Interferometry Microscopy: Unraveling the Microscopic World
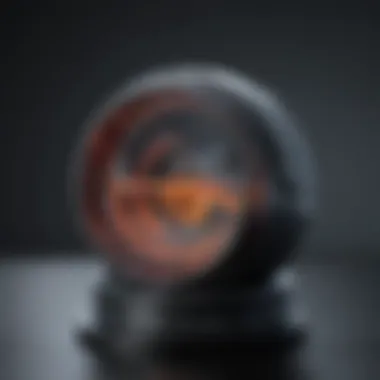
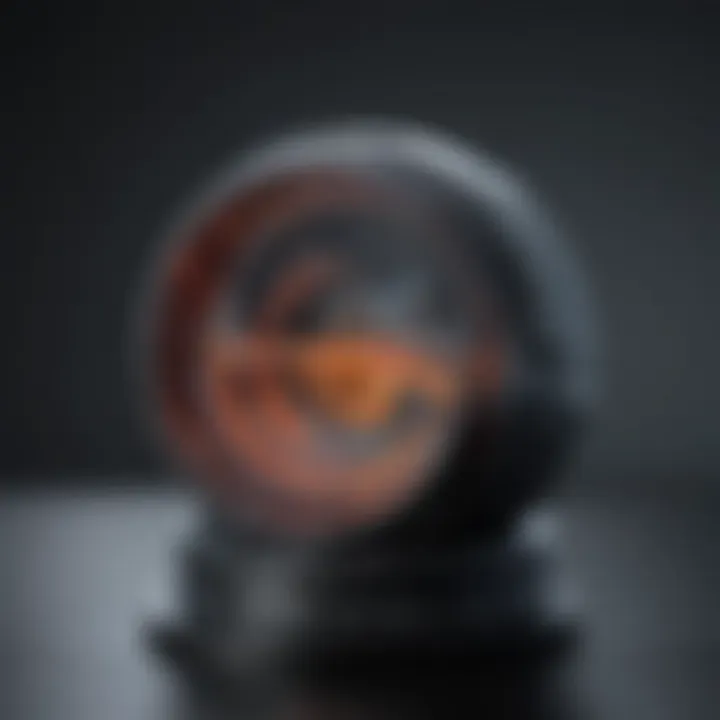
Intro
Interferometry microscopy represents a unique confluence of physics and biology, allowing scientists to visualize structures at a microscopic level. This technique leverages the principles of interferometry to achieve high-resolution imaging of samples, providing insights into both biological and material phenomena. The growing interest in this technology is not only due to its advanced imaging capabilities but also for its applications across various fields, including medicine, materials science, and nanotechnology.
This article aims to provide an in-depth exploration of interferometry microscopy, addressing both the theoretical underpinnings and practical applications. By highlighting the essential principles and methodologies used in this field, we will elucidate the advantages and disadvantages compared to traditional imaging methods.
The following sections will guide readers through the nuances of the technique, offering a comprehensive understanding of why interferometry microscopy is crucial in contemporary scientific research.
Intro to Interferometry Microscopy
Interferometry microscopy stands as a pivotal technique in the analysis of microscopic structures. It combines the principles of wave interference with microscopy to offer insights into the morphology and material characteristics of samples. Understanding this discipline is essential for researchers and practitioners in both biological and material sciences. The accuracy and sensitivity of this method allow for detailed imaging and analysis, which opens avenues for advancements in various fields.
Definition and Overview
Interferometry microscopy can be defined as a technique that utilizes the interference of light waves to create images of transparent samples. When light waves overlap, they can produce patterns of light and dark areas. These patterns arise due to differences in the optical path length, which correlate with the physical properties of the object being examined. This method enables the visualization and measurement of structures at resolutions that typically surpass those achievable with conventional microscopy techniques. Various setups, like optical setups based on Michelson and Mach-Zehnder configurations, are commonly utilized to achieve the desired interference patterns.
Historical Development
The roots of interferometry can be traced back to the 19th century. The early developments began with pioneers like Augustin-Jean Fresnel and Joseph-Louis Lagrange, who explored wave optics. However, significant advancements in interferometry microscopy emerged in the 1950s with the work of researchers like H. K. Wong. These individuals demonstrated that wave interference could be harnessed for microscopy. The ensuing years saw rapid technological progress, especially with the advent of lasers in the 1960s, which drastically improved interference microscopy techniques. The introduction of digital detectors and computing facilitated further advancements in image processing, allowing for high-resolution imaging of complex biological and materials science structures.
"Interferometry has not just advanced our technical capabilities; it fundamentally altered our understanding of microscopic phenomena."
As researchers continue to refine techniques and explore new applications, the relevance of interferometry microscopy today is more pronounced than ever. Its historical evolution underscores the significance of merging technology with fundamental principles in science. The journey from early wave optics to the sophisticated devices used today illustrates an ongoing commitment to unraveling the complexities of the microscopic world.
Fundamental Principles of Interferometry
Understanding the Fundamental Principles of Interferometry is crucial for grasping the full potential of interferometry microscopy. This section explores the core concepts that underpin this innovative technique, examining how these principles enable enhanced imaging capabilities and precise measurements in a wide variety of applications. These foundational elements not only advance scientific exploration but also inform the design and operation of interferometric systems.
Wave Interference Concepts
Wave interference is a pivotal concept in interferometry microscopy. It refers to the phenomenon that occurs when two or more waves overlap. This overlap can produce a resultant wave that exhibits new patterns of intensity and phase. The constructive interference happens when waves align perfectly, amplifying their effect, while destructive interference occurs when waves are out of sync, diminishing their impact.
In the context of interferometry microscopy, these interference patterns can provide valuable insights into the sample being analyzed. As light reflects off different surfaces within a specimen, the variations in path lengths result in different phases. Interferometers capture these variations, translating them into visual information that can be analyzed quantitatively.
The ability to measure tiny shifts in these interference patterns allows researchers to determine various properties of the sample. This might include measurements of distance, thickness, and refractive index, which are crucial for diverse fields like biology and materials science.
Coherence and Its Role
Coherence refers to the correlation between the phases of waves at different points in space and time. In interferometry microscopy, the coherence of the light source is significant because it determines the clarity and contrast of the interference patterns observed. There are two types of coherence: temporal and spatial.
- Temporal coherence describes how consistent the phase of a wave remains over time. A light source with high temporal coherence will produce a stable wave pattern, resulting in clearer interference fringes.
- Spatial coherence concerns the correlation between light at different points across a beam’s cross-section. High spatial coherence ensures that the waves can interfere with one another, producing sharp and defined fringes.
Having appropriately coherent light sources, such as lasers, is vital for achieving high-resolution images in interferometric microscopy. Without ample coherence, signal noise may obscure the fine details of the sample, hindering accurate analysis. Thus, optimizing coherence is essential for extracting precise data and making valid scientific conclusions.
The clarity of interference patterns is directly related to the coherence properties of the light used in interferometry. Clear patterns lead to better measurement accuracy and provide more reliable results.
In summary, the principles of wave interference and coherence form the backbone of interferometry microscopy. These concepts not only facilitate innovative imaging techniques but also expand the horizons for research applications across various scientific domains. As technology advances, further refinement of these principles is expected to yield even greater advancements in microscopy capabilities.
Technical Foundations of Interferometric Microscopy
Understanding the technical foundations of interferometric microscopy is crucial for leveraging its full potential. This section delves into the core concepts that make this microscopy method distinct from others. By grasping these fundamentals, one can appreciate both the capabilities and limitations of this technique. The focus here is on optical path differences and the functionality of detectors, pivotal elements in the realm of interferometric microscopy.
Optical Path Differences
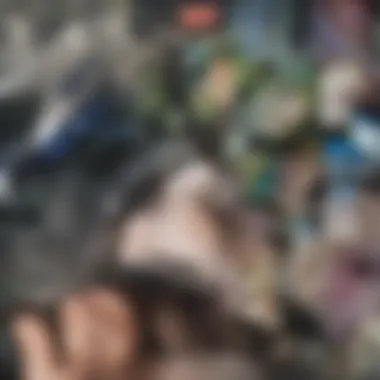
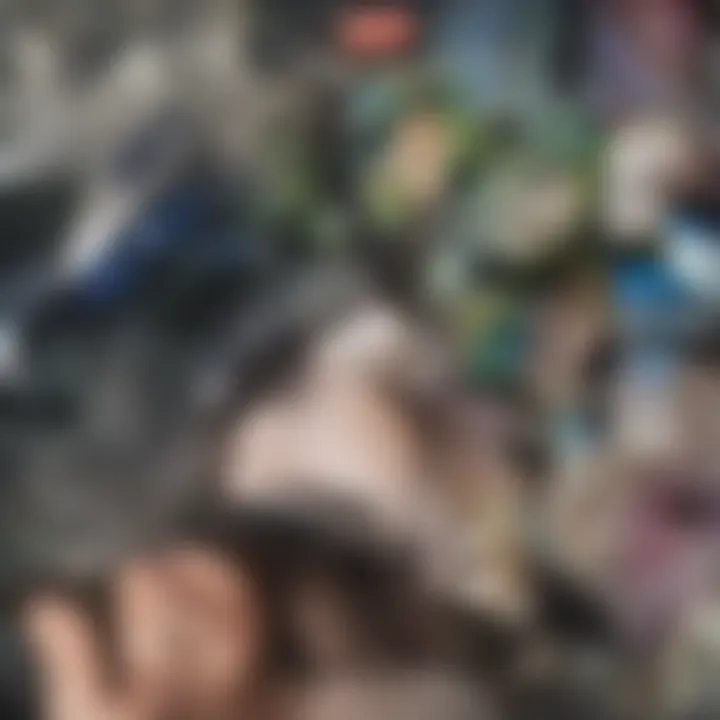
Optical path difference (OPD) is the cornerstone of interferometry. It fundamentally represents the difference in the distance light travels in two different paths before they combine. In interferometric microscopy, measuring OPD allows researchers to extract critical information about the sample being studied.
When two light waves meet, they interfere. This can cause amplification or cancellation of light depending on their phase difference. The phase difference arises from the OPD and is essential for generating the interference patterns that form the basis of this microscopy technique.
- Importance of OPD in Imaging: OPD directly influences the resolution and accuracy of measurements. A precise understanding permits clearer imaging and quantification of topographic features.
- Impact on Biological Samples: In biological imaging, OPD can help explore microscopic structures like cell membranes and organelles. These subtle details can be crucial for understanding functionality and health at the cellular level.
- Technical Constraints: Variations in environmental conditions such as temperature and pressure can influence OPD. Instruments must be calibrated to mitigate these effects, ensuring reproducibility and reliability in data.
Detectors and Their Functionality
In the framework of interferometric microscopy, detectors play a vital role in capturing and transforming optical signals into measurable data. Various types of detectors exist, each offering unique advantages and limitations.
- Types of Detectors:
- Functionality: The primary function of any detector is to convert light into an electrical signal. This signal can be analyzed and processed to produce visual representations of microscopic structures. The choice of detector influences the resolution, sensitivity, and speed of the imaging process.
- Integration with Technology: Modern interferometric microscopes often incorporate digital signal processing to enhance image quality. This integration allows for more sophisticated imaging techniques and improved real-time analysis of samples.
- Charge-Coupled Devices (CCDs): These detectors are widely used due to their high sensitivity and ability to capture detailed images.
- Photomultiplier Tubes (PMTs): Known for their strong amplification of signals, PMTs are useful in low-light conditions.
- Avalanche Photodiodes (APDs): These detectors are optimal for time-sensitive applications due to their fast response time.
Types of Interferometric Microscopy Techniques
Understanding the different types of interferometric microscopy techniques is crucial for researchers and professionals in the field. Each method has unique features and applications that cater to specific study requirements. This section explores two prominent techniques: Full-Field Interferometry and Phase-Shifting Interferometry. Both these methods enhance imaging capabilities, allowing for comprehensive analysis of biological and material specimens.
Full-Field Interferometry
Full-Field Interferometry is a technique that provides simultaneous imaging of an entire field rather than a point-by-point approach. This method enables rapid acquisition of images, which is especially beneficial for observing dynamic processes in live cells and biological samples. The full-field nature helps in reducing motion artifacts that may occur due to sample movement during imaging.
Key components of Full-Field Interferometry include the use of a coherent light source and a beam splitter, which divides light into two paths: one directed at the sample and the other at a reference mirror. The light waves later combine, and interference patterns are observed. The resulting patterns provide valuable information about surface topography and phase changes of the examined material.
Benefits of Full-Field Interferometry:
- Speed of imaging: Fast data acquisition for both static and dynamic events.
- Comprehensive data collection: Captures an entire field, reducing the time required for analysis.
- High resolution: Capable of resolving fine structures in samples.
Phase-Shifting Interferometry
Phase-Shifting Interferometry is another significant technique used in interferometry microscopy. Unlike Full-Field Interferometry, this method relies on controlling the phase of the reference beam using multiple measurements, allowing for precise depth information about the sample. This technique is adept at quantifying small variations in optical path length, which is critical for applications where minute changes must be detected and analyzed.
In Phase-Shifting Interferometry, a sequence of images is captured as the phase of the reference beam is altered. The changes in phase yield interference fringes, from which highly accurate measurements of the sample can be derived. This technique is particularly useful in fields like material science, where assessing surface quality and defects is paramount.
Considerations when using Phase-Shifting Interferometry:
- Depth resolution: Provides detailed depth measurements which are crucial for understanding sample structures.
- Complex data processing: Requires several images and sophisticated algorithms for data interpretation.
The choice between Full-Field and Phase-Shifting Interferometry often depends on the specific requirements of the study, such as the type of sample, the desired resolution, and the speed of analysis needed.
"Each interferometry technique offers unique advantages. Understanding these differences is key to selecting the right method for your research."
In summary, both types of interferometric microscopy techniques hold significant importance in advancing our understanding of microscopic phenomena. Researchers must consider the characteristics and applications of each method to maximize the effectiveness of their studies.
Applications of Interferometry Microscopy
Interferometry microscopy holds significant importance in numerous scientific fields, particularly in biological imaging and material science investigations. Its capacity to provide high-resolution images and perform quantitative measurements is a distinct benefit in research and development. This section will consider the applications in both biology and material science, which underscore the power and utility of this sophisticated imaging technique.
Biological Imaging
Biological imaging utilizes interferometry microscopy to explore cell structures, dynamics, and behaviors. This approach is increasingly recognized for its precision and ability to deliver detailed information about biological systems. The key characteristics of this imaging technique include non-invasive analysis and the capacity to visualize fine structures in real-time, which are critical aspects for various research goals.
Cell Morphology Analysis
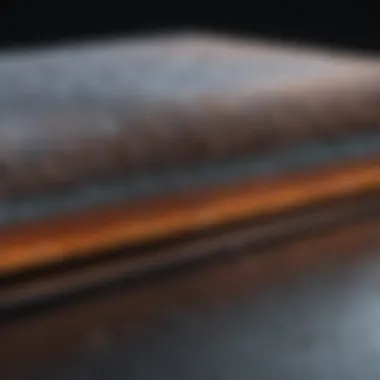
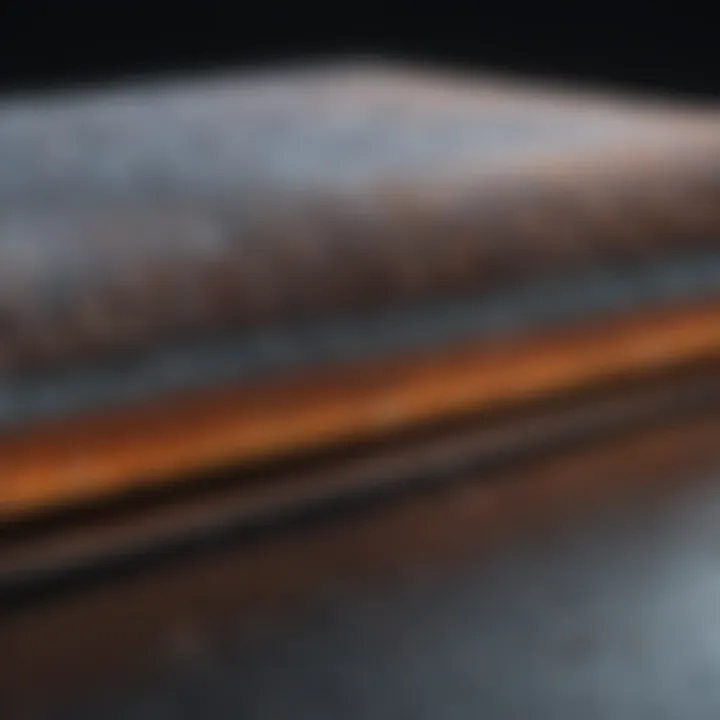
Cell morphology analysis focuses on the shape and structure of cells. This method is essential for understanding the functionality and health of cells in both research and clinical settings. A significant reason for its popularity is its ability to produce intricate details that traditional methods cannot achieve. For example, it can reveal subtle changes in cell shapes that may indicate pathological conditions, such as cancer.
The unique feature of this analysis is that it can be performed without labeling or altering the cells, thus preserving their natural state. This non-invasive aspect allows researchers to study live cells over time, providing critical insights into their behavior and interactions. However, a challenge of this method may include the complexity of data interpretation, which requires a skilled understanding of the results.
Live Cell Imaging
Live cell imaging refers to the observation of living cells in their natural environment over time, allowing for the investigation of dynamic processes such as cell division and migration. The key characteristic here is the ability to capture events in real-time, which serves as a major advantage over static imaging methods.
The unique feature of live cell imaging is its capacity to monitor changes at the cellular level without disruption. This ability provides a clear understanding of biological processes as they unfold. However, the necessity for maintaining optimal conditions for the cells throughout the imaging process poses a practical challenge. There is often a limit to the duration and specificity of what can be observed, which can impact findings.
Material Science Investigations
Material science utilizes interferometry microscopy to assess and analyze the properties and structures of materials at very small scales. The resolution and quantitative capabilities of this technique make it invaluable for understanding material behavior and properties, thus fostering innovation in material development.
Surface Topography Assessment
Surface topography assessment evaluates the surface characteristics of materials, including roughness, texture, and morphology. This technique's merit lies in its detailed visualization, which is crucial for applications in various industries such as electronics and coatings. Understanding surface properties helps optimize material performance.
A unique feature of surface topography assessment is its ability to provide topographical maps with high precision. This capability is vital for ensuring materials meet specifications for specific applications. However, interpreting surface topography data can be complex, as surface features may be influenced by numerous factors, including environmental conditions.
Stress and Strain Mapping
Stress and strain mapping involves measuring how materials deform under stress, which is critical for evaluating material performance and integrity. This method is beneficial in the fields of engineering and manufacturing, where materials must withstand various forces. Understanding stress distribution can lead to better design and failure prevention in structures.
A unique feature of stress and strain mapping is the capability to visualize stress concentrations and patterns that may not be otherwise observable. This characteristic aids in predicting points of failure and enhancing material design. However, a limitation of this technique is that it often requires controlled environments to produce accurate results, complicating data collection in real-world scenarios.
Interferometry microscopy serves as a bridge between theoretical understanding and practical applications across various fields, advancing both research and technology.
In summary, the applications of interferometry microscopy in biological imaging and material science highlight its versatility and significance. Each application benefits from unique features of the technique, providing valuable insights while also facing specific challenges to their implementation and interpretation.
Comparative Analysis with Traditional Microscopy
Interferometry microscopy represents a significant advancement in imaging techniques compared to traditional microscopy. Understanding the strengths and weaknesses of each method is crucial for researchers and practitioners who choose the appropriate tool for their specific applications. This analysis focuses on two major aspects: resolution capabilities and quantitative measurements.
Resolution Capabilities
In terms of resolution, interferometry microscopy excels through its ability to detect minute differences in optical path length. Unlike traditional optical microscopy, which is often limited by diffraction, interferometric techniques can achieve higher resolution imaging.
- Interferometric Techniques: Techniques such as phase-shifting interferometry can provide sub-wavelength resolution.
- Comparison with Optical Microscopy: Traditional optical systems may struggle at the nanoscale, while interferometric approaches allow for more detailed scrutiny of samples.
- Practical Implications: This enhanced resolution is particularly valuable in biological studies where cellular components require precise identification.
The capacity for higher resolution translates to superior imaging of fine structures. This can help scientists distinguish between cellular morphologies that might appear similar under less sensitive microscopy methods.
"In the competitive landscape of microscopy, resolution can define the successful identification and characterization of subjects under study."
Quantitative Measurements
Quantitative measurements in interferometry microscopy are fundamental to its utility in scientific research. The technique allows for precise calculations of sample characteristics, such as displacement, refractive index changes, and surface contours.
- Phase Measurements: Interferometry can measure phase changes corresponding to physical properties of samples.
- Data Acquisition: This data is then translated into quantitative metrics that aid in studying physical and biological phenomena.
- Benefits Over Traditional Methods: Traditional quantitative methods, while useful, often lack this level of precision and can be more prone to subjectivity.
Utilizing interferometry microscopy, researchers can obtain data that offers not just qualitative insights but also rigorous quantitative analysis. By providing numeric data alongside visual representations, the technique supports a more comprehensive understanding of microscopic structures.
In summary, the comparative analysis of interferometry microscopy with traditional microscopy reveals distinct advantages in terms of resolution and quantitative measurements. These aspects are essential for advancing research and development in both biological and material sciences.
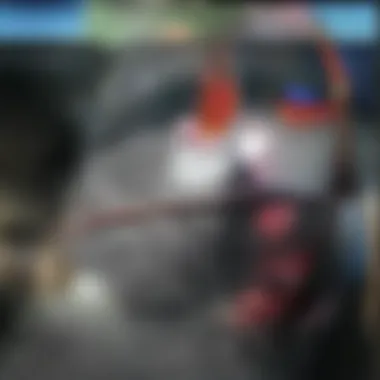
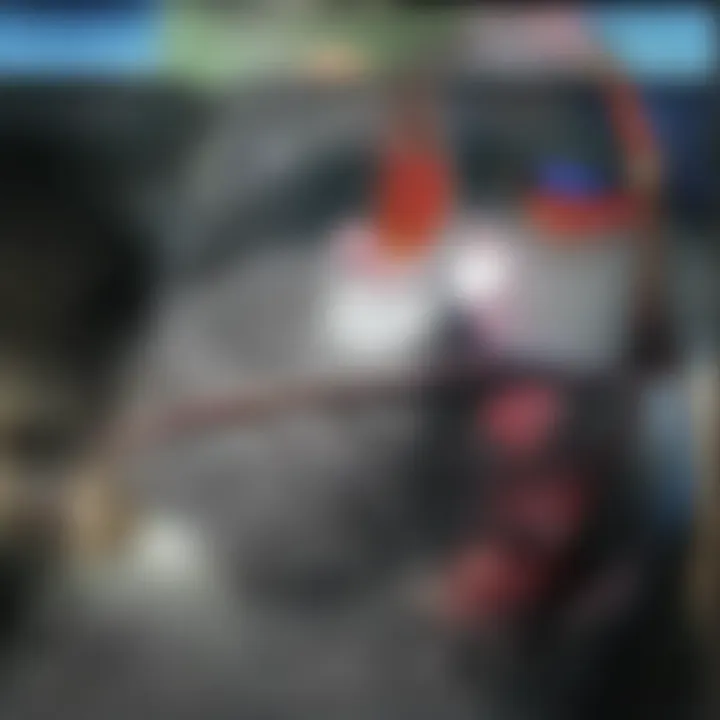
Challenges and Limitations
The domain of interferometry microscopy, while promising, is not without its challenges and limitations. A comprehensive understanding of these factors is essential for researchers and practitioners. Recognizing the inherent constraints allows for a more nuanced application of the technology and informs future advancements. Discussing both instrumental constraints and sample preparation difficulties provides insight into the complexities that affect experimental outcomes.
Instrumental Constraints
Interferometry microscopy relies on precise optical systems. One significant issue is the sensitivity to environmental disturbances. Factors like vibrations, air turbulence, and temperature fluctuations can substantially affect measurements.
Moreover, the hardware itself poses limitations. For example, the resolution and sensitivity of detectors dictate the quality of the images obtained. Some interferometric setups may only achieve certain resolutions when used under optimal conditions. If the system is not calibrated correctly, results can vary widely, making reproducibility a challenge. In some cases, the cost of high-quality components may limit access for certain research facilities, impacting the generalization of results across diverse environments.
Sample Preparation Difficulties
Sample integrity is crucial in interferometry microscopy. Proper sample preparation can be demanding. Biological samples are often subject to destruction or alteration when prepared inadequately. Consequently, careful consideration of the preparation techniques used is critical. Different materials require specific methods, and the choice can significantly affect the results.
For instance, minimizing the thickness of a sample is crucial to avoid excessive optical path differences that can lead to misinterpretations.
Additionally, some materials cannot withstand certain chemical treatments, limiting the options for preparation. This can lead to inconsistencies in results, as different protocols may yield varying outcomes for the same sample type.
Overall, the challenges related to instrumentation and sample preparation need careful management. Advances in technology can mitigate some of these issues, but it is important for practitioners to remain aware of limitations that could impact the fidelity of their conclusions.
Future Prospects of Interferometry Microscopy
Interferometry microscopy holds immense potential for future advancements in science and technology. The continuing evolution of this field is crucial for various reasons. First, as our understanding of biological and material phenomena deepens, the demand for high-resolution imaging techniques will likely increase. Interferometry microscopy can fill this gap, offering insights that are vital for researchers and industry professionals alike.
Although current applications are remarkable, there is much room for improvement. Interferometric techniques can be optimized for better resolution and throughput. These advancements will not only improve current functionalities but also expand the scope of research that can be conducted using these techniques. As researchers face increasingly complex biological structures or materials with unique properties, the adaptability of interferometry microscopy will be a key asset.
Additionally, the ongoing miniaturization of optical components and improvements in computational imaging techniques will enable more portable and efficient instruments. This could democratize access to high-quality microscope imaging, allowing a broader range of professionals to utilize these tools for scientific, educational, or industrial purposes.
Technological Advances
In the realm of technological advancements, improvements are already being seen in detectors and imaging systems. Innovations like high-speed cameras and advanced algorithms for image processing play a significant role. These technologies will enable faster data acquisition while maintaining high-resolution imaging, allowing for live cell imaging and dynamic research.
Moreover, the integration of machine learning and artificial intelligence within interferometry microscopy presents exciting possibilities. These technologies can analyze massive datasets more efficiently than traditional methods, bringing valuable insights that may have previously remained undiscovered. The combination of interferometric methods with such advanced technologies will enhance measurement precision and application variety.
Recent developments in adaptive optics are also noteworthy. This technology compensates for distortions in optical systems, improving image clarity. With adaptive optics integrated into interferometry microscopy, researchers could achieve unprecedented levels of detail in imaging microscopic structures.
Expanding Research Applications
The applications of interferometry microscopy are indeed boundless. Areas such as genetics, material science, and nanotechnology are ripe for exploration. For instance, studies related to cellular mechanics and biomolecular interactions could benefit significantly from the enhanced resolution and accuracy provided by interferometric methods.
In material science, understanding the microstructure of materials is critical. Techniques developed around interferometry microscopy can help monitor stress and strain within materials at the nanoscale, aiding in the design of stronger and more durable products.
Furthermore, interferometry microscopy’s role in biomedical research is expanding. With the ability to observe live cells in real time, this technique offers a window into complex biological processes. This capability can facilitate longitudinal studies, where changes in living systems are tracked over time, leading to a deeper comprehension of diseases or therapeutic responses.
In summary, the future of interferometry microscopy is bright. With technological advances and expanding research applications, this field will continue to contribute significantly to scientific knowledge and practical solutions across various disciplines.
Finale
In this article, we have dissected the multifaceted world of interferometry microscopy, highlighting its significance in the study of microscopic structures. The conclusion synthesizes the key insights into the principles and applications of this advanced imaging technique. Understanding these aspects clarifies why interferometry microscopy is not merely a supplementary tool; it is essential for precise measurements that surpass traditional microscopy capabilities.
Summary of Key Insights
Interferometry microscopy stands out due to its unique ability to deliver high-resolution images and quantitative data that are critical in various scientific fields. Here are the main points discussed in the article:
- Advanced Measurement: Interferometry allows for the analysis of small-scale changes in refractive index, surface topology, and other features that are often missed by conventional methods.
- Versatile Applications: This technique finds use in biological imaging, such as cell morphology and live cell studies, as well as material science, where it can assess surface topography and stress patterns.
- Challenges: While powerful, the technique does face hurdles like instrumental constraints and demanding sample preparation, which limit its accessibility.
Final Thoughts
As we reflect upon the advancements and future prospects of interferometry microscopy, it becomes clear that this technology holds immense potential for further exploration in both biological and material sciences. The continuous evolution of this field suggests that new methodologies and tools will emerge, broadening its application scope. The investment in developing better instruments and techniques will yield significant benefits, enhancing our capacity to decipher complex microscopic environments. It is vital for researchers, educators, and students to stay informed about these developments, as they potentially shape the future of scientific inquiry and innovation in microscopy.
Interferometry microscopy is not just about seeing the invisible; it is about understanding the fundamental principles that govern the microscopic world.