High Throughput Genotyping: Techniques and Applications
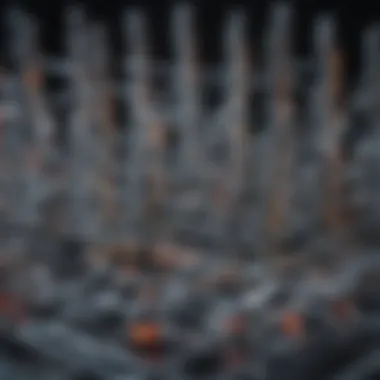
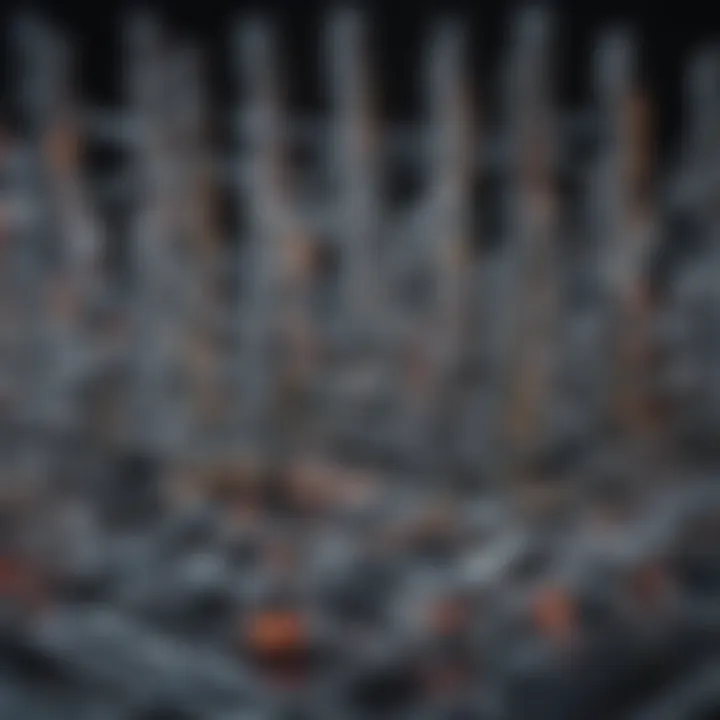
Intro
High throughput genotyping is increasingly recognized as an essential tool in genetic research and its applications across various domains. This transformative approach allows concurrent analysis of numerous genetic markers from multiple samples efficiently. Its relevance in advancing knowledge in fields like agriculture, medicine, and evolutionary biology cannot be understated. By understanding the principles and techniques of high throughput genotyping, professionals and researchers can apply this technology effectively to address pressing questions within their respective fields.
Background and Context
Overview of the research topic
High throughput genotyping represents a significant shift in how researchers approach genetic analysis. The ability to quickly assess vast amounts of genetic information enables more comprehensive studies on population genetics, marker-assisted selection, and disease association analyses. This method leverages the integration of advanced technologies, making it possible to analyze genetic markers at an unprecedented scale.
Historical significance
Historically, genotyping was often a slow and resource-intensive process. Early methods like restriction fragment length polymorphism (RFLP) required significant time and labor, limiting the scope of genetic research. The development of polymerase chain reaction (PCR) and, subsequently, next-generation sequencing (NGS) technologies laid the groundwork for high throughput genotyping. By increasing the speed and decreasing the cost of genotyping, these innovations dramatically expanded the possibilities for genetic research.
Key Findings and Discussion
Major results of the study
The application of high throughput genotyping has led to several key findings. For instance, its use in agriculture facilitates the identification of favorable traits in crops, such as drought resistance and yield improvement. Additionally, in the medical field, high throughput genotyping aids in detecting genetic predispositions to various diseases, enabling personalized medicine approaches.
Detailed analysis of findings
A comprehensive analysis of the technique reveals both strengths and limitations. On one hand, high throughput genotyping allows for:
- Large-scale sample analysis
- Enhanced statistical power in studies
- Discovery of novel genetic markers
On the other hand, certain limitations exist:
- High initial costs associated with equipment
- Data complexity requiring advanced bioinformatics tools
- Potential for errors in genotyping accuracy
"High throughput genotyping not only accelerates research but also broadens our understanding of genetic variation in natural populations."
In closing, this article investigates the principles, techniques, and applications of high throughput genotyping thoroughly. By synthesizing findings and discussing both its advantages and challenges, it aims to provide readers with a solid understanding of the significance and future directions of this crucial technology.
Prologue to High Throughput Genotyping
High throughput genotyping is a critical development in genomic research. It allows for the simultaneous analysis of numerous genetic markers. This capability is particularly valuable in studies where large sample sizes are required. As we explore this field, understanding the significance and utility of high throughput genotyping will become clear. It plays a pivotal role in various domains such as agriculture, medicine, and evolutionary biology.
Through high throughput techniques, researchers can gather vast amounts of data efficiently. This efficiency accelerates the pace of scientific discovery. Moreover, it enables the detailed exploration of genetic variations across populations. Such analyses can lead to insights in areas like disease susceptibility and trait development in crops.
Defining High Throughput Genotyping
High throughput genotyping can be defined as the process of genotyping many samples simultaneously. This means that large-scale genetic analysis can be conducted quickly and cost-effectively. Techniques involved include multiplex PCR, sequencing, and microarray technologies. These techniques support the systematic investigation of genetic ancestry, functional genomics, and comparative genomics.
By employing high throughput methods, researchers can address complex biological questions more thoroughly. The scale of analysis has expanded from single genes to entire genomes. This shift allows for a more holistic understanding of biology.
Historical Context and Evolution
The field of high throughput genotyping has evolved significantly over the past few decades. Initially, genotyping methods were labor-intensive, requiring extensive time and resources. As DNA sequencing technology advanced, the need for efficient analysis grew.
In the early 1990s, the introduction of polymerase chain reaction (PCR) revolutionized genetic research. This technique enabled the amplification of specific DNA sequences, making genotyping more accessible. The following years saw the rise of microarray technology, which allowed for the simultaneous analysis of thousands of SNPs.
Today, next-generation sequencing has become a cornerstone of high throughput genotyping. It provides unrivaled data output and resolution. This evolution represents a profound shift in genomic capabilities, empowering scientists to explore genetic information like never before.
Underlying Principles of High Throughput Genotyping
High throughput genotyping is driven by several fundamental principles that enable efficient analysis of genetic variation. Understanding these principles is crucial for appreciating how high throughput techniques contribute to genomic research. By examining genomic architecture, amplification techniques, and bioinformatics, one can grasp the comprehensive nature of this field.
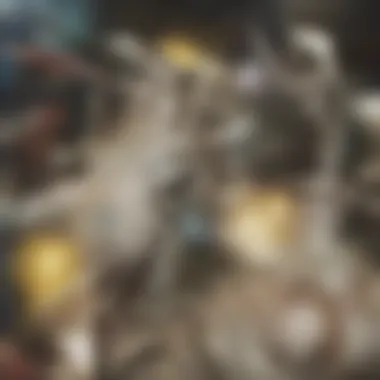
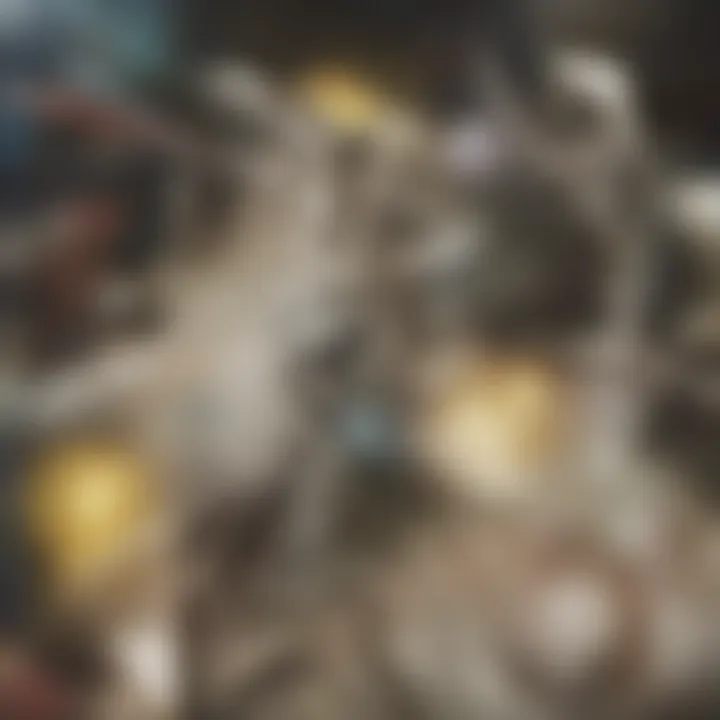
Genomic Architecture and Variation
Genomic architecture refers to the organization and structure of an organism's genome, which plays a vital role in determining its traits. Different organisms exhibit significant genomic variation, which can occur at multiple levels including single nucleotide polymorphisms (SNPs), insertions, deletions, or structural variations. This variation provides a rich landscape for exploring genetic diversity.
- Importance of Genetic Variation: Understanding genetic variation is essential for identifying the genetic basis of traits and diseases. It informs breeding programs in agriculture and enhances our knowledge of evolutionary processes.
- Architectural Features: The presence of repeated sequences, regulatory elements, and gene neighborhoods can influence phenotypic outcomes and may pose challenges in interpretation.
These explorations into genomic architecture allow researchers to pinpoint specific markers associated with desirable traits or predispositions to certain conditions.
PCR and Amplification Techniques
Polymerase Chain Reaction (PCR) is a cornerstone technique pivotal for high throughput genotyping. PCR allows for the exponential amplification of specific DNA regions, making it easier to analyze low quantities of DNA from various samples. The success of high throughput approaches often hinges on the ability to efficiently and accurately amplify genetic material.
- Types of PCR Techniques: It includes standard PCR, quantitative PCR (qPCR), and digital PCR. Each serves different needs in quantifying and analyzing genetic material. Here are some brief descriptions:
- Standard PCR: Primarily used for amplifying specific DNA segments.
- qPCR: Enables quantification of DNA, providing insights into relative abundance.
- Digital PCR: Offers precise quantification without relying on standard curves.
The selection of the appropriate PCR technique is essential in ensuring accurate and reliable results when genotyping across high numbers of samples.
Data Handling and Bioinformatics
The massive amounts of data generated from high throughput genotyping require advanced bioinformatics tools for analysis. Efficient data handling can be the difference between deriving meaningful insights and information overload.
- Data Processing: Data preprocessing involves steps such as quality control, normalization, and filtering out noise. Poorly managed data can lead to inaccurate conclusions.
- Bioinformatics Software: Utilization of software for variant calling, annotation, and visualization is critical. This allows researchers to interpret data at scale, identifying significant patterns or correlations.
- Some notable bioinformatics tools include GATK, PLINK, and SNPRelate. These applications assist in different stages of genotyping analysis.
"The proper integration of genomic methods and data analysis can revolutionize our approach to genetics, accelerating discoveries that impact diverse fields."
Future advancements in technology will likely enhance these principles further, driving unprecedented insights into the genetic basis of life.
Key Techniques in High Throughput Genotyping
High throughput genotyping encompasses several key techniques that allow for the efficient analysis of genetic variations across large sample sets. These methods provide the foundation for various applications in agriculture, medical diagnostics, and evolutionary studies. Understanding these techniques is essential as they directly influence the quality and speed of genetic insights that researchers can gain. Moreover, the choice of technique often depends on the specific research goals, budget, and available technology.
SNP Genotyping Methods
Single Nucleotide Polymorphism (SNP) genotyping is a fundamentally important method in high throughput genotyping. SNPs are the most common type of genetic variation among individuals, making their detection crucial in many genetic studies. Biological interpretation mainly relies on identifying patterns of SNPs that may indicate relationships to certain traits or diseases.
There are various techniques for SNP genotyping:
- TaqMan Assays: This method uses fluorescent probes to detect SNPs during the PCR process.
- Allele-specific PCR: Here, primers are designed to match one allele while not matching the other, allowing for differentiation.
The benefits of these methods include high specificity and quick processing. However, the technical limitations, such as the need for high-quality DNA and knowledge of the SNPs in advance, can be challenging.
Next-Generation Sequencing
Next-Generation Sequencing (NGS) has revolutionized the landscape of genomic analysis. By allowing for the sequencing of millions of fragments simultaneously, NGS enables the identification of not just SNPs but also insertions, deletions, and structural variations within genomes.
Among the benefits of NGS are:
- Comprehensive data retrieval: It provides a thorough picture of the genetic variation present.
- Cost-Effectiveness: As technology advances, costs have significantly reduced, making NGS widely accessible.
However, researchers must also navigate complexities related to data analysis and interpretation, requiring robust bioinformatics infrastructure.
Microarray Technology
Microarray technology is another cornerstone of high throughput genotyping. This technique utilizes a solid substrate onto which DNA or RNA molecules are fixed, allowing for simultaneous analysis of thousands of genetic markers. Microarrays are particularly useful for comparative gene expression studies and genotyping large populations.
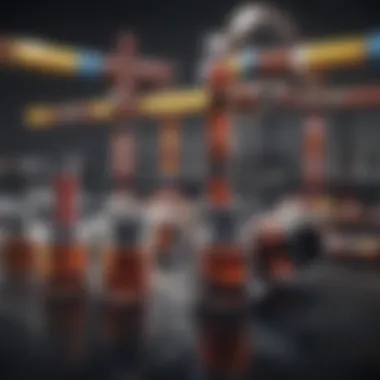
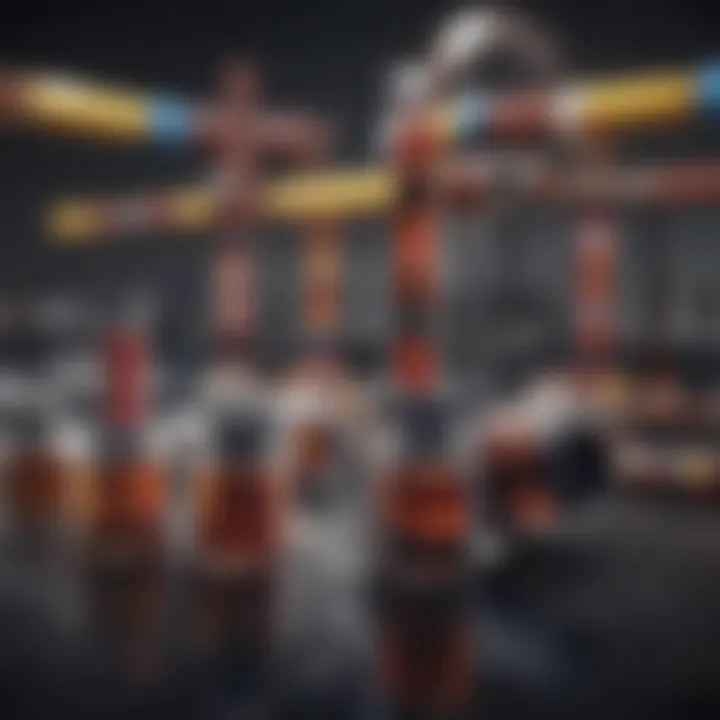
Some advantages include:
- High-throughput capability: This method can analyze thousands of samples at once, saving time.
- Flexibility: Researchers can customize microarrays for specific genetic markers or gene targets.
Despite these advantages, microarray technology can present drawbacks such as potential cross-hybridization and the need for sophisticated interpretation of the data produced.
Digital PCR Techniques
Digital PCR represents a groundbreaking advancement in quantitative genotyping. This technique partitions a sample into numerous small reactions, providing a more precise quantification of DNA sequences than traditional PCR methods.
Key points of interest include:
- Sensitivity: Digital PCR can detect rare alleles with high precision due to its partitioning approach.
- Robust data: It minimizes variations caused by PCR amplification differences.
Nonetheless, the complexity of digital PCR technology and its operational costs can limit accessibility for some laboratories.
In summary, the key techniques in high throughput genotyping serve diverse roles in genomic studies. As technology advances, these methods will continue to evolve, enhancing their contribution to genetic research and applications across multiple domains.
Applications of High Throughput Genotyping
High throughput genotyping has transformed the approach to genomic research and practical applications across multiple fields. This section explores various sectors where high throughput genotyping has proven indispensable. Understanding these applications can illuminate its significance and potential benefits, as well as encourage innovations that may arise in the future.
Agricultural Genomics
High throughput genotyping plays a crucial role in agricultural genomics by facilitating the breeding of crops and livestock with desirable traits. The ability to genotype large numbers of individuals efficiently allows for more precise selection based on genetic characteristics. In this context, marker-assisted selection becomes a powerful tool, where specific genetic markers linked to traits such as disease resistance or yield can be identified swiftly.
Moreover, this technology can aid in the study of genetic diversity within species. By assessing genetic variation, researchers can make informed decisions related to conservation and breeding strategies. The advent of genomics in agriculture enables farmers and scientists to develop varieties that are resilient to climate change and pests, ultimately leading to improved food security.
Clinical and Medical Diagnostics
High throughput genotyping significantly advances clinical and medical diagnostics. It enables healthcare practitioners to analyze a multitude of genetic markers associated with various diseases efficiently. This capability is particularly vital for identifying predispositions to certain conditions, guiding personalized medicine approaches, and tailoring treatment plans based on an individual’s genetic makeup.
The integration of high throughput technologies in diagnostics can lead to the early detection of genetic disorders, which is often crucial for effective intervention. Furthermore, tracking disease outbreaks and understanding population genetics has become more streamlined, allowing for better public health responses. The implications for patient care and health outcomes are vast, demonstrating the profound impact of this technology in the medical field.
Conservation Biology and Biodiversity Studies
In the realm of conservation biology, high throughput genotyping is essential for maintaining biodiversity. This approach assists in monitoring genetic diversity among endangered species and assessing the effects of habitat fragmentation. Conservationists can identify genetically distinct populations, which is critical for effective management strategies. Protecting diverse genotypes helps enhance resilience to environmental changes, making populations more sustainable in the long term.
Additionally, high throughput genotyping can support efforts to track the effectiveness of conservation programs over time. By establishing baseline genetic data, scientists can evaluate whether conservation strategies are having a positive impact. This method also includes investigating the genetic basis of traits vital for survival in natural habitats, thus informing future conservation efforts.
Evolutionary Studies
In evolutionary biology, high throughput genotyping facilitates the exploration of evolutionary processes. By analyzing genetic variation across populations, researchers can infer phylogenies and understand relationships between species. This technology enables questions regarding adaptation and speciation to be addressed more thoroughly.
The fine-scale genetic data obtained through high throughput genotyping allows for the examination of evolutionary change on a micro-evolutionary scale. Studies can track how populations evolve in response to environmental pressures. This understanding is critical for developing theories about natural selection and adaptation, thereby contributing to a more nuanced appreciation of biodiversity.
High throughput genotyping is not just a tool; it is a gateway to unraveling complexities in genomics and related fields, driving forward research and practical applications.
Challenges in High Throughput Genotyping
High throughput genotyping has quickly established itself as a critical component of genomic research. However, the journey towards its widespread adoption is not without challenges. Understanding these challenges provides valuable insights into the field's constraints and future potential. Factors including technical limitations, ethical considerations, and data interpretation issues must be addressed to maximize the impact of high throughput genotyping.
Technical Limitations
One of the prominent hurdles in high throughput genotyping is technical limitations. Despite advances, available technologies still encounter issues that hinder optimal performance.
- Sensitivity and Specificity: High throughput systems, such as next-generation sequencing, require high sensitivity and specificity. In some cases, inaccuracies may arise, leading to false positives or negatives. This can skew study results.
- Sample Quality and Preparation: The quality of DNA samples can greatly influence the outcomes. Poor-quality samples can compromise the whole process, resulting in genotyping errors.
- Scalability Issues: As projects expand, scaling up high throughput genotyping efforts can lead to bottlenecks in sample processing and analysis. This is particularly pertinent in large-scale genome-wide association studies (GWAS).
- Cost Considerations: Although prices have decreased, the initial setup costs can remain significant. Organizations need to invest in equipment and trained personnel to ensure proper application of high throughput genotyping techniques.
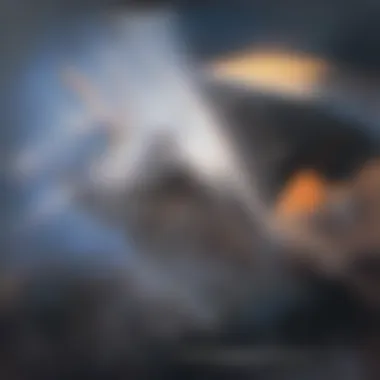
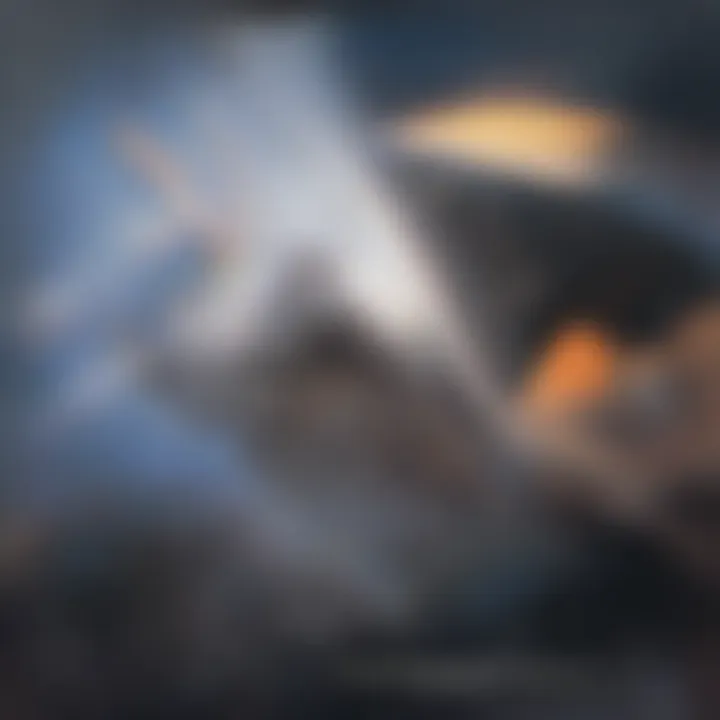
Ethical Considerations
As with any powerful technology, high throughput genotyping raises ethical considerations that cannot be overlooked. These concerns revolve around privacy, consent, and the potential misuse of genetic information.
- Privacy and Data Security: The storage and handling of genetic data raise significant privacy concerns. There is a risk that sensitive information could be exposed or misused, leading to discrimination.
- Informed Consent: Participants in genomic studies must fully understand the implications of sharing their genetic information. A lack of transparency can undermine the ethical foundation of research.
- Equitable Access: Not all groups have equal access to genomic technologies. This can lead to disparities in health care and research benefits, promoting inequality in healthcare outcomes.
Data Interpretation and Analysis Issues
Handling the vast amounts of data generated presents data interpretation and analysis issues. The complexity of genetic data necessitates robust analysis methods to draw accurate conclusions.
- Bioinformatics Challenges: The integration of bioinformatic tools into high throughput genotyping is essential. However, bioinformatics is still developing, and many researchers struggle to analyze large datasets effectively.
- Incorrect Assumptions: Researchers may inadvertently make assumptions when interpreting results. For instance, correlating genotypic variation directly to phenotypic outcomes can be misleading.
- Standardization: Lack of standardization in data processing can result in disparate results across studies, complicating meta-analyses and limiting the reproducibility of findings.
"The ability to interpret and trust the findings of high throughput genotyping is critical for the scientific community and the impact on health and agriculture."
Navigating the challenges outlined is vital for researchers, educators, and professionals engaged in high throughput genotyping. Each facet—technical limitations, ethical considerations, and data interpretation—plays a significant role in the nuanced understanding of this field. Addressing these challenges can help unlock the full potential of high throughput genotyping, pushing the boundaries of genetic research and its applications.
Future Directions in High Throughput Genotyping
High throughput genotyping is evolving rapidly, and its future directions hold great promise for advancing genomic research. This section focuses on innovations, the integration of artificial intelligence, and potential impacts on personalized medicine. Understanding these aspects is essential as they reflect the trajectory of genomic technologies in various applications.
Innovations in Genotyping Approaches
The drive for innovation defines the future of high throughput genotyping. Emerging technologies are enhancing the efficiency and accuracy of genomic analyses. Techniques like CRISPR-based systems for precise genotyping are gaining traction. These technologies allow researchers to identify genetic variations with unprecedented specificity.
Moreover, innovations in multiplexing capabilities enable the analysis of multiple variants in a single assay. This approach saves time and reduces costs, making large-scale studies more feasible.
New platforms such as Ion Torrent and PACBIO are redefining the landscape by offering faster sequencing speeds and reduced sample requirements. These advancements facilitate a better understanding of complex traits in organisms. Improved sensitivity in detecting low-frequency variants is also contributing to our understanding of diseases and genetic diversity.
Integrating Artificial Intelligence in Genomic Studies
Artificial intelligence is becoming integral to genomic studies. Machine learning algorithms can analyze vast datasets swiftly, identifying patterns that may remain hidden from traditional methods. This integration provides several benefits:
- Enhanced Data Analysis: AI can automate the identification of genetic variants, streamline workflows, and reduce the time required for data interpretation.
- Predictive Modeling: By utilizing historical data, AI can assist in predicting outcomes based on genetic information, a vital asset in both clinical and agricultural settings.
- Personalized Approaches: AI-driven analyses facilitate tailored treatment plans in medicine. This is particularly beneficial for understanding complex genetic interactions and patient responses.
As AI tools evolve, they will amplify the capabilities of high throughput genotyping, making it even more powerful in uncovering insights into genetic variation and related phenotypic expressions.
Potential Impacts on Personalized Medicine
The implications of high throughput genotyping on personalized medicine are profound. By deciphering an individual’s genetic makeup, healthcare can shift from a one-size-fits-all approach to more targeted interventions. Here are some ways this could manifest:
- Tailored Therapies: Understanding specific genetic markers can lead to medications tailored to individual profiles, improving efficacy and reducing adverse effects.
- Preventive Strategies: Identification of genetic predispositions allows for early interventions and lifestyle modifications to prevent diseases from developing.
- Integration of Genotype Data: As more genomic information becomes available, integrating it with clinical data will create comprehensive models that improve treatment outcomes.
"The future of medicine is personal; understanding our genome is a crucial step in that journey."
Finale
High throughput genotyping represents a cornerstone in the field of genomics, reflecting its significance in various areas, including agriculture, medicine, and evolutionary biology. This approach allows us to analyze genetic variations across multiple samples simultaneously. Understanding the mechanisms and techniques of high throughput genotyping is crucial for researchers and practitioners alike. It enables them to harness large datasets, leading to more informed decision-making and innovative discoveries.
Recap of High Throughput Genotyping Importance
The importance of high throughput genotyping cannot be overstated. It provides:
- Efficiency: By processing numerous samples at once, it saves time and labor costs compared to older methods.
- Enhanced Resolution: High throughput systems can detect subtle genomic variations that might be overlooked in smaller, single-sample studies.
- Broader Application: From crop improvement in agriculture to understanding complex diseases in healthcare, the applications are vast and impactful.
This methodology is essential for researchers seeking to make sense of large quantities of genetic data. As genomic technologies advance, the integration of high throughput approaches becomes not just advantageous but necessary for staying competitive in scientific research.
Final Thoughts on Future Prospects
The future of high throughput genotyping holds considerable promise. Innovations in genotyping approaches, especially those blending artificial intelligence, can optimize data analysis processes. Such integrations may:
- Lead to increased accuracy in variant calling and phenotype association.
- Facilitate personalized medicine, improving patient care tailored to individual genetic profiles.
- Extend the reach into underexplored areas of biodiversity and conservation, providing essential insights into species interactions and ecosystem health.
In summary, as technology evolves, so too will the capabilities and applications of high throughput genotyping. Continuous exploration will not only enhance our understanding but also open new frontiers in genetics and beyond. Embracing these advancements will pave the way for future breakthroughs, fostering an era where genomic data drives transformative changes in numerous disciplines.