occur. Access to high-resolution structures allows for rational drug design, ensuring that new compounds have the potential to be effective.
Protein Function Analyses
Understanding the function of proteins is crucial for advancing biochemical and medical sciences. Case studies involving the PDB have enabled researchers to delve deeper into protein functions. One prominent case includes the study of enzyme mechanisms using the structure of carbonic anhydrase. The PDB structure facilitated insights into the catalytic process and substrate interaction, enriching our understanding of enzyme kinetics.
Another notable study involves the analysis of G-protein coupled receptors (GPCRs), important in cell signaling. The PDB provides structural information that has been used in understanding the activation mechanisms of these receptors, offering possibilities for drug targeting. This insight into protein function not only enhances our biological knowledge but also drives therapeutic development.
"The PDB is indispensable for integrating structural biology into drug design and discovery processes. Without such a resource, many breakthroughs would not have been possible."
Future Directions for PDB Development
The Protein Data Bank (PDB) is at a critical juncture where its evolution can significantly enhance structural biology. The pace of scientific discovery, fueled by advancements in technology, necessitates continuous development in the PDB's infrastructure and data management capabilities. The future of the PDB hinges on the incorporation of contemporary trends in collaborative research and data visualization. This section will explore these essential directions, highlighting their potential benefits and the considerations that must be addressed as the PDB continues to grow.
Integration with Other Databases
Integrating the PDB with other specialized databases can foster a more comprehensive understanding of biological macromolecules. By establishing linkages with repositories such as UniProt for protein sequences or the Gene Ontology for functional annotations, researchers can access multidimensional data with ease. This interconnectedness promotes an interdisciplinary approach to studying proteins and allows for richer analysis.
Benefits of integration include:
- Enhanced Data Accessibility: Researchers can retrieve comprehensive datasets without switching between multiple platforms, streamlining their workflow.
- Improved Research Synergy: Different scientific fields, from genomics to proteomics, can gain insights into the structure-function relationships of proteins, fostering collaborative projects.
- Holistic Insights: By combining structural information with functional and sequence data, scientists can formulate hypotheses more effectively.
This integration requires careful planning. Data standards and formats must align, ensuring interoperability. Moreover, continuous updates to all linked databases are crucial to maintain recognized accuracy. Thus, while the promise of integration is substantial, dedicated effort is required to ensure its effective realization.
Enhancements in Data Visualization
The visual representation of molecular structures plays a fundamental role in structural biology. Enhancements in data visualization techniques are vital for improving the interpretability of complex datasets. Advanced visualization tools can assist researchers in identifying patterns, abnormalities, or vital features within molecular structures more effectively.
Potential enhancements include:
- 3D Interactive Models: Tools allowing users to manipulate and explore models in a three-dimensional space, enhancing their understanding of structural relationships.
- Dynamic Simulations: Real-time simulations of molecular interactions can provide insights into the behavior of proteins under different conditions.
- User-Centric Interface Improvements: Simplifying navigation and user experiences can facilitate broader access, especially for educational purposes.
By embracing these enhancements, the PDB can become a more potent tool for education and research, catalyzing discoveries in structural biology.
The commitment to improving visualization must also take into account user expertise. Advanced tools should not alienate those with less technical proficiency. Hence, tutorials and resources to support a wide range of users will be equally essential. The balance between complexity and accessibility will determine the success of these developments.
Ends
In summation, the conclusions drawn from the discourse on the Protein Data Bank (PDB) elucidate its indispensable role in the field of structural biology. The PDB acts as a central repository where critical biological data is stored, facilitating research and innovation. One of its key impacts lies in its capacity to streamline the process of data sharing among scientists worldwide. This has accelerated discoveries in drug design, protein engineering, and various other biological applications.
Recap of PDB Impact on Science
The influence of the PDB on science cannot be overstated. The database has transformed how researchers approach structural biology. By providing easy access to a vast array of protein structures, it has opened avenues for new research methodologies. For instance, prior to the existence of the PDB, obtaining structural data was cumbersome and often limited. Now, scientists can readily access detailed information on protein structures that are vital for studies in drug discovery, medical research, and biotechnology innovations. The PDB's ongoing updates based on new findings ensure that it remains a relevant resource, thereby continuing to foster advancements in science.
Call to Action for Continued Research
It is crucial for the scientific community to actively engage with the PDB and to advocate for its enhancement. Continued research efforts will not only improve the quality of the database but also enrich our understanding of protein functions and interactions. This includes encouraging new submissions from researchers using modern techniques. Scientists and institutions should also invest their resources into exploring innovative uses of the data. Emphasizing reproducibility and transparency in research can significantly boost the value drawn from the PDB. In this way, the PDB can sustain its momentum as a foundational pillar in structural biology for years to come.