Effective Strategies for Measuring Capacitance
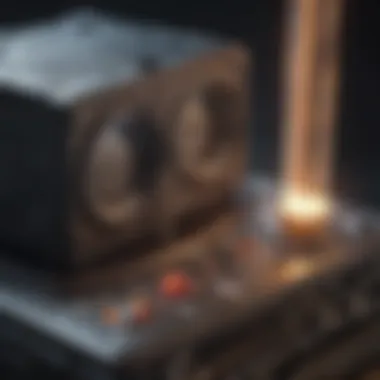
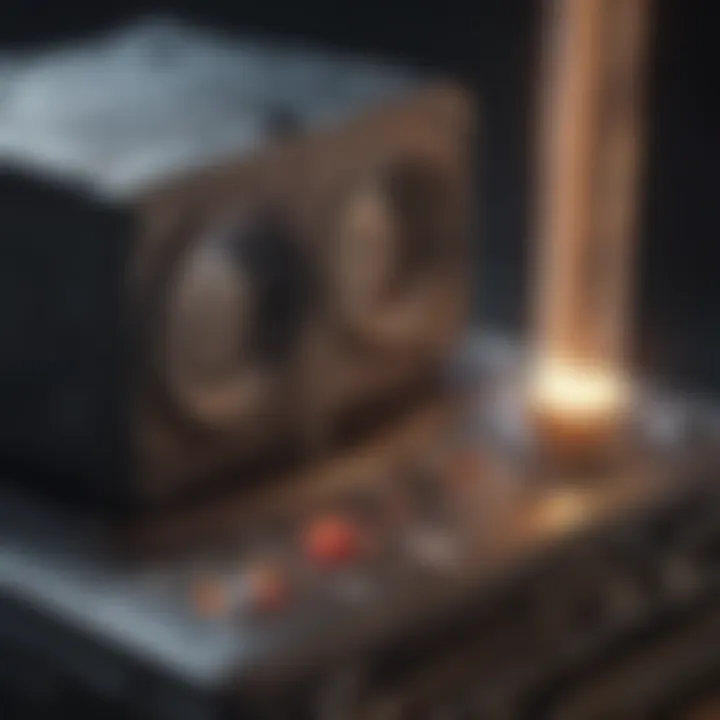
Intro
Capacitance measurement is crucial in various fields of electronics. Understanding capacitance helps in designing circuits and diagnosing issues effectively. It involves quantifying a capacitor's ability to store electrical charge. This article investigates methods to measure capacitance in electrical systems, emphasizing the importance of precise measurements and the role they play in electronics.
Background and Context
Overview of the Research Topic
The measurement of capacitance is a fundamental aspect of electrical engineering. It can impact circuit performance significantly. The ability to measure capacitance accurately affects the design choices and functionality of devices ranging from simple electronics to complex systems.
Historically, the need for measuring capacitance emerged with the growth of electrical technologies. Early experiments in electricity led to the discovery of capacitors, which store charge. Subsequently, various techniques evolved to gauge capacitance effectively, serving various applications in academia and industry.
Historical Significance
From primitive capacitors made of simple materials to sophisticated modern devices, the journey of capacitance measurement reflects technological progress. Techniques like the use of LCR meters and bridge circuits have emerged, expanding the toolkit for engineers and scientists alike. These advancements are not just historical footnotes; they offer practical lessons in dealing with measurement challenges today.
Key Findings and Discussion
Major Results of the Study
Different techniques for measuring capacitance range widely in complexity and application. For example, using basic capacitors is suitable for low-tech applications, while high-accuracy needs may call for an LCR meter or a bridge circuit. Each method carries distinct pros and cons, which are crucial to consider.
Moreover, the article underscores practical challenges such as stray capacitance, temperature effects, and component aging. Accurate measurement requires awareness of how these challenges may distort readings.
Detailed Analysis of Findings
The techniques discussed include:
- Capacitors: Basic yet effective for specific applications.
- LCR Meters: Provide accurate readings of capacitance along with inductance and resistance.
- Bridge Circuits: Offer high precision, especially in laboratory settings.
Understanding capacitance measurement is essential for effective electrical design and diagnostics.
Preface to Capacitance
Capacitance is a fundamental property of electrical systems, embodying the ability to store electrical charge. Understanding capacitance is essential for engineers, technicians, and anyone involved in electronics. It serves as the basis for various applications, from simple circuits to complex electronic devices. Capacitance directly influences how energy is stored and released, affecting the performance and efficiency of electrical systems.
Definition of Capacitance
Capacitance is defined as the ratio of the electric charge stored on a conductor to the potential difference between its plates. Mathematically, it is represented as:
[ C = \fracQV ]
where (C) is capacitance, (Q) is the charge, and (V) is the voltage. This relationship indicates that a capacitor can hold more charge at a higher voltage, highlighting its capacity to influence circuit behavior.
Different types of capacitors, such as ceramic, electrolytic, and tantalum, exhibit distinct capacitance characteristics based on their material properties and physical structure. Such understanding is crucial for selecting the right component in circuit design.
Importance of Capacitance in Electronics
Capacitance plays a pivotal role in several electronic applications. Here are some key reasons why capacitance measurement is indispensable:
- Energy Storage: Capacitors store electrical energy and release it when needed. This feature is exploited in power management systems, smoothing out voltage fluctuations.
- Filtering Signals: In communication systems, capacitors help filter out noise, allowing for clearer signal transmission. This is particularly important in radio frequency applications.
- Timing Applications: Many circuits utilize capacitors in timing applications, contributing to functions such as oscillators and pulse generators.
- Coupling and Decoupling: Capacitors can couple alternating currents while blocking direct currents, making them essential in audio and signal processing.
Given these applications, a deep understanding of measurement techniques is critical. Precise capacitance values enable engineers to design efficient systems that function reliably in various conditions. Moreover, recognizing how environmental factors and component interactions affect capacitance can significantly enhance diagnostic processes in fault finding and equipment maintenance.
"Accurate capacitance measurement is not just about numbers; it's about ensuring the reliability of electrical systems."
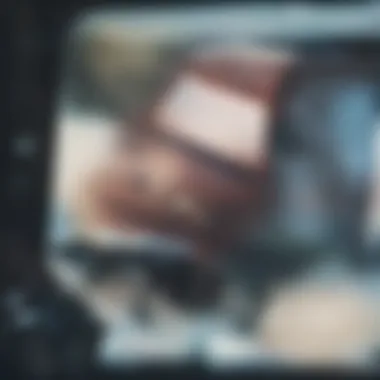
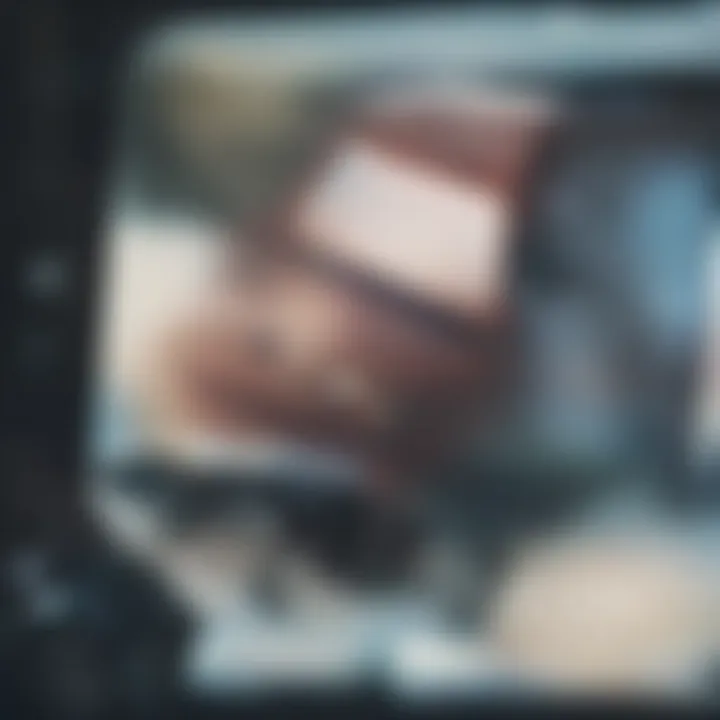
As we delve further into the methods for measuring capacitance, it becomes evident that mastery of these concepts is vital for success in the field of electronics.
Fundamental Concepts of Measuring Capacitance
Understanding the fundamental concepts of measuring capacitance is crucial for anyone involved in electronics. This knowledge helps not only in precise measurements but also in diagnosing issues in electronic systems. The measurement of capacitance affects circuit performance, reliability, and overall design. Therefore, efficient measurement techniques and the factors influencing these values must be understood.
Capacitors and Their Role in Measurement
Capacitors are essential components in electronic systems, serving various functions such as energy storage, filtering, and timing. When measuring capacitance, recognizing the role of these components is vital. Typically, capacitors store electrical energy in an electric field, characterized by their capacitance value measured in farads.
Different types of capacitors, like ceramic, electrolytic, and tantalum, exhibit unique behaviors under varying conditions. Their internal structure and material influence how they perform in real-world scenarios. For instance, electrolytic capacitors have a greater capacitance value but are polarized, limiting their applicable circuitry. Understanding these differences is fundamental when selecting capacitors to achieve accurate measurements.
"The performance of capacitors can not only affect the measurement but also the entire circuitry in which they are used."
Factors Affecting Capacitance Values
The capacitance values are not static; they are influenced by various factors. Among the primary factors are:
- Dielectric Material: The dielectric material within a capacitor significantly affects its ability to store charge. Each material has a specific dielectric constant that determines its efficiency.
- Temperature: Temperature variations can alter the capacitance characteristics. Most capacitors have specified temperature ranges where their performance is stable.
- Frequency: At different frequencies, capacitors can demonstrate varying impedance. This frequency dependency is important as it can skew measurements if not taken into account.
- Voltage Levels: The voltage applied to a capacitor can lead to electrical breakdown or altered performance due to dielectric stretching.
When measuring capacitance, it is essential to account for these factors. They can lead to measurement inaccuracies, potentially resulting in faulty circuit performance. Therefore, adopting a systematic approach to measuring capacitance that considers these variables is crucial for obtaining reliable results.
Capacitance Measurement Techniques
Understanding capacitance measurement techniques is fundamental for professionals and students in the field of electronics. Accurate capacitance measurement is crucial in ensuring system reliability. This section explores various techniques that help in evaluating capacitance values effectively. Each technique has its unique advantages and limitations, which are important for specific applications.
Using an LCR Meter for Capacitance Measurement
The LCR meter is a widely-used instrument for measuring inductance (L), capacitance (C), and resistance (R). Its ease of use and versatility make it a preferred choice in many environments.
- Setup: Connect the capacitor to the LCR meter ensuring proper polarity. Some capacitors may have polarity and incorrect connections can affect readings.
- Measurement: Choose the capacitance measurement mode on the meter. The display will show the capacitance value directly.
- Calibration: Check if the LCR meter requires calibration. Regular calibration ensures accuracy and consistency in results.
The LCR meter provides both AC and DC measurements, but AC measurements are more common. This is due to their ability to give more accurate readings for many capacitors.
Capacitance Bridge Method
The capacitance bridge method is a precise technique that compares an unknown capacitor with a standard capacitor. This method is advantageous for high precision measurement but requires a more complex setup.
- Components: A basic capacitance bridge consists of a standard capacitor, a null detector, and a variable capacitor.
- Procedure: Balance the bridge circuit by adjusting the variable capacitor until the null point is reached. At this point, the capacitance of the unknown capacitor can be calculated based on known values.
- Accuracy: This method can reach high precision, thus it is often employed in laboratory settings.
Careful consideration is needed when selecting components, as the quality of the instruments can impact the overall measurement accuracy.
Time-Domain Reflectometry
Time-domain reflectometry (TDR) is another advanced technique for measuring capacitance, particularly useful for assessing long cables and connections in aerospace and automotive applications.
- Principle: This method involves sending a fast pulse through a transmission line and observing the reflections. The way these reflections behave gives insight into the capacitance of the line.
- Analysis: By analyzing the time it takes for the pulse to travel and reflect, one can deduce information about the impedance, which correlates with capacitance.
- Applications: TDR is especially useful in fault detection and can locate issues like breaks or shorts in a line.
Considered state-of-the-art, TDR enables engineers to troubleshoot complex systems effectively.
AC vs. Measurement Methods
When measuring capacitance, the choice between AC and DC measurement methods can affect results significantly.
AC Measurement:
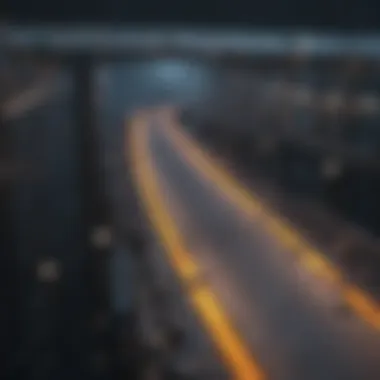
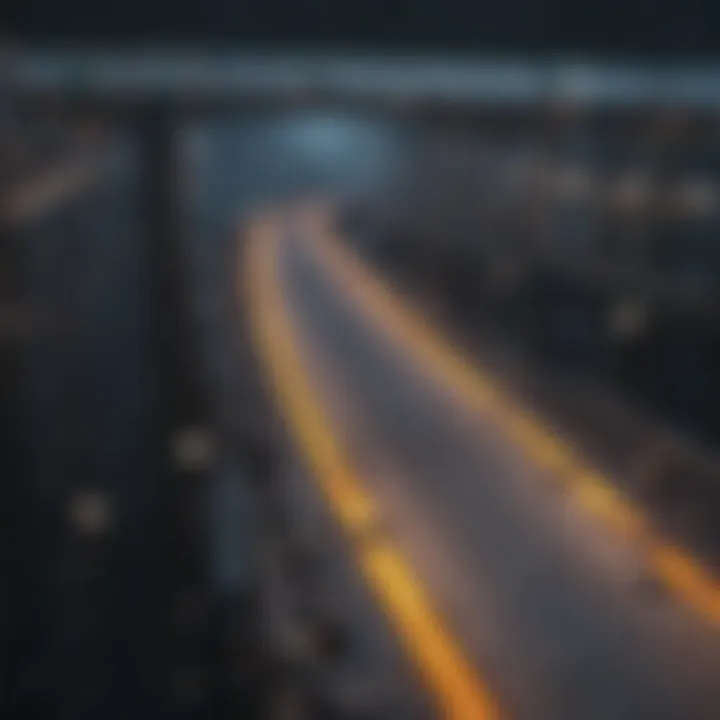
- Efficiency: Preferred in most applications due to its ability to account for capacitive reactance.
- Results: AC measurements provide a clearer picture of how a capacitor performs when alternating current is applied.
DC Measurement:
- Simplicity: Offers straightforward setups and is less dependent on frequency components.
- Limitations: Not suitable for all types of capacitors as it can charge non-ideal capacitors and alter the results.
Practical Considerations in Capacitance Measurement
When measuring capacitance, practical considerations play a significant role in ensuring accuracy and reliability. Understanding these factors can help researchers and professionals achieve precise measurements, which are crucial for effective design and troubleshooting in electrical systems. In this section, we will explore three essential elements: calibration of measurement devices, environmental factors influencing readings, and dealing with measurement noise.
Calibration of Measurement Devices
Calibration of measurement devices is among the most critical steps in capacitance measurement. The accuracy of the readings depends heavily on the calibration procedures performed on the measuring equipment, such as LCR meters and capacitance bridges.
Calibration involves adjusting the measurement device to match a known standard. This can involve using reference capacitors with well-established values. Regular calibration reduces systematic errors that might skew results.
Precision in capacitance measurement can directly impact the performance of sensitive electronics. If devices are not calibrated, inaccuracies can propagate through circuit designs, leading to potential failures or inefficient operation. Therefore, it is advisable to perform calibration periodically based on the manufacturer's recommendations not only before measurements but also after maintenance work.
Environmental Factors Influencing Readings
Environmental factors significantly influence capacitance measurements. Factors such as temperature, humidity, and electromagnetic interference can alter the readings and introduce variability.
- Temperature: Capacitors can change their capacitance value with temperature shifts. Understanding the temperature coefficient of the capacitor helps in predicting how temperature may affect measurements. It is essential to perform measurements at controlled temperatures or document temperature variations encountered during testing.
- Humidity: High levels of humidity may lead to the absorption of moisture in materials surrounding the capacitor, altering their dielectric properties. Using dry environments can minimize this risk during measurement tasks.
- Electromagnetic Interference: Electrical noise from other devices can affect capacitance measurements as well. Shielding techniques, such as using twisted-pair cables and differential measurement setups, can help mitigate these influences.
Dealing with Measurement Noise
Measurement noise is an unavoidable aspect of capacitance measurement and can heavily impact the accuracy of results. Various sources contribute to noise, including thermal or ambient electrical noise. Addressing measurement noise requires specific strategies and techniques.
- Use of Shielding: Shielding devices and cables can minimize noise interference. Employing coaxial cables for connections also helps prevent external electromagnetic interference.
- Averaging Techniques: Taking multiple measurements and calculating the average can help to smooth out the effect of noise.
- Low-Noise Equipment: Opting for low-noise measurement devices or amplifiers can significantly improve the fidelity of the measurements.
Accurate capacitance measurements are crucial for ensuring reliable operation in electrical systems.
In summary, practical considerations in capacitance measurement encompass calibration, environmental factors, and strategies to mitigate noise. Attention to these elements can greatly enhance the accuracy and reliability of measurements, which is essential for the optimal functioning of electronic systems.
Challenges in Measuring Capacitance
Measuring capacitance accurately is crucial for various applications in electrical systems. Challenges in this area can substantially affect the performance and reliability of electronic devices. Understanding these challenges helps professionals avoid common pitfalls and improve measurement accuracy. Addressing issues related to capacitance measurements not only aids in electrical design but also supports effective diagnostics and maintenance practices.
Non-Ideal Capacitor Behaviors
Capacitors often do not behave as perfect components in real-world applications. Non-ideal behaviors can significantly impact measurement outcomes. Factors such as dielectric losses, equivalent series resistance (ESR), and leakage currents play vital roles in these behaviors. These attributes can result in incorrect capacitance readings, leading to potential design flaws or equipment failure.
For instance, an ideal capacitor is expected to store energy without any lost energy. However, in practical scenarios, some energy always dissipates. Understanding these non-ideal aspects is essential for accurate measurements. Thus, testing protocols often incorporate corrections for these characteristics.
Common approaches for mitigating issues include:
- Calibration: Regularly calibrating measurement tools can help account for non-ideal behaviors.
- Operating Conditions: Measuring capacitors under conditions similar to their intended usage can give more reliable readings.
- Component Selection: Choosing high-quality capacitors with lower ESR and dielectric losses can enhance performance.
By being aware of non-ideal behaviors, professionals can take informed actions to minimize measurement errors.
Parasitic Capacitance and Its Effects
Parasitic capacitance arises from the unintentional coupling between different conductors in a circuit. This coupling can introduce additional capacitance that alters the expected readings. It is often a result of physical proximity or layout issues in circuit design and is a common challenge in high-frequency applications.
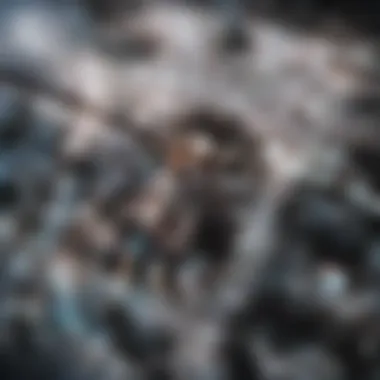
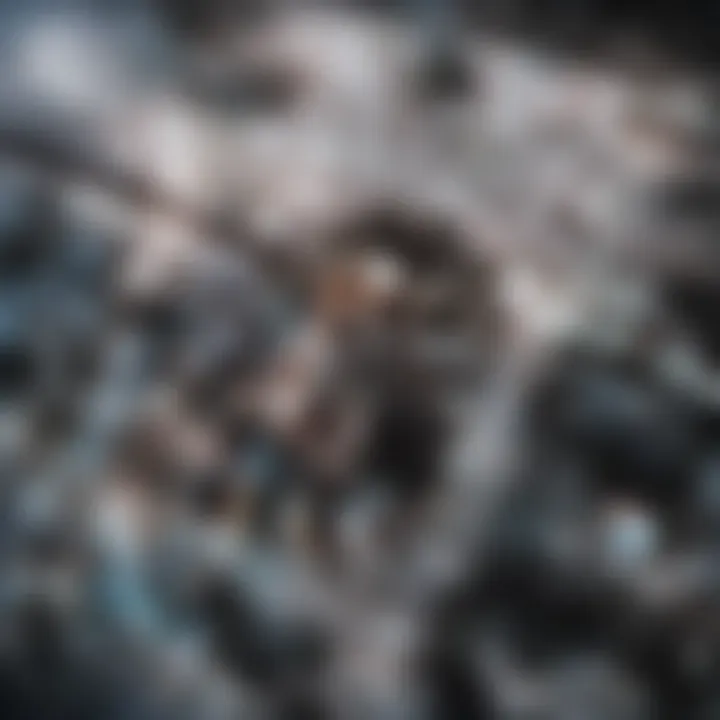
The effects of parasitic capacitance can skew measurements, especially in sensitive electronic equipment. In some cases, it may completely overshadow true capacitance values, making accurate assessment difficult.
Strategies to deal with parasitic capacitance include:
- Proper Circuit Layout: Designing printed circuit boards (PCBs) with careful attention to the distance and orientation of components can reduce parasitic effects.
- Shielding Techniques: Implementing shielding can protect sensitive components from the influence of unwanted capacitance.
- Measurement Technique Selection: Choosing appropriate methods and instruments tailored to minimize the influence of parasitic capacitance during testing is critical.
Applications of Capacitance Measurement
Measuring capacitance plays a crucial role in various fields, especially in electronics and electrical engineering. This section elaborates on the applications of capacitance measurement, detailing how it informs design decisions, maintenance practices, and ongoing research. Proper understanding of capacitance ensures more reliable and efficient systems, leading to better performance and longevity of electrical devices.
Capacitance in Circuit Design
Capacitance measurements are essential in circuit design. Designers utilize these measurements to select appropriate capacitors that meet the specific needs of a project. For instance, the capacitance value determines how a circuit responds to voltage changes and how it stores energy. If the values are not accurate, it can lead to issues such as signal distortion and reduced efficiency.
Key considerations include:
- Capacitance Tolerance: This refers to the permissible variation in capacitance from its nominal value. Understanding tolerance helps in selecting components that will operate well under expected conditions.
- Voltage Ratings: Different capacitors are rated for different maximum operating voltages. Engineers must ensure the chosen capacitors can handle peak voltages without failing.
- Temperature Coefficient: This indicates how capacitance changes with temperature. Different materials respond variably to temperature changes, influencing design choices.
The correct application of capacitance within circuit design is fundamental to achieving desired outcomes like stability and reliability.
Testing and Maintenance of Electrical Equipment
Capacitance testing is critical during the maintenance phase of electrical equipment. Regular measurements help identify potential faults before they evolve into more serious issues. This proactive approach aids in sustaining operational efficiency and preventing downtime.
Benefits of regular capacitance testing include:
- Early Fault Detection: Changes in capacitance can signal growing problems in capacitors or related components. Identifying these early can reduce repair costs.
- Increased Equipment Lifespan: Well-maintained capacitors work more efficiently, leading to a longer lifespan for both the capacitor and the equipment it supports.
- Safety Assurance: Faulty capacitors can lead to electrical hazards. Regular testing mitigates risks associated with unexpected failures.
Technicians often use tools like LCR meters to conduct capacitance tests as part of routine maintenance checks.
Implications in Research and Development
In research and development, capacitance measurement supports innovation and experimentation. Scientists and engineers explore new materials and designs, relying on accurate capacitance data to inform their hypotheses and prototypes.
Important aspects of capacitance in R& include:
- Material Development: Researchers actively investigate new materials that can lead to better capacitor performance. Understanding how capacitance varies with different substances fosters advancements in technology.
- Device Prototyping: When creating prototypes, engineers use capacitance measurements to refine their designs. These measurements help in tuning performance features to meet user needs more effectively.
- Innovative Applications: As technology evolves, capacitance measurement is crucial in developing applications such as energy storage systems and advanced electronic devices.
Accurate capacitance measurement drives progress and innovation in the electronic field.
In summary, the applications of capacitance measurement are vast. It enhances circuit design, allows for efficient testing of electrical equipment, and fosters advancements in research and development. With continued attention to measurement accuracy, engineers and researchers can contribute significantly to technological progress.
The End
The conclusion of this article serves as a pivotal point to synthesize the extensive discussions presented on measuring capacitance within electrical systems. It highlights the critical nature of accurate capacitance measurement in modern electronics as well as its various applications in circuit design, testing, and research. Understanding the limitations and challenges associated with capacitance measurements is equally important. Each methodology discussed throughout this article bears significance in practical scenarios, offering readers a clear roadmap to address specific capacitance measurement needs.
Prioritizing effective strategies ensures quality results. Factors such as calibration, environmental influences, and the handling of parasitic capacitance need careful consideration. This article also aims to underline the relevance of capacitance measurements, not only in resolving practical issues but also in enhancing the overall performance of electrical systems.
In summary, the knowledge shared within this article emphasizes the interplay between theory and practical applications. The journey of exploring capacitors, measurement techniques, and the challenges in measurement forms a comprehensive foundation for readers. Following this summary, the future directions in capacitance measurement research will bring forth new avenues for exploration and efficiency improvements.
Summary of Key Points
- Significance of Capacitance: Capacitance measurements are essential for circuit functionality, design accuracy, and diagnostic processes.
- Measurement Techniques: Different techniques, like using LCR meters and capacitance bridges, were discussed, showcasing their specific applications and benefits.
- Challenges: Measurement noise and parasitic capacitance present marked challenges that can distort results.
- Practical Considerations: Calibration and environmental factors play a significant role in measurement accuracy.
- Applications: A broad spectrum of applications was highlighted, including circuit design and maintenance.
Future Directions in Capacitance Research
The landscape of capacitance measurement is evolving. As technology progresses, several areas indicate promising advancements for research:
- Nanotechnology: The integration of capacitors at nanoscale levels opens new avenues for enhanced measurement techniques and devices.
- Wireless Measurement: Innovations in wireless technology could improve measurement accuracy while reducing interference from environmental factors.
- Smart Sensors: The development of intelligent sensors capable of real-time capacitance measurement will enhance monitoring capabilities in various applications.
- Standardization: There is a growing emphasis on establishing standardized protocols for capacitance measurements to ensure consistency across the industry.
Moving forward, researchers and practitioners within electronics will likely explore these potentials, driving improvements in measurement techniques and applications. The future holds promise in overcoming current limitations and fostering breakthroughs in capacitance measurement.