Mitochondrial Repair Therapy: A Comprehensive Overview
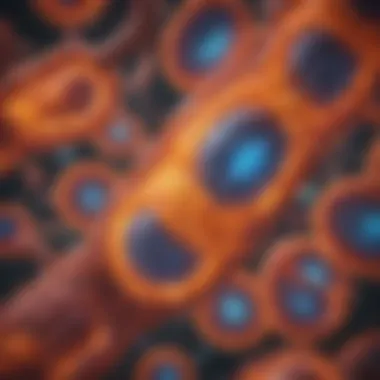
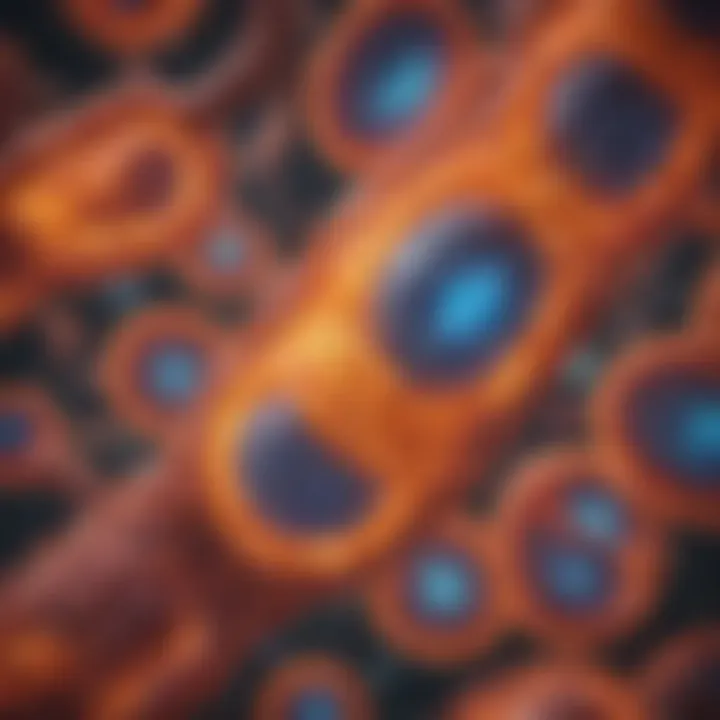
Intro
Mitochondrial repair therapy stands as a significant and innovative domain within the realm of medical science. It addresses a crucial aspect of cellular health by focusing on the mitochondria, often labeled as the "powerhouses" of the cell. These organelles are vital for energy production, influencing both metabolic processes and overall cell function. The growing interest in this area stems from increasing recognition of mitochondrial dysfunction as a contributing factor to a array of diseases, including neurodegenerative disorders, metabolic syndromes, and even some forms of cancer.
As researchers unveil the complexities of mitochondrial biology, therapeutic strategies continue to evolve. This exploration details both the foundational principles behind mitochondrial function and innovative approaches designed to restore it. Through a careful examination of current trends and findings, this article aims to illuminate how mitochondrial repair therapy can transform therapeutic practices in modern medicine.
Background and Context
Overview of the Research Topic
The study of mitochondrial repair therapy is anchored in the understanding of mitochondrial wellbeing and its far-reaching implications for health. Mitochondria generate adenosine triphosphate (ATP), the energy currency for cellular processes. Beyond this, they play roles in regulating metabolism, apoptosis, and reactive oxygen species (ROS) production. Disruption to mitochondrial function can lead to an energy crisis within cells, triggering a cascade of pathological events.
Mitochondrial repair therapy seeks to restore this function. Techniques being explored range from gene therapy, which aims to correct genetic dysfunction, to targeted antioxidants that mitigate ROS damage. Additionally, advancements in stem cell therapy show promise in regenerating damaged mitochondria.
Historical Significance
The historical trajectory of research into mitochondria began in the late 19th century. However, it was not until the 20th century that our understanding began to take shape. The discovery of mitochondrial DNA in the 1960s was a breakthrough that spurred innovations in genetic studies and linkages to various diseases.
In recent decades, the focus has shifted towards understanding how best to harness this knowledge for therapeutic purposes. In 2010, a pivotal study shed light on the role of mitochondrial dysfunction in aging and degenerative diseases, propelling a wave of research aimed at developing tangible therapies. As the body of research expands, major findings now signal pathways through which therapy can remedy mitochondrial imbalances, with the potential to alter the landscape of treatment options significantly.
Key Findings and Discussion
Major Results of the Study
Current research in mitochondrial repair therapy highlights several promising findings:
- Gene therapy: Techniques to introduce or modify genes that regulate mitochondrial function show potential, targeting conditions caused by specific genetic mutations.
- Mitochondrial replacement techniques: Emerging methods aim to replace defective mitochondria in oocytes, providing possibilities for preventing mitochondrial diseases in subsequent generations.
- Pharmacotherapy: Compounds like EPI-743 have demonstrated efficacy in mitigating mitochondrial dysfunction in clinical trials for conditions such as Friedreich's ataxia.
Detailed Analysis of Findings
Each of these areas is underpinned by compelling data indicating progress in mitigating the effects of mitochondrial diseases.
For instance, gene therapy offers hope for patients suffering from Leigh syndrome caused by mitochondrial mutations. Initial results suggest improvements in motor function and cognitive abilities after treatment. Similarly, pharmacologic interventions are under evaluation in multiple clinical trials, indicating a trend towards more accessible treatments for those affected by dysfunctional mitochondria.
"The ongoing advancements in mitochondrial research are revolutionizing our understanding of various diseases and their treatments, heralding a new era in regenerative medicine."
As this field evolves, the implications of these findings are vast, potentially shifting how we approach medical conditions inherently linked to mitochondrial health. With each breakthrough, the future of mitochondrial repair therapy appears increasingly promising, poised to become a cornerstone in the treatment of numerous conditions.
Understanding Mitochondria
Mitochondria are often referred to as the powerhouses of the cell, and for good reason. Their primary role is to generate adenosine triphosphate (ATP), the essential energy currency that powers countless biological processes. Beyond energy production, mitochondria also play significant roles in cellular metabolism, apoptosis, and maintaining cellular homeostasis. Understanding the intricacies of mitochondrial function is pivotal in the context of mitochondrial repair therapy.
Mitochondrial dysfunction is linked to a diverse array of diseases, making it vital to grasp their structure, role in metabolism, and genetic components. This knowledge lays the foundation for new treatment strategies aimed at revitalizing mitochondrial function.
In this article, we will delve into the key facets of mitochondrial biology. A comprehensive understanding of mitochondria can help us appreciate how their dysfunction can lead to various pathologies. This overview is essential, as it illuminates the rationale behind developing therapies that target these organelles in the quest for improved health outcomes.
Mitochondrial Structure and Function
Mitochondria are unique organelles characterized by a double-membrane system. The outer membrane is smooth and allows small molecules and ions to pass freely. In contrast, the inner membrane is highly folded into structures known as cristae, significantly increasing the surface area for biochemical reactions. Within this inner membrane lies the mitochondrial matrix, containing enzymes, mitochondrial DNA, and ribosomes. This distinct architecture is crucial for their function.
The primary function of mitochondria is aerobic respiration, where energy is produced through the electron transport chain, resulting in ATP synthesis. This process also generates reactive oxygen species (ROS), which, while necessary for signaling, can become harmful in excess. Thus, maintaining mitochondrial balance is critical.
Role in Cellular Metabolism
Mitochondria are at the core of cellular metabolism, linking the various metabolic pathways that sustain life. They facilitate the oxidative breakdown of carbohydrates, fats, and proteins, converting these macromolecules into usable energy forms. Mitochondria are central to numerous metabolic processes, including the citric acid cycle, fatty acid oxidation, and the urea cycle, highlighting their importance in overall physiological function.
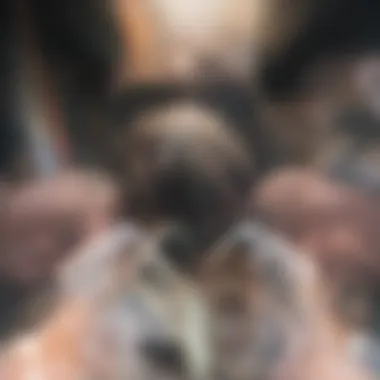
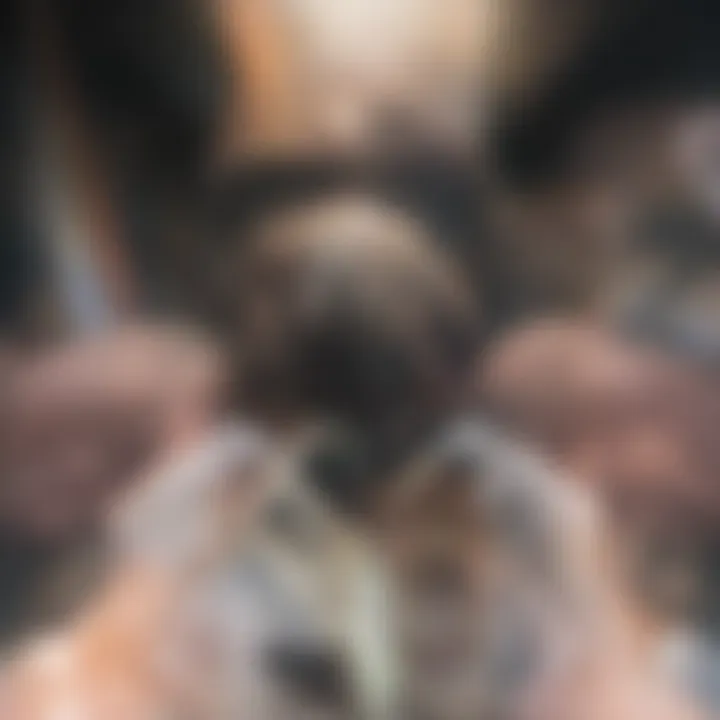
Moreover, mitochondria help regulate metabolic pathways involving insulin signaling and the metabolism of glucose and lipids. The capacity of mitochondria to coordinate these pathways underscores their role as metabolic hubs in the cell.
Mitochondrial Genome
Mitochondria possess their own distinct genome, separate from the nuclear DNA. This mitochondrial DNA (mtDNA) is circular and contains genes essential for mitochondrial function, particularly those involved in oxidative phosphorylation. The unique inheritance pattern, being passed down maternally, makes mtDNA a focal point in studies of genetic diseases linked to mitochondrial dysfunction.
Mutations in mtDNA can lead to a variety of inherited mitochondrial disorders, evidencing the significance of these genetic elements. The study of the mitochondrial genome is pivotal in understanding many pathologies, facilitating research aimed at correcting genetic defects through strategies such as gene therapy.
"The structure, metabolism, and genetic details of mitochondria form the basis for exploring therapeutic avenues aimed at restoring their function."
The Impact of Mitochondrial Dysfunction
Mitochondrial dysfunction stands as a critical aspect of human health, influencing various biological processes and diseases. Mitochondria are essential organelles that not only serve as powerhouses for cell energy but also participate in a myriad of cellular activities. When their function declines, it can lead to significant health consequences, ranging from metabolic disorders to neurodegenerative diseases. Understanding the impact of mitochondrial dysfunction is paramount for developing effective therapies that target these intricate biological systems.
Bioenergetics and Disease
Bioenergetics refers to the flow of energy within living systems. Mitochondria play a pivotal role in this process by generating adenosine triphosphate (ATP) through oxidative phosphorylation. When mitochondrial function deteriorates, ATP production decreases, which can disrupt cellular processes. Conditions such as diabetes, heart disease, and chronic fatigue syndrome have been linked to impaired mitochondrial energy metabolism.
In these diseases, the energy deficit can lead to cellular stress, causing inflammation and damage. The crucial point here is that mitochondrial health is fundamental for maintaining energy homeostasis. Thus, strategies aimed at improving bioenergetics may hold promise for alleviating disease symptoms and enhancing overall health.
Mitochondrial Dysfunction in Aging
Aging is a complex biological process, and mitochondrial dysfunction has emerged as a key component. As individuals age, mitochondrial DNA accumulates mutations, and the efficiency of the electron transport chain often declines. This results in increased production of reactive oxygen species (ROS), which can inflict further damage on cellular structures, including lipids, proteins, and DNA.
The relationship between mitochondrial dysfunction and aging results in a vicious cycle. The declining mitochondrial function contributes to age-related diseases, such as Alzheimer's and Parkinson's disease. Moreover, the role of mitochondria in cellular senescence is gaining attention, as this process can accelerate the aging phenotype, impacting tissue function and resilience. Recognizing these factors is essential in the quest for interventions that may slow down the aging process, specifically those that focus on enhancing mitochondrial health.
Neurological Disorders Linked to Mitochondrial Damage
Neurological disorders are frequently associated with mitochondrial damage. Conditions such as Huntington's disease, amyotrophic lateral sclerosis (ALS), and multiple sclerosis have mitochondrial dysfunction at their core. This damage can lead to neurodegeneration, characterized by the loss of neuronal integrity and function.
Mitochondria are crucial for neuronal survival due to their role in energy production and regulation of apoptotic pathways. When these organelles fail, neurons cannot maintain their energy requirements, leading to cell death. Furthermore, impaired mitochondrial dynamics can exacerbate neuroinflammation, a common feature in many neurodegenerative conditions. Understanding the pathways through which mitochondrial dysfunction contributes to neurological disorders is essential for developing targeted therapeutic strategies.
Mitochondrial Role in Metabolic Disorders
Mitochondrial dysfunction is central to the pathogenesis of metabolic disorders, such as obesity and Type 2 diabetes. Mitochondria not only manage energy production but also play roles in fat metabolism and insulin sensitivity. When mitochondrial function declines, it can lead to an imbalance in energy storage and expenditure.
In obesity, it is observed that there is often a reduction in mitochondrial capacity. This diminishes the body’s ability to efficiently oxidize fatty acids, contributing to insulin resistance. Consequently, understanding the mitochondrial mechanisms in these disorders can lead to strategies that promote metabolic flexibility and improve overall health.
In summary, the impact of mitochondrial dysfunction spans a spectrum of critical health issues. By recognizing the interconnectedness of mitochondrial health with various diseases, researchers and clinicians can work towards innovative therapeutic approaches that potentially mitigate the deleterious effects of mitochondrial impairment.
Current Approaches to Mitochondrial Repair
Mitochondrial repair therapy plays a critical role in addressing mitochondrial dysfunction, which is linked to various diseases. The importance of this topic lies in the innovative approaches that aim to restore mitochondrial health. These strategies can potentially enhance energy production, reduce oxidative stress, and improve overall cellular function. Understanding these approaches is essential for both researchers and clinicians, as they pave the way for future treatment modalities.
Antioxidant Strategies
Antioxidant strategies focus on combatting oxidative stress that damages mitochondrial components. Mitochondria are natural producers of reactive oxygen species (ROS), which can lead to cellular damage if not balanced. Approaches like using N-acetylcysteine (NAC) and Coenzyme Q10 have gained attention.
These antioxidants can help neutralize excess ROS, protecting mitochondrial integrity. They enhance electron transport chains and boost ATP production. Their role in improving mitochondrial function is under active research. Ongoing studies explore their combination with other therapies to optimize outcomes.
Gene Therapy Techniques
Gene therapy offers a target-focused approach to correct mitochondrial dysfunction at the genetic level. Techniques include the use of CRISPR-Cas9 technology to repair mutated mitochondrial DNA. This method aims to restore normal function by replacing or editing specific genes responsible for the dysfunction.
Current research highlights the potential of this technique for treating genetic mitochondrial disorders, such as Leber’s Hereditary Optic Neuropathy. Challenges such as delivery mechanisms and off-target effects remain prevalent, demanding rigorous examination in controlled environments.
Pharmacological Interventions
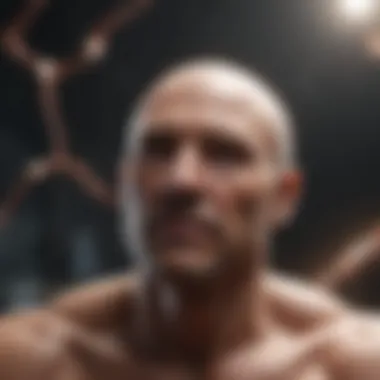
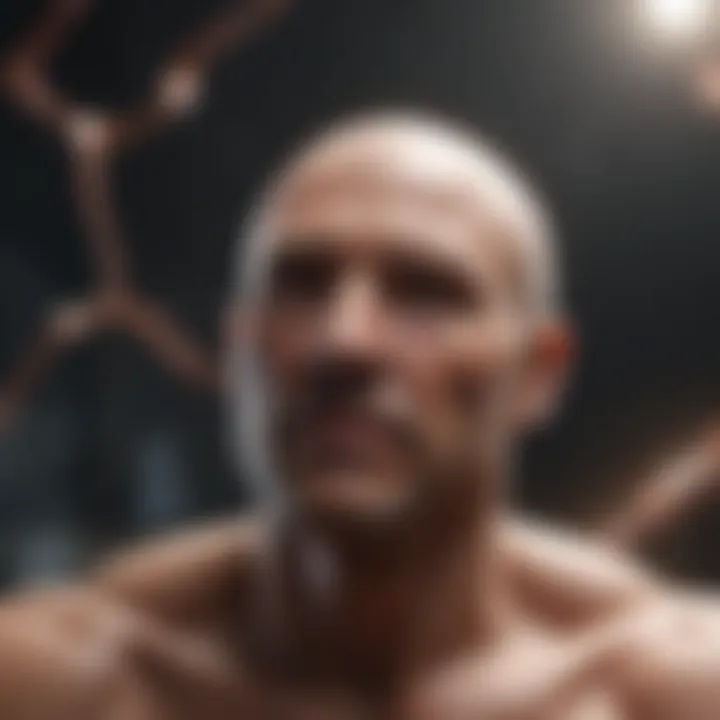
Pharmacological interventions can optimize mitochondrial function through the administration of specific drugs. For example, substances like Metformin, widely known for its anti-diabetic effects, have shown promise in improving mitochondrial biogenesis. Similarly, drugs that target mitochondrial pathways can enhance energy production and decrease cell death in conditions like heart failure.
The consideration of drug interactions and patient-specific factors is crucial in these interventions. Further clinical trials are essential to establish efficacy and safety for various mitochondrial-related diseases.
Cellular Reprogramming Approaches
Cellular reprogramming approaches offer a novel pathway to rejuvenate damaged mitochondria. By inducing stem cell-like properties, scientists can create cells with enhanced regenerative capabilities. The interest in factors like Yamanaka factors demonstrates potential for creating pluripotent cells that rejuvenate defective mitochondria.
This area of research is still developing. There is an ongoing debate regarding the ethical implications and safety of such techniques. However, the prospects of restoring cellular health through reprogramming cannot be overlooked.
As the field of mitochondrial repair therapy evolves, it is evident that understanding the underlying mechanisms of these approaches can lead to enriched therapies in the context of various diseases.
Innovative Mitochondrial Repair Therapies
Innovative mitochondrial repair therapies represent a transformative shift in the approach to treating mitochondrial dysfunction. These therapies target the underlying issues related to mitochondrial capabilities, offering the potential to reverse, or at least ameliorate, the cellular defects associated with various diseases. They hold promise for enhancing both the quality of life and longevity of individuals affected by mitochondrial-related ailments. This section will delve into specific elements related to the use of stem cells, regenerative medicine, and mitochondrial replacement techniques.
Stem Cell-Based Interventions
Stem cell-based interventions are emerging as a valuable tool in combating mitochondrial dysfunction. The ability of stem cells to differentiate into various cell types allows for the potential repair or replacement of damaged cellular mitochondrial function. In certain conditions, such as muscular dystrophies or neurodegenerative diseases, stem cells can be engineered to enhance mitochondrial performance. For instance, researchers are exploring the use of induced pluripotent stem cells (iPSCs), which can be derived from adult cells, making them a versatile option for patients. By reprogramming these cells to restore or optimize mitochondrial function, it may be possible to improve overall cellular energy levels and mitigate disease progression.
Moreover, stem cell therapies can offer regenerative benefits by promoting cellular repair mechanisms. When administered correctly, they may aid in the restoration of energy production and reduce the impacts of mitochondrial dysfunction on cellular processes. However, there are still challenges to be addressed, such as ensuring the safe integration of these stem cells into existing tissues without adverse effects.
Regenerative Medicine Strategies
Regenerative medicine strategies seek to harness the body's own repair mechanisms to restore mitochondrial function. This can be achieved using various approaches, such as tissue engineering, where biomaterials and cells are combined to create functional tissue constructs. The aim is to cultivate new mitochondria, replenishing those that are damaged or dysfunctional. Key advancements in biomaterials and scaffolding technologies are enabling researchers to create environments that promote mitochondrial health and functionality.
In addition, the exploration of pharmacological agents that support tissue regeneration provides another avenue for mitochondrial repair. Compounds like antioxidant molecules can mitigate oxidative stress, which is detrimental to mitochondria. Strategies aimed at enhancing mitochondrial biogenesis are also critical. For example, exercise has been shown to stimulate the production of new mitochondria, suggesting that lifestyle changes can complement more direct therapeutic interventions.
Mitochondrial Replacement Therapy
Mitochondrial replacement therapy (MRT) is a groundbreaking approach designed to prevent the transmission of mitochondrial diseases from mother to child. This technique involves replacing defective mitochondria in a patient's egg or embryo with healthy mitochondria from a donor. There are several methodologies to achieve this, such as pronuclear transfer, where two embryos are created, one with defective mitochondria and the other with healthy ones, and then extracting the nucleus from the affected embryo and implanting it into the healthy one.
MRT raises important considerations, especially ethical implications about gene modification and the potential long-term effects on offspring. There are regulatory hurdles to navigate, as the therapy itself is relatively new within the medical landscape. Nonetheless, MRT could pave the way for eradicating certain mitochondrial disorders and offers hope to families affected by these chronic conditions.
"Mitochondrial replacement therapy holds profound implications for genetic diseases, marking a significant advancement in reproductive medicine."
By investigating these innovative therapies, we can begin to understand their role in reshaping mitochondrial repair strategies, potentially leading to better outcomes for patients suffering from mitochondrial dysfunction.
Clinical Applications of Mitochondrial Repair Therapy
The exploration of mitochondrial repair therapy is critical in the modern healthcare landscape. Understanding the clinical applications allows for a deeper appreciation of potential benefits derived from restoring mitochondrial function. Mitochondrial dysfunction is linked to numerous diseases, making targeted interventions highly relevant. By focusing on specific diseases where mitochondrial repair can play a role, we uncover the applicability of theoretical concepts in practical situations.
Treatment of Muscular Dystrophies
Muscular dystrophies, a group of genetic disorders characterized by progressive skeletal muscle degeneration, present significant challenges in treatment options. Mitochondrial dysfunction has been identified as contributing to muscle degeneration. Therapeutic strategies such as gene therapy and supplementation with coenzyme Q10 have shown promising results. By enhancing mitochondrial function, these approaches aim to improve muscle strength and endurance in affected individuals. This focus on mitochondrial health is leading researchers to explore various methods for repairing or replacing damaged mitochondria in muscle cells.
Neurodegenerative Disease Management
The management of neurodegenerative diseases, including Alzheimer’s and Parkinson’s, highlights the importance of mitochondrial health in the brain. Mitochondrial repair therapy focuses on methods such as neuron-specific gene therapy to repair mitochondria. Benefits include improved energy metabolism and reduced oxidative stress within neuronal cells. Clinical trials have indicated potential for these therapies to slow disease progression or improve quality of life. This area of research continues to grow, indicating that mitochondrial dysfunction could be central to novel therapeutic strategies.
Implications for Cardiovascular Conditions
Mitochondrial repair therapy has significant implications for cardiovascular health. Conditions such as ischemic heart disease and heart failure may be linked to mitochondrial malfunction. Interventions can include antioxidants that target mitochondrial oxidative stress, aiming to restore cardiovascular function. Enhanced mitochondrial function has been associated with improved heart performance and better patient outcomes. The prospect of applying these therapies in clinical practice raises expectations for future treatments in cardiovascular diseases.
Mitochondrial repair therapy offers a path to treat complex conditions that arise from mitochondrial dysfunction, potentially changing the landscape of how we approach various diseases.
By understanding these clinical applications, we can better appreciate the role of mitochondrial repair therapy in transforming treatment approaches. Each category of application exhibits unique benefits and strategies that highlight the necessity of continuing research in this area. This ongoing exploration may lead to breakthroughs that improve clinical outcomes for diverse patient populations.
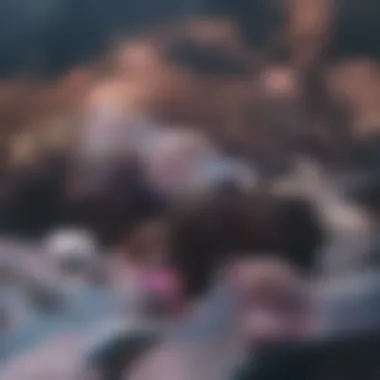
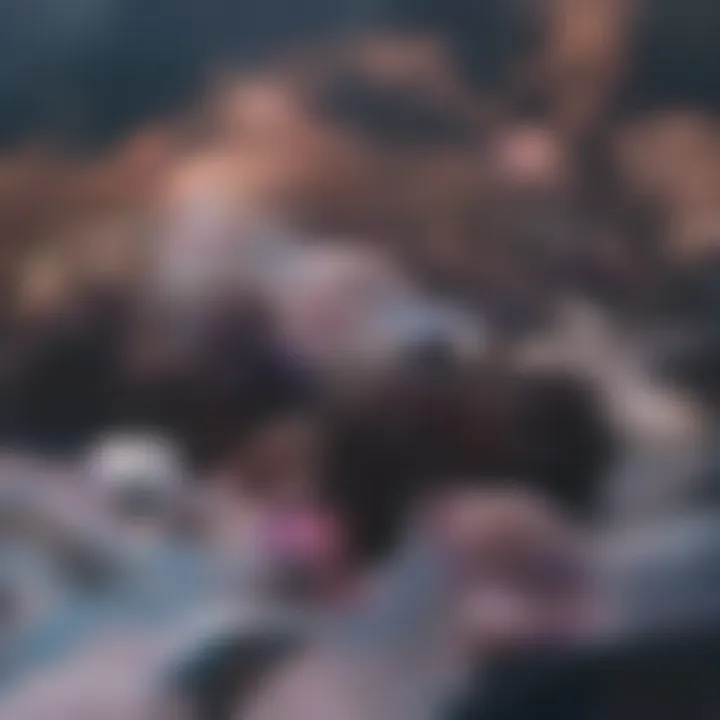
Ethical Considerations
The discussion around mitochondrial repair therapy encompasses not just the scientific advancements but also the ethical landscape that comes with such innovations. As therapeutic strategies evolve, it is imperative to consider ethical implications. This is especially true as mitochondria play a crucial role in human health and are increasingly targeted for medical interventions. The potential benefits of enhanced mitochondrial function must be weighed against the risks and moral considerations that accompany such therapies.
One of the core elements of ethical consideration is the regulatory framework for mitochondrial therapy. Regulatory bodies must establish guidelines to ensure the safety and efficacy of treatments involving mitochondrial manipulation. It is essential for laws to adapt alongside innovations to safeguard both medical practitioners and patients. Current regulations vary widely across countries, leading to discrepancies in therapy availability and standards. This uncertainty can lead to unjustified practices or the commercial exploitation of unproven therapies. Thus, a uniform regulatory structure can help unify approaches and ensure that mitochondrial therapies are tested rigorously before public use.
Regulatory Framework for Mitochondrial Therapy
Developing appropriate regulations is a multi-faceted process involving scientific assessment, public health considerations, and ethical scrutiny. Governments and health organizations need to evaluate existing protocols while considering advances in genetic medicine and biotechnology. Regulatory frameworks must cover:
- Safety evaluations: Ensuring any therapy poses minimal risk to recipients.
- Efficacy validation: Confirming that treatments effectively restore mitochondrial function without adverse side effects.
- Informed consent: Patients must understand the implications of undergoing mitochondrial therapy, including potential outcomes and risks.
Furthermore, ongoing research plays a significant role. As new findings emerge, regulatory bodies must remain flexible to incorporate new knowledge. Keeping the regulations updated ensures that ethical standards can evolve to match the pace of scientific progress.
Ethical Implications of Mitochondrial Replacement
Mitochondrial replacement therapy presents unique ethical quandaries. While the primary goal is to treat and prevent diseases linked to mitochondrial dysfunction, several grey areas arise.
Some of the ethical implications include:
- Germline Modification: Mitochondrial replacement techniques may lead to changes in germline cells, potentially affecting future generations. This raises questions about consent for unborn individuals.
- Societal Impact: As these therapies become accessible, the risk of social disparities should not be overlooked. Access might be more pronounced in wealthier populations, leading to potential inequalities in treatment.
- Possible Misuse: There is concern that such technologies could be used for non-therapeutic enhancements, which raises moral questions about the extent to which we should intervene in human biology.
"The ethical implications of mitochondrial therapies are not just theoretical; they impact policy-making and public opinions about genetic modification and human intervention."
Future Directions in Mitochondrial Therapy
The exploration of mitochondrial therapy is on the rise and is reshaping understandings in cellular and molecular medicine. Key elements include innovative research areas and the integration of personalized medicine approaches. Emphasizing these aspects is important for grasping the next steps in treating mitochondrial-related diseases. These therapies could profoundly affect current treatment modalities, leading to more effective and targeted interventions.
Emerging Research Areas
The scientific community is focusing on various research avenues to uncover new strategies for mitochondrial repair therapy. Some important areas include:
- Mitochondrial Biogenesis: Researchers are investigating ways to stimulate the growth and division of healthy mitochondria. This can improve overall cellular function and combat the effects of aging.
- CRISPR Gene Editing: The technology's potential lies in its ability to correct genetic mutations in mitochondrial DNA. This could become a viable method for treating genetic disorders linked to these organelles.
- Wearable Technologies: Advances in monitoring mitochondrial function through wearable devices could provide real-time data on metabolic activity, facilitating timely interventions.
- Nutraceuticals: Certain compounds, like Coenzyme Q10 and PQQ, are under scrutiny for their roles in enhancing mitochondrial function. Research continues to ascertain their effectiveness and safety.
Overall, these research areas hold promise for the development of new therapies that provide greater benefits with fewer side effects.
Potential for Personalized Medicine
Personalized medicine stands to revolutionize the way mitochondrial therapy is applied to individual patients. This approach tailors treatment based on a person's unique genetic profile, lifestyle, and specific mitochondrial dysfunctions.
- Tailored Interventions: Each person's mitochondrial makeup can affect how they respond to therapies. Personalized strategies may lead to customized treatments, maximizing efficacy and minimizing adverse reactions.
- Genetic Profiling: Understanding genetic predispositions will allow clinicians to predict which therapies will work best for different patients. Using tools like genomic sequencing, targeted actions can be designed.
- Patient-Centric Innovations: Integrating patient feedback into clinical trials can foster the development of therapies that resonate more effectively with those receiving treatment.
Ending
The conclusion serves as a crucial capstone to this extensive exploration of mitochondrial repair therapy. Here, the importance of recognizing the interplay between mitochondrial function and overall health becomes evident. Advances in the understanding of mitochondria have led to innovative therapies that promise to address various diseases. These developments indicate a paradigm shift in treatment options, steeping traditional medical practices in newer biologic insights.
Much has been detailed throughout this article — from the fundamental roles of mitochondria in bioenergetics to the consequences of their dysfunction in several health conditions. The emerging therapies highlighted reflect a growing commitment to not only treat symptoms but also target the underlying mitochondrial impairments. This is particularly significant for diseases where there are limited treatment options.
Additionally, ethical considerations and future directions provide a robust framework for how mitochondrial therapies might evolve further. Ensuring that these therapies are deployed responsibly will be paramount.
Summary of Key Points
- Mitochondria are essential for maintaining cellular energy balance.
- Dysfunction can trigger a wide range of health issues, including neurodegenerative and metabolic disorders.
- Current repair strategies include antioxidants, gene therapy, and regenerative medicine.
- Ethical implications must be considered as therapies advance.
- Personalized approaches may increase the efficacy of treatments in the future.
The Future of Mitochondrial Repair Therapy
The landscape of mitochondrial repair therapy holds promise for personalized medicine. Future research will likely uncover more precise mechanisms that can be targeted for intervention. Unraveling the complexities of mitochondrial dynamics may also enhance our understanding of associated diseases.
Key areas of focus in future studies will include:
- Development of targeted delivery systems for therapies.
- Exploration of mitochondrial function in stem cell therapy.
- Understanding the interactions between mitochondrial health and systemic diseases.
As scientists continue to innovate, the potential to revolutionize how we treat a variety of health conditions is significant. Mitochondrial repair therapy may well become a cornerstone of modern medical practice, offering hope and improved outcomes for patients across the globe.