Measuring Amplitude: Importance and Methodologies
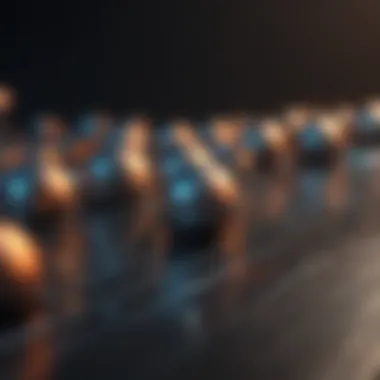
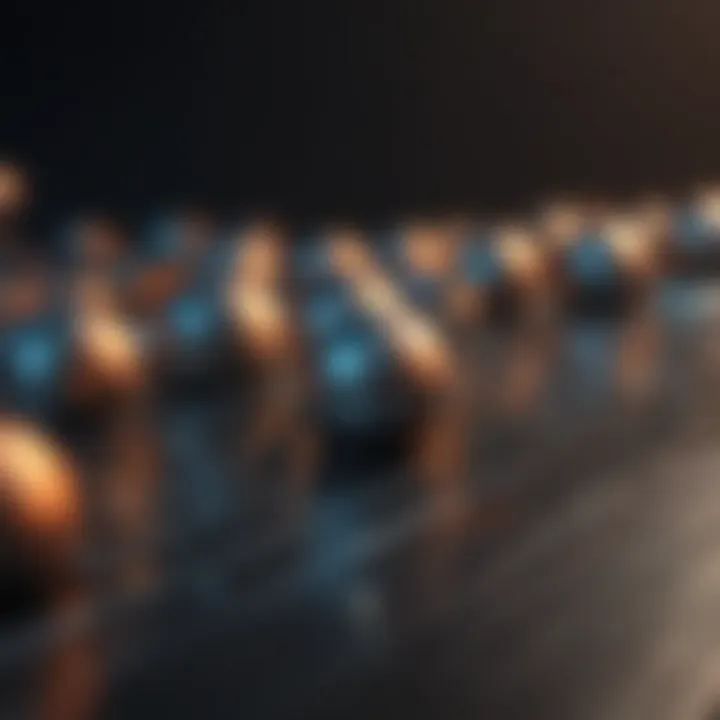
Intro
Understanding amplitude measurement is crucial across various scientific fields. This article explores its importance and the methodologies used to measure it accurately. From physics to biology, the implications of amplitude can determine everything from sound quality to signal strength.
Background and Context
Overview of the Research Topic
Amplitude refers to the extent of a change or fluctuation in a variable. In the context of physics, it often describes the height of a wave. Measuring this effectively allows scientists to assess various phenomena, such as sound waves in acoustics, electromagnetic waves in telecommunications, and even changes in brain waves in neurobiology. Each discipline has its own unique causes and implications regarding amplitude.
Historical Significance
Historically, the understanding of wave and amplitude concepts began with early experiments by figures such as Galileo and Newton. Their work laid foundational ideas that were later expanded by researchers like Thomas Young, who studied wave interference. This progress continued through the work of Heinrich Hertz, who demonstrated electromagnetic waves, showing that amplitude plays a central role in understanding modern physics.
Key Findings and Discussion
Major Results of the Study
Through the viewing lens of amplitude measurement, recent studies unveil remarkable insights in fields like medical imaging and audio engineering. Innovative techniques such as interferometry enhance measurement precision. This results in improved imaging quality in MRI technology. Furthermore, in the domain of sound engineering, proper amplitude measurement is vital for reproducing audio accurately.
Detailed Analysis of Findings
The methodology for measuring amplitude varies significantly depending on the context. For sound measurement, decibels are commonly used, providing a logarithmic scale essential for accurate assessments. In the realm of electromagnetism, oscilloscopes allow for real-time amplitude data representation, crucial for the analysis of signals.
"Accurate amplitude measurement is not merely a technical necessity; it is the cornerstone of reliable scientific inquiry."
Moreover, techniques such as Fourier analysis allow researchers to decompose complex signals, aiding in the identification of amplitude-related properties across various signals. Understanding these methodologies enriches the broader conversation regarding the implications of amplitude beyond immediate scientific inquiry.
This narrative underscores that measuring amplitude is more than an academic exercise. It bridges theory and practice across diverse applications, underscoring the importance of continuous advancements in measurement methodologies.
Prolusion to Amplitude
Understanding amplitude is fundamental for a wide range of scientific fields. Amplitude refers to the measurement of the extent of a wave's oscillation or the distance from the mean position to the peak of the wave. This is crucial in physics, engineering, biology, and other disciplines. Accurate amplitude measurements provide insight into the properties of waves and signals, enabling researchers and professionals to analyze data with precision.
The importance of amplitude cannot be understated. When sound, light, or electromagnetic waves are involved, knowledge of amplitude often determines how these phenomena are perceived or utilized. For example, in audio engineering, the amplitude of sound waves correlates with loudness, affecting how we experience music or speech. In communication systems, the amplitude is vital for signal integrity and clarity.
This article will cover various aspects of amplitude, including its definition, types, measurement techniques, and applications across different scientific disciplines. Moreover, understanding how to measure amplitude accurately is essential for reliable data interpretation and practical implementations.
Definition of Amplitude
Amplitude can be defined as the maximum extent of a vibration or oscillation, typically measured from the position of equilibrium. It fundamentally represents one of the key characteristics of waves, describing how far the wave moves from its central position. Understanding this definition is important because it lays the groundwork for further exploration into the types of amplitude and their significance in various applications.
Types of Amplitude
Different types of amplitude exist to serve various needs in measuring and analyzing waveforms. The primary types discussed include
- Instantaneous Amplitude
- Peak Amplitude
- Root Mean Square Amplitude
Instantaneous Amplitude
Instantaneous amplitude indicates the amplitude of a waveform at a specific moment in time. Its significance lies in the ability to capture transient signals that might be missed with other measurement forms. The key characteristic of instantaneous amplitude is its time-dependent nature, allowing for real-time analysis of oscillations. It is particularly beneficial for applications requiring precision in dynamic conditions, such as in monitoring electrical signals or fluid dynamics. However, because it fluctuates constantly, it may not provide an overall view of the signal's behavior.
Peak Amplitude
Peak amplitude refers to the maximum amplitude value of a wave during its cycle. It is easy to measure and is often used as a fundamental descriptor of wave strength. The utility of peak amplitude is notable in audio processing; it helps to prevent distortion by ensuring signals remain within a certain range. One unique aspect is it gives a clear indication of the wave's extreme points but can sometimes overlook lower amplitude variations that may be relevant for certain applications.
Root Mean Square Amplitude
Root mean square amplitude, often abbreviated as RMS amplitude, represents the effective level of a varying waveform. This measurement is key for understanding power in electrical systems because it correlates with the energy conveyed by the wave. RMS amplitude is advantageous as it provides a single value representing the wave's overall power, making it an attractive choice for engineers and physicists. However, it requires more complex calculations than simpler types, which can be a disadvantage if quick evaluations are needed.
The Role of Amplitude in Science
Amplitude is a crucial concept that extends beyond mere measurements; it encapsulates dynamic interactions within various scientific disciplines. Understanding amplitude allows scientists to analyze wave behaviors, assess energy levels, and interpret signal quality. This multifaceted nature makes the study of amplitude essential in physics, biology, and engineering. The significance is not just academic; it impacts technological advancements and the foundational knowledge required in these fields.
Significance in Physics
In physics, amplitude primarily pertains to wave phenomena, including sound, light, and electromagnetic waves. A greater amplitude indicates more energy and a stronger signal. For instance, in sound waves, increased amplitude relates directly to volume. This concept is fundamental in acoustics and helps explain how sound travels in different environments.
Moreover, the amplitude of electromagnetic waves is significant in understanding phenomena like radio transmission and optics. Higher amplitudes correspond to higher intensities, influencing the behavior of photons in applications like lasers and fiber optics. Therefore, understanding the amplitude is critical for physicists seeking to manipulate wave behaviors effectively in various practical scenarios.
Relevance in Biology
In biology, amplitude plays a vital role in areas such as bioacoustics and cellular signaling. For instance, studying the amplitude of vocalizations in animals provides insights into their communication strategies and social behaviors. Measuring sound amplitude helps researchers determine how various species perceive and respond to sound cues in their environment, which is essential for understanding ecological dynamics.
Additionally, in cellular biology, amplitude of signals can indicate the intensity of cellular responses to stimuli. Stronger signals can trigger more robust biological reactions, which is crucial when studying hormonal responses or neural communication. Thus, the measurement of amplitude in biological systems is important not just for understanding interactions but also for applying such knowledge in medical and technological advancements.
Applications in Engineering
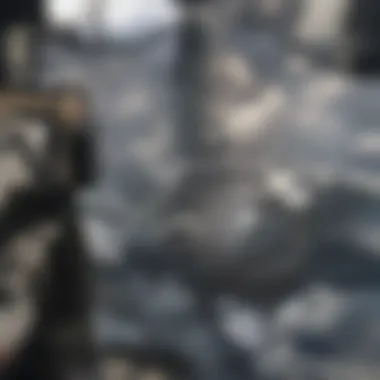
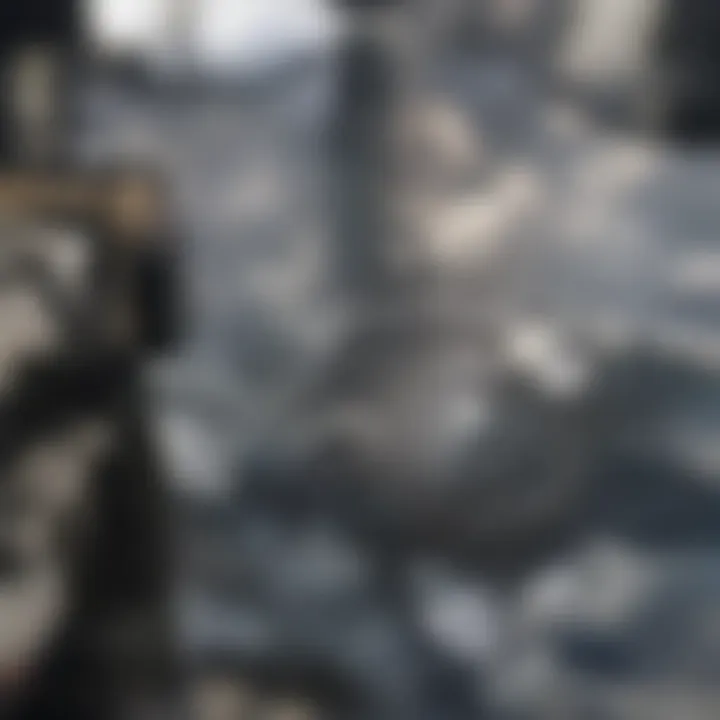
In engineering, amplitude measurement is vital across multiple domains including audio engineering, telecommunications, and structural analysis. Engineers utilize amplitude to design and optimize systems for sound and vibration control. For instance, managing audio amplitude ensures clarity in sound reproduction systems.
In telecommunications, understanding signal amplitude allows engineers to enhance data transmission. High amplitude signals can effectively transmit more data over long distances, making amplitude measurement key in developing efficient communication networks.
Moreover, amplitude plays a role in structural engineering, where the amplitude of vibrations can indicate potential structural failures. Engineers assess these measurements to ensure safety and longevity of structures under various forces.
In summary, the role of amplitude in science is multi-dimensional, influencing fundamental theories and practical applications alike. Understanding amplitude's significance across diverse fields underscores its importance in both academic inquiry and real-world problem-solving.
Fundamental Principles of Amplitude Measurement
Understanding the fundamental principles of amplitude measurement is essential for comprehending how various waveforms and signals represent physical phenomena. This section focuses on two primary concepts: waveforms and the comparison between time-domain and frequency-domain analyses. Grasping these principles not only enhances the precision of measurements but also informs the methodologies used across scientific disciplines.
Understanding Waveforms
Waveforms are graphical representations of a signal's amplitude over time. They provide a vital basis for analyzing any periodic phenomenon, be it electrical signals or sound. A waveform illustrates how the amplitude varies, delivering insights into signal characteristics such as period, frequency, and shape.
In practical scenarios, waveforms can differ significantly based on the context. For instance, the waveform of a sine wave is smooth and continuous, while a square wave oscillates sharply between high and low states. Each type of waveform behaves uniquely under certain conditions, influencing the approaches used in measurement.
Key aspects of waveforms include:
- Periodicity: Indicates how regularly a waveform repeats, essential for determining frequency.
- Amplitude Variation: Illustrates signal strength and energy, crucial for applications in fields like telecommunications.
- Shape: The shape of the waveform, whether sinusoidal, triangular, or rectangular, informs about the inherent properties of the signal.
In summary, understanding waveforms allows researchers and professionals to choose appropriate measurement techniques, leading to more accurate results in various applications.
Time Domain vs Frequency Domain
The distinction between time-domain and frequency-domain measurements is a cornerstone in amplitude measurement methodologies. Each domain offers unique perspectives on the same data, influencing the analysis and interpretation of signals.
- Time Domain: In this domain, amplitude is represented as a function of time. This approach is beneficial for observing how a signal changes temporally. It helps detect anomalies, transients, and noise. Instruments like oscilloscopes operate primarily in the time domain, displaying waveforms directly.
- Frequency Domain: Conversely, the frequency domain represents a signal by plotting its amplitude against frequency. This transformation is often achieved using the Fourier Transform. The frequency domain offers insights into the frequency components present in a signal, which is particularly useful for sound analysis, electrical signal processing, and system diagnostics.
In essence, while time-domain measurements provide immediate insights into waveform behavior over time, frequency-domain analyses uncover the underlying periodicities and harmonic content that may be hidden in time-based views. Understanding this duality is crucial for effective amplitude measurement, guiding practitioners in selecting the right tools for specific contexts.
Accurate amplitude measurement should consider both time domain and frequency domain analyses to gain a holistic understanding of signal properties.
By grasping these fundamental principles, individuals working with amplitude measurements can significantly enhance their methodological rigor and interpretative accuracy.
Techniques for Amplitude Measurement
Measuring amplitude is critical across multiple disciplines, from engineering to biology. The chosen technique directly influences measurement accuracy and the reliability of results. Understanding the variety of techniques available aids professionals in selecting the appropriate method for specific applications.
Analog Measurement Techniques
Analog measurement techniques have been around for a long time. They are often preferred for their simplicity and familiarity. Instruments like analog oscilloscopes and voltmeters are commonly used. These devices display amplitude in a straightforward, often intuitive manner. They convert the electrical signal into a visual format, allowing for easy interpretation.
Benefits of analog measurement include:
- Real-time observation: Users can see changes in amplitude instantly.
- Simplicity: They are generally easier to operate for those with basic electronic knowledge.
- Cost-effective: Analog instruments are often less expensive than their digital counterparts.
However, there are considerations to keep in mind. Analog devices can be less precise compared to digital methods. They might not capture high-frequency signals accurately. Additionally, they are more susceptible to environmental noise. This can affect the fidelity of the measurement.
Digital Measurement Techniques
Digital measurement techniques have become prevalent in modern applications. Digital oscilloscopes and software-based spectrum analyzers offer precise measurements and advanced functionalities. They convert signals into numerical data, which can be processed, stored, and analyzed easily. This enables more complex analyses that are often necessary in scientific research and technical applications.
Some key advantages include:
- Higher accuracy: Digital methods provide more consistent and reliable readings.
- Advanced processing: Data can be analyzed using various algorithms for deeper insights.
- Storage capabilities: Measurements can be saved and retrieved for later analysis.
Nonetheless, digital systems also have drawbacks. They require a more thorough understanding of signal processing. Setup can be more complicated, and there is a learning curve involved in effectively using these tools. Additionally, dependence on software and hardware increases vulnerability to malfunctions.
Optical Methods for Amplitude Measurement
Optical methods are increasingly recognized for measuring amplitude, particularly in fields like physics and engineering. Techniques such as laser interferometry and photoelasticity demonstrate how light interacts with materials to provide amplitude data. These methods can achieve incredibly precise measurements, often at micro or nanometer levels.
The benefits of optical methods include:
- Extremely high resolution: They can detect minute changes in amplitude.
- Non-destructive: Many optical techniques do not alter the character of the sample.
- Broad applicability: They are applicable in various fields, including materials science and biology.
However, factors like environmental conditions can affect results. Temperature, humidity, and vibration can introduce noise into the measurements. Moreover, the equipment can be costly and requires specific expertise to operate effectively.
"Selecting the right technique for amplitude measurement is crucial. The method impacts the accuracy, reliability, and type of analysis possible."
In the journey of understanding amplitude measurement, these techniques are foundational. A clear grasp of analog, digital, and optical approaches ensures accuracy and promotes effective application in real-world scenarios.
Tools and Instruments for Measuring Amplitude
When considering measuring amplitude, the right tools and instruments play a pivotal role. These devices facilitate accurate measurements, ensuring that the gathered data is reliable and applicable to various scientific fields. Without appropriate tools, the very essence of amplitude measurement would be compromised. The significance of these instruments extends beyond mere functionality; they contribute to advancing research, improving applications, and enhancing understanding in numerous disciplines.
Oscilloscopes
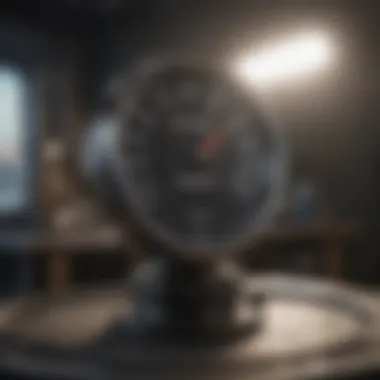
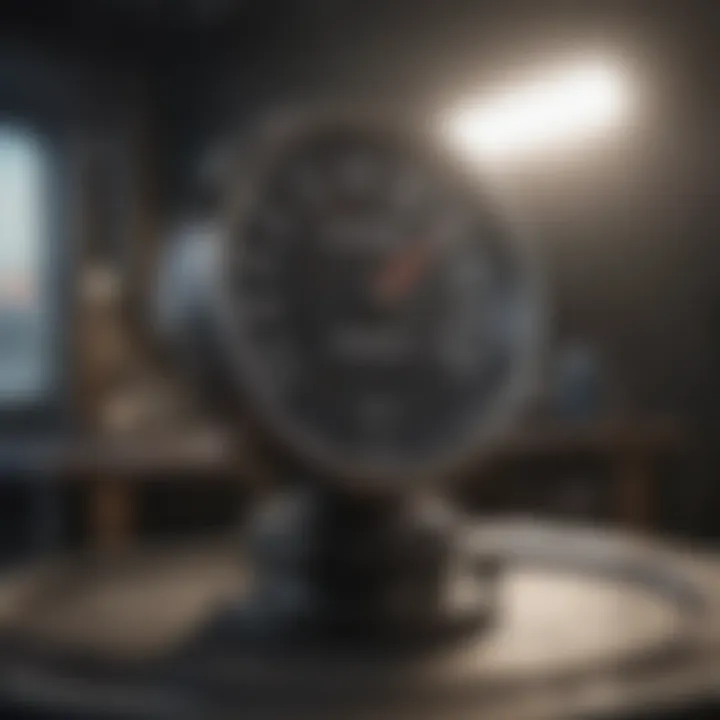
Oscilloscopes are fundamental instruments in the realm of amplitude measurement. They provide a visual representation of electrical signals over time, allowing users to observe waveforms directly. By displaying the changes in voltage as a function of time, oscilloscopes enable scientists and engineers to analyze characteristics such as amplitude, frequency, and periodicity.
One key benefit of oscilloscopes is their versatility. They can measure various types of signals, from analog to digital. Moreover, modern oscilloscopes come equipped with advanced features, such as the ability to perform mathematical functions on the displayed waveforms, including FFT (Fast Fourier Transform) analysis. This capability makes oscilloscopes valuable in applications ranging from telecommunications to automotive engineering.
Another consideration is the bandwidth of the oscilloscope, which determines the frequency range it can accurately measure. A higher bandwidth allows the measurement of higher frequency signals, which is crucial for many high-tech applications. Additionally, the sampling rate affects the resolution of the waveform. It is essential to select an oscilloscope that meets the specific requirements of the task at hand, balancing price with performance needs.
Spectrum Analyzers
Spectrum analyzers serve another vital role in measuring amplitude, primarily focusing on frequency domain analysis. Instead of displaying signals over time, they present signals as a function of frequency. This allows users to identify the amplitude of various frequency components within a given signal.
One important aspect of spectrum analyzers is their ability to detect and measure very weak signals amidst background noise. This is particularly useful in fields such as telecommunications and broadcasting, where signal integrity is paramount. A spectrum analyzer helps pinpoint problems related to signal distortion, interference, or inadequate signal levels.
When selecting a spectrum analyzer, both resolution bandwidth and dynamic range are critical factors. The resolution bandwidth affects how finely the instrument can discern between close frequency signals, while dynamic range refers to the measure of the smallest signal that can be reliably detected relative to the largest. Importantly, many modern spectrum analyzers have integrated digital signal processing capabilities, further enhancing their performance and utility.
Data Acquisition Systems
Data acquisition systems (DAS) are instrumental for recording and analyzing amplitude measurements over time. They consist of an array of components, including sensors, signal conditioning devices, and software for data analysis. DAS systems enable continuous monitoring of amplitude across various applications in scientific research, industrial processes, and environmental studies.
The primary advantage of data acquisition systems is their ability to collect and process large volumes of data in real-time. This characteristic is particularly valuable when monitoring transient events, where changes occur rapidly and must be captured promptly. Additionally, data can be recorded for extended periods, facilitating longitudinal studies and trend analysis.
While selecting a DAS, the sampling rate, number of channels, and input voltage range are significant considerations. A higher sampling rate ensures more precise timing in recording amplitude variations, while multiple channels allow simultaneous measurement of different signals. Furthermore, software analysis tools are crucial for transforming raw data into useful insights, enhancing the overall measurement process.
"The right tools and instruments can transform the method of measuring amplitude into a sophisticated practice, delivering vital insights across various scientific endeavors."
Calibration Techniques in Amplitude Measurement
Calibration is a critical step in the process of amplitude measurement. Accurate readings are essential not only for scientific research but also for engineering applications and medical diagnostics. The reliability of measurements hinges on how well instruments are calibrated. Without proper calibration, even the most sophisticated equipment can produce misleading data, leading to erroneous conclusions. This section will detail the importance of calibration in amplitude measurement as well as standard procedures commonly employed for this essential task.
Importance of Calibration
Calibration ensures that measurement tools produce results that are consistent and comparable to standard references. This is fundamental in ensuring the integrity and accuracy of amplitude measurements. Key benefits of proper calibration include:
- Enhanced Accuracy: Calibration aligns measurement instruments to known standards, increasing the precision of readings.
- Consistency: Regular calibration ensures that measurements are consistent over time, allowing for reliable comparisons across different datasets or over various time periods.
- Traceability: Established calibration procedures create a documented chain of trust back to national or international standards, enhancing the credibility of the measurements.
- Quality Control: In regulatory environments, proper calibration practices are often mandated. Non-compliance can lead to significant legal and financial implications.
In scientific and engineering contexts, the implications of uncalibrated instruments can range from minor discrepancies to critical failures. For instance, in medical imaging, inaccurate amplitude measurements can lead to misdiagnosis.
Common Calibration Procedures
Calibration can take several forms, depending on the type of instrument and the specific requirements of the measurement task. Here are some common procedures:
- Direct Comparison: This method involves comparing the instrument's output to a standard reference signal. Differences are noted and adjustments made to the instrument to align it with the known standard.
- Cross-Calibration: This technique uses multiple instruments to measure the same quantity and compares the results. It helps identify discrepancies and systematic errors in measurements across different devices.
- Software Calibration: Some modern instruments come equipped with software that can perform calibration automatically. This process usually involves inputting known standards into the software, which adjusts the instrument's settings accordingly.
- Periodic Calibration: Regularly scheduled calibration is essential to maintain measurement accuracy. Instruments should be calibrated based on their usage frequency and environmental conditions.
Regular calibration of measurement instruments is not just a best practice, it is a necessary step in ensuring data integrity across scientific fields.
Utilizing these calibration techniques can reinforce the validity of amplitude measurements and bolster trust in the data produced. Accurate measurements pave the way for reliable conclusions, facilitating advancements across multiple disciplines.
Challenges in Amplitude Measurement
The measurement of amplitude is crucial across various scientific fields and industries. However, it is not without its challenges. Understanding these challenges helps to appreciate the complexity of obtaining accurate amplitude readings. The two primary difficulties faced when measuring amplitude are noise interference and environmental factors. These issues can distort measurements significantly, leading to inaccurate results and misinterpretations.
Noise Interference
Noise interference refers to any unwanted disturbances that can alter the amplitude signals. These disturbances can originate from various sources, including electrical equipment, electromagnetic fields, and even mechanical vibrations. In measurements, noise can mask the desired signal or introduce errors, making it challenging to isolate the true amplitude of the waveform being studied.
Noise is a persistent problem in almost all fields that require amplitude measurement. For instance, in audio signal processing, background noise can affect the clarity and quality of sound recordings. To mitigate noise interference, researchers often employ filtering techniques. These can involve software algorithms that help distinguish between the desired signal and superfluous noise. Additionally, using specialized equipment designed to minimize noise, such as shielded cables and low-noise amplifiers, can enhance measurement accuracy.
Moreover, calibrating instruments for specific environments can also be helpful. Regular calibration ensures that any inherent noise characteristics of the measurement system are accounted for, resulting in more reliable readings.
Environmental Factors
Environmental factors significantly influence the accuracy of amplitude measurements. These include factors like temperature, humidity, and even atmospheric pressure. For example, variations in temperature can affect sensor sensitivity and instrument calibration. This means that amplitude readings can fluctuate simply due to changes in the environment.
Maintaining a controlled environment is essential when precision is necessary. In laboratories, controlled conditions can be achieved through climate control systems that stabilize temperature and humidity levels. In field applications, understanding local environmental conditions can lead to better planning and execution of measurement tasks.
It is also important to consider how the location of measurements influences the results. For instance, conducting amplitude measurements near large sources of electromagnetic radiation may lead to significant inaccuracies. Therefore, selecting appropriate locations for measurements can help minimize the impact of environmental factors.
Understanding and addressing these challenges in amplitude measurement is essential for researchers and professionals. It not only improves the reliability of results but also enhances the credibility of the findings.
Advanced Amplitude Measurement Techniques
In the realm of amplitude measurement, utilizing advanced techniques is critical to obtain accurate and reliable data. These methods often surpass traditional measurement approaches, especially when addressing complex waveforms or when the signals are deeply embedded in noise. Advanced techniques assist researchers and professionals in extracting meaningful information that conventional techniques might miss.
When it comes to amplitude measurement, precision is key. Advanced amplitude measurement techniques enhance sensitivity and provide clarity, which are crucial in fields like seismology, audio processing, and medical imaging. Both the Fourier Transform and Wavelet Transform stand out as significant methodologies in this category, each offering unique advantages.
Fourier Transform Techniques
The Fourier Transform technique has proven to be an essential tool in analyzing waveforms. By converting time-domain signals into frequency-domain representations, the Fourier Transform allows for deeper insights into the signal characteristics. This approach is particularly beneficial in identifying the frequency content of signals, enabling researchers to discern patterns and assess the amplitude effectively.
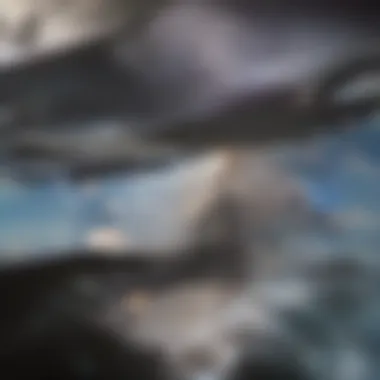
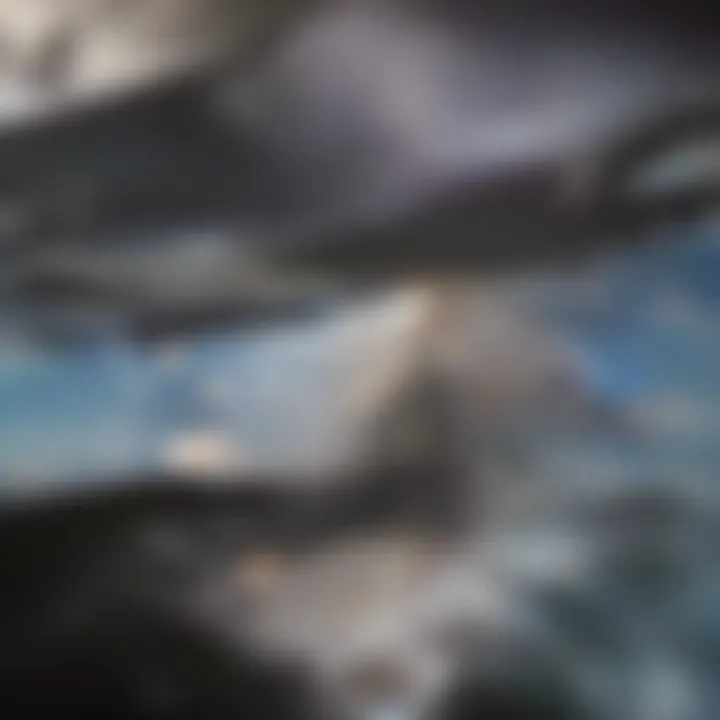
One of the leading advantages of this technique is its ability to isolate specific frequencies. In applications such as sound engineering, the Fourier Transform simplifies the process of filtering unwanted noise or emphasizing certain sound frequencies. Its application can further extend into image processing and telecommunications, where understanding frequency content can inform modulation techniques.
Key considerations when applying the Fourier Transform include:
- Windowing: Choice of window functions can significantly affect results.
- Aliasing: Care must be taken to prevent loss of information due to inadequate sampling rates.
- Computational Resources: Depending on signal complexity, the processing may require substantial computational power.
A common implementation is done using software tools that incorporate the Fast Fourier Transform (FFT) algorithm to expedite calculations. Here is a simple Python example of performing a Fourier Transform using NumPy:
Wavelet Transform Applications
The Wavelet Transform is another advanced method that operates differently from the Fourier Transform. It provides both time and frequency information simultaneously. This dual capability allows for high-resolution analysis of signals that exhibit transient or non-stationary behavior.
This technique is notably beneficial in scenarios involving finite or short-duration signals, such as seismic data and biomedical signals. Unlike Fourier analysis, Wavelet Transform adapts to the signal's characteristics at various scales, making it a powerful tool for signals that vary rapidly over time.
Some key applications include:
- Seismic Analysis: Helps in interpreting geological structures based on wave propagation.
- Image Compression: Enables enhancements in image data without significant loss of information.
- Noise Reduction: Effectively separates useful signals from noise in medical imaging and audio signals.
Adopting Wavelet Transform requires consideration of the chosen wavelet basis, the scale of analysis, and the boundary effects that can affect results. In practice, selecting the right wavelet can significantly influence the quality of the data interpretation.
Real-World Applications of Amplitude Measurements
Understanding amplitude measurement is crucial in many fields. From geology to music, amplitude plays a significant role in various applications. This section elaborates on how amplitude measurements are applied in real-world scenarios, emphasizing their benefits and considerations.
Seismic Amplitude Measurement
In seismology, measuring the amplitude of seismic waves is vital for assessing the strength of earthquakes. The amplitude can indicate the energy released during an event. Seismographs are used to capture these waves. By analyzing the amplitude, scientists can determine the earthquake's magnitude and its potential impact on structures.
This application directly influences public safety and engineering standards. For example, areas prone to earthquakes might use amplitude measurements to develop better building codes. Accurate earthquake predictions and assessments can help mitigate damage and save lives. Additionally, amplitude data can assist in understanding tectonic movements, which is crucial for future planning and risk management.
Audio Signal Processing
In audio technology, amplitude is a core component of sound waves. Audio engineers use amplitude measurements to manipulate sound quality in recordings. For instance, mixing and mastering processes rely heavily on controlling the amplitude of different audio tracks. Adjusting the amplitude can enhance clarity and balance within a soundscape.
Moreover, amplitude modulation is essential in radio broadcasting. It allows the transmission of audio over long distances by varying the amplitude of the carrier wave. This method ensures better sound quality and reception. Understanding amplitude helps audio professionals create a more engaging listening experience while maintaining the integrity of the sound.
Medical Imaging Techniques
Amplitude measurements are also critical in medical imaging, particularly in ultrasound. This technique uses sound waves to create images of organs inside the body. The amplitude of reflected sound waves determines the brightness of the image produced. Higher amplitudes indicate denser tissues, which can assist in diagnosing various medical conditions.
Beyond ultrasound, other imaging techniques, such as MRI, also consider amplitude. Analyzing signal amplitude helps in obtaining clearer images for better diagnosis. This application illustrates the importance of amplitude measurement in healthcare, emphasizing the need for precision in medical diagnostics and the potential to improve patient outcomes.
Accurate amplitude measurement in medical imaging can significantly enhance diagnostic capabilities, impacting patient care considerably.
Real-world applications of amplitude measurement extend beyond these examples. Each application not only demonstrates the utility of amplitude in mitigating risks and enhancing technologies but also highlights the need for precision across various fields. Understanding these applications provides insight into why accurate amplitude measurement remains vital in our daily lives.
Future Trends in Amplitude Measurement
The exploration of amplitude measurement continues to evolve. New methodologies and technologies are emerging regularly. This section discusses important trends shaping the future of amplitude measurement. Understanding these trends is valuable for researchers and practitioners alike. They may impact data accuracy, efficiency, and the overall development of various scientific fields.
Emerging Technologies
Recent advancements in technology are influencing amplitude measurement significantly. Some of these include:
- Laser Interferometry: This technique measures amplitude with high precision using laser beams. It is less susceptible to environmental interferences than traditional methods.
- Nanotechnology: The applications of nanotech in sensors have allowed for miniature devices that can measure amplitude at a molecular or atomic level. These sensors will be crucial in biomedical applications.
- Quantum Sensors: These are at the forefront of scientific research. They enhance measurement precision by leveraging quantum mechanics. As this field matures, we may see more practical applications in amplitude measurement.
The integration of these technologies leads to better data collection, enhancing our ability to analyze complex phenomena.
Influence of Artificial Intelligence
Artificial Intelligence (AI) is starting to play a significant role in amplitude measurement. AI algorithms can analyze vast amounts of data quickly. They can identify patterns that are often missed by human operators. Benefits of incorporating AI in amplitude measurement include:
- Real-time Analysis: AI enables the immediate processing of amplitude data. This is particularly useful in applications like audio processing and seismic activity detection.
- Predictive Analytics: Through machine learning, AI can predict potential amplitude variations. This feature improves preparedness in fields like disaster management and engineering.
- Anomaly Detection: AI algorithms can automatically highlight unusual amplitude readings. This improves diagnostics in medical imaging and other areas.
Integrating AI in amplitude measurement not only enhances efficiency but also opens new pathways for innovation.
As both technologies advance, we can expect significant improvements in the reliability and applicability of amplitude measurements across various fields. This alignment promises to enhance the insights obtained from measurements and broaden the scope of applications.
Closure
The conclusion section serves as a vital component of this article on amplitude measurement. It synthesizes the information discussed and reaffirms the importance of understanding amplitude and its measurement across various disciplines. A clear conclusion allows readers to grasp the essence of the entire discourse and emphasizes the relevance of accurate amplitude measurement in modern science and technology.
Summary of Key Points
This article presented a comprehensive overview of amplitude measurement, covering essential definitions, types, and methodologies used in various fields. Key points include:
- Definition of Amplitude: Understanding its fundamental meaning acts as the foundation for further exploration.
- Types of Amplitude: Awareness regarding instantaneous, peak, and root mean square amplitude highlights different measurement perspectives.
- Roles in Science: The significance of amplitude in physics, biology, and engineering showcases its multidisciplinary importance.
- Measurement Techniques: A distinction between analog, digital, and optical techniques provides insight into practical approaches used today.
- Tools and Instruments: Discussing tools like oscilloscopes and spectrum analyzers informs readers about essential equipment in amplitude measurement.
- Calibration Techniques: This emphasizes the need for accuracy and consistency, vital for reliable measurements.
- Challenges and Solutions: Knowledge of common challenges such as noise interference aids in developing better measurement strategies.
- Advanced Techniques: Technologies such as Fourier and Wavelet transforms illustrate cutting-edge methodologies in this domain.
- Real-World Applications: Practical uses in seismic measuring, audio processing, and medical imaging highlight the implications of amplitude measurement.
- Future Trends: Discussion of emerging technologies and AI serves to inspire ongoing innovation in measurement techniques and applications.
The Implications of Accurate Amplitude Measurement
Accurate amplitude measurement carries significant implications across various scientific and engineering domains. It not only ensures the reliability of data but also enhances the quality of research and development processes. Key implications include:
- Data Integrity: Precise amplitude measurements contribute to the integrity of experimental results, which is crucial in scientific research.
- Innovations in Technology: As measurement techniques improve, so do the capabilities of technology, leading to advancements in fields such as telecommunications and audio engineering.
- Safety and Reliability: In applications like medical imaging and seismic assessments, accurate measurements are vital for safety and informed decision-making.
- Research Advancements: Enhanced measurement capabilities facilitate more detailed investigations, stimulating innovation and development in various sectors.