Exploring Scanning Electrochemical Microscopy: Insights and Applications
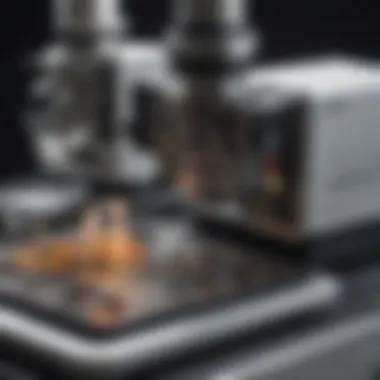
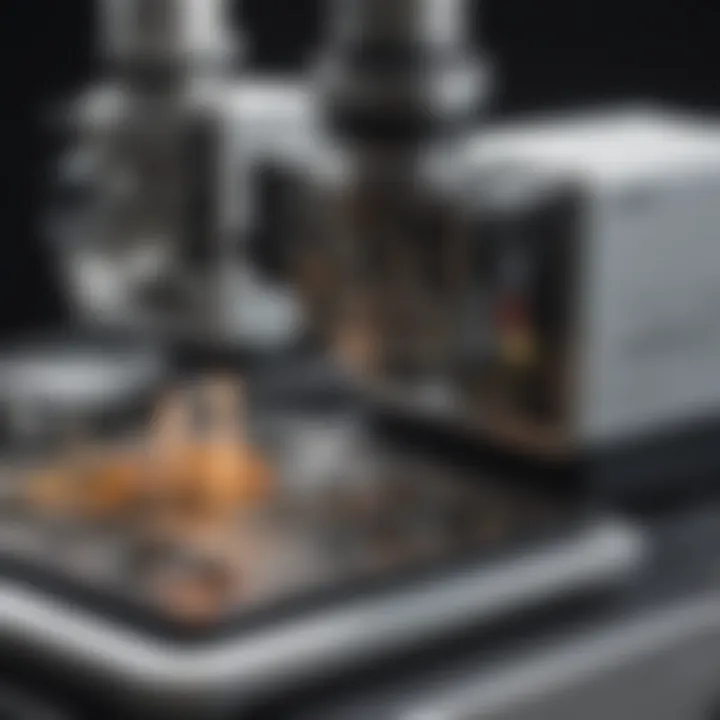
Intro
Scanning electrochemical microscopy (SECM) has emerged as a pivotal technique in the realm of electrochemical analysis. This powerful tool allows the in-depth study of electrochemical processes at the nanoscale. Understanding its principles is essential for both researchers and practitioners interested in technological advancements. In this article, we will explore the foundational concepts of SECM, examine its diverse applications, and consider its future directions.
Background and Context
Overview of the Research Topic
SECM provides a unique capability to map electrochemical activity in real time. It involves scanning a microelectrode across a sample surface while measuring current responses. This enables scientists to visualize chemical processes with unprecedented detail. The versatility of SECM extends beyond mere visualization; it enables manipulation within electrochemical environments, giving it utility in multiple scientific domains.
Historical Significance
First introduced in the late 1980s, SECM has gained traction as a significant technique in electrochemistry. Early studies focused on its fundamental methodology. Over time, applications expanded into materials science, biology, and even environmental studies. Its evolution reflects a growing desire for precise and localized electrochemical measurements. As a result, SECM was recognized for its potential to enhance our understanding of complex chemical systems.
Key Findings and Discussion
Major Results of the Study
SECM's distinctive advantages lie in its high spatial resolution and sensitivity. It has been successfully applied to study ion transfer dynamics, nanoscale material properties, and biological interactions. Recent studies illustrate its capability to identify electroactive species at the single-cell level, marking a significant step in bioelectrochemistry.
Detailed Analysis of Findings
In analyzing SECM results, several key factors must be taken into consideration:
- Electrode Material: The choice of microelectrode can drastically affect sensitivity and resolution.
- Scanning Parameters: Conditions under which the scanning occurs impact the overall interpretation of data.
- Environmental Factors: Variables such as temperature and ionic strength also play vital roles in measurement accuracy.
"SECM stands at the intersection of chemistry, biology, and materials science, providing a platform for multidisciplinary research."
As researchers continue to develop this technique, its applications will likely broaden, furthering our understanding of both fundamental chemistry and practical applications.
Preface to Scanning Electrochemical Microscopy
Scanning electrochemical microscopy (SECM) is a pivotal technique in modern analytical chemistry and materials science. Its ability to probe electrochemical processes at the nanoscale allows it to unveil intricate details within various systems. Understanding SECM is crucial for anyone involved in material characterization, biological research, or environmental monitoring, as it combines electrochemistry with high-resolution imaging. By offering real-time insights into surface phenomena, SECM enhances our capability to analyze complex materials and reactions.
Historical Context
The inception of scanning electrochemical microscopy can be traced back to the late 1980s and early 1990s. Early developers aimed to visualize electrochemical reactions, pushing the boundaries of spatial resolution. The foundational ideas stemmed from traditional electrochemical methods, which provided limited spatial information. As technology evolved, researchers began integrating scanning probe techniques, allowing for nanoscale resolution.
SECM established itself through pivotal studies that demonstrated its versatility. Initially, it was used primarily in electrochemistry, but its applications expanded across materials science and biological systems. As awareness grew around the importance of interfacial phenomena, SECM became a primary tool for investigating these dynamics in various states of matter.
Defining Scanning Electrochemical Microscopy
Scanning electrochemical microscopy is defined as a scanning probe technique that employs a microelectrode to localize electrochemical measurements at the sample surface. The working principle involves positioning a small electrode in close proximity to the surface of interest. This allows for the measurement of the local electrochemical activity, giving insights into redox reactions and Ion transfer processes.
The system setup typically consists of a potentiostat, a positional feedback loop, and a microelectrode. By applying a voltage at the electrochemical call, practitioners can determine the current responses that occur when substances interact with the electrode. The resulting data can be processed to render spatial maps, illustrating the electrochemical characteristics of the sample. This functionality is valuable in various contexts including studying corrosion, developing catalysts, and examining cellular environments.
"SECM stands as a bridge, connecting electrochemical analysis to imaging at an unprecedented resolution, revealing the unseen dynamics within materials and biological systems."
Fundamentals of SECM
Understanding the fundamentals of Scanning Electrochemical Microscopy (SECM) is crucial for grasping the versatility and applicability of this technique in various scientific realms. SECM offers a nanoscale perspective on electrochemical processes, merging electrochemistry, microscopy, and material science into a singular investigative tool. This section elucidates the basic principles of electrochemistry that underpin SECM and details the key components integral to the SECM setup, which together form the backbone of effective usage in research and practical applications.
Basic Principles of Electrochemistry
Electrochemistry forms the core of SECM operations. At its essence, it studies chemical processes that involve the movement of electrons and ions. This involves oxidation and reduction reactions, where a substance either loses electrons during oxidation or gains them during reduction. A basic principle in electrochemistry is the Nernst equation, which relates the concentration of reactants and products to the potential of the electrochemical cell. This foundational insight allows researchers to manipulate conditions, optimize reactions, and interpret data with precision.
Electrochemical interfaces play a significant role in SECM, as they determine how an applied potential can influence the detection of analytes. By leveraging these properties, researchers can quantify localized reactions and monitor their dynamics in real-time. The relevance of electrochemical principles thus permeates through the analytical capabilities of SECM, enabling detailed investigation of complex systems such as catalytic surfaces and biological interactions.
Key Components of SECM Setup
The effectiveness of SECM hinges on its specific components, which come together to create a comprehensive analytical framework. Each component plays a distinct role in ensuring accurate measurements and reliability in data interpretation.
Electrode Types
Electrode types are fundamental to the success of any SECM experiment. The choice of electrode directly affects the sensitivity and selectivity of measurements. Common electrode materials include glassy carbon, gold, and platinum, each offering unique properties. For instance, glassy carbon electrodes are widely appreciated for their stability and low capacitance, while platinum electrodes can provide enhanced electrocatalytic properties. The beneficial characteristic of these electrodes is their ability to generate a measurable current in response to electrochemical reactions.
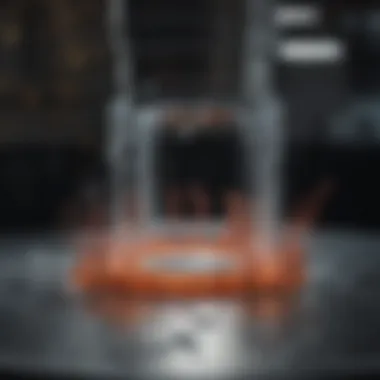
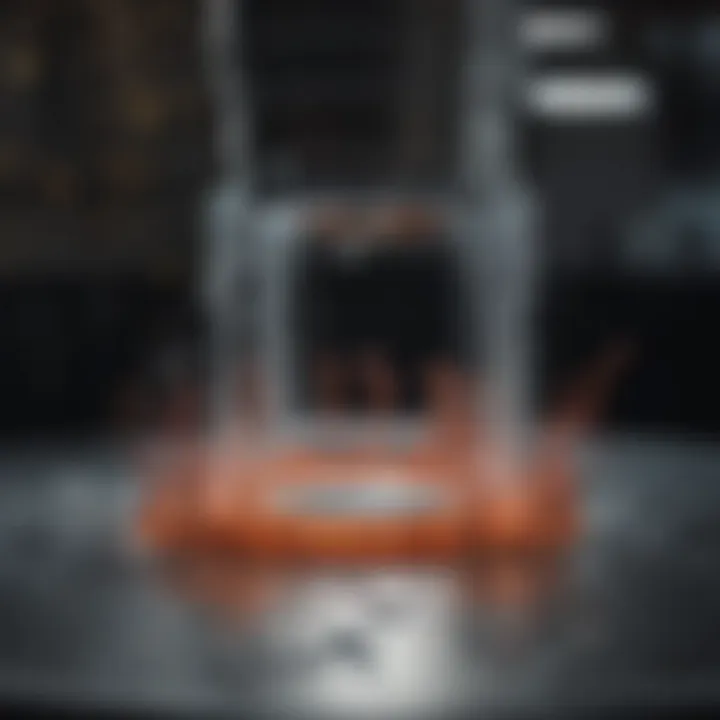
A notable feature of electrode design is the ability to modify their surfaces to enhance performance. This aspect allows for tailored applications that can address specific analytical challenges. However, there can be limitations, such as durability and the potential for fouling, which researchers must consider when designing their experiments.
Scanning Mechanism
The scanning mechanism in SECM is pivotal for achieving high-resolution imaging. This aspect involves the controlled movement of the probe, typically an ultramicroelectrode, across the sample surface. The key characteristic of this mechanism is its ability to maintain a precise distance from the sample, which is vital for accurate measurements.
One unique feature is its capability to analyze samples in both conductive and non-conductive states, broadening the range of applications. However, challenges can arise from environmental factors, such as vibrations or thermal drifts, which may affect measurement accuracy. Addressing these limitations is part of ongoing research in refining SECM methodologies.
Control System
The control system is the integral component that orchestrates the entire SECM process. This system manages the probe’s movement and optimizes the electrochemical conditions required for analysis. The key characteristic of this system is its ability to execute complex algorithms that can adapt to real-time changes in the sample environment.
A unique benefit is the incorporation of feedback loops that enhance measurement reliability. The system adjusts probe positioning based on current readings, enabling high-resolution topographic mapping of surfaces. Despite this, there are recognized limitations, such as the need for calibration and the complexity involved in system integration, which can affect overall ease of use.
Methodology in SECM Studies
In scanning electrochemical microscopy (SECM), the methodology employed can significantly dictate the outcomes of experiments. Proper methodology forms the backbone of the entire analytical process, ensuring results are reliable and valid across different contexts. The importance of methodology in SECM studies cannot be overstated, as it lays the groundwork for precision in understanding various electrochemical phenomena.
The methodology includes key components such as preparation of samples and calibration procedures. Each of these elements plays a critical role in the experimental setup and influences the quality of the data collected. By embracing a rigorous methodological approach, researchers can avoid common pitfalls and enhance the accuracy of their findings.
Preparation of Samples
Preparation of samples in SECM is fundamental for achieving precise measurements. The process involves several steps, tailored to the specific needs of the experiment. First, samples must be cleaned thoroughly to remove any contaminants that could interfere with electrochemical measurements. Common cleaning methods include chemical, ultrasonic, and electrochemical cleaning. Each technique offers advantages in terms of efficiency and effectiveness, depending on the sample type and desired purity.
Next, the choice of the substrate is vital. The material used can affect the electron transfer processes and the overall responses observed. Researchers often choose conductive substrates to facilitate better interfacing with the SECM tip. Moreover, ensuring the sample surface is free of defects will lead to more reproducible results.
Once samples are prepared, they should be handled with care. Environmental factors, such as exposure to air or moisture, can alter their properties. It is crucial to store samples appropriately prior to measurement, preferably in conditions that mitigate degradation.
Calibration Procedures
Calibration procedures are essential for ensuring the accuracy and reliability of SECM measurements. These procedures allow for the establishment of a known relationship between the measured data and the actual electrochemical activity of the sample. One common method of calibration involves using standard redox-active solutions. By conducting measurements with these standards, researchers can obtain a baseline response that can be compared with subsequent experimental data.
Moreover, the calibration can also encompass the response of the scanning electrode itself. Proper adjustment of scanning parameters, such as tip distance and voltage, is necessary. Regular calibration of the system helps to identify any drifts or changes in the setup that might affect measurements over time.
In addition, software settings for data acquisition play an important role. Ensuring that the software is properly configured to collect data under the right conditions is critical. Misconfiguration can lead to erroneous data interpretation, resulting in misleading conclusions.
The extent to which methodology, including sample preparation and calibration procedures, is executed directly influences the integrity of findings in SECM studies.
Overall, a meticulous approach to methodology in SECM studies not only optimizes measurements but also enhances the credibility of the results, propelling the scientific understanding of electrochemical processes. By implementing effective preparation and calibration strategies, researchers can unlock the full potential of SECM in various applications.
Types of Scanning Modes
Scanning electrochemical microscopy (SECM) employs various scanning modes, each designed to enhance the capabilities of the technique in specific research scenarios. These modes are essential to understanding the spatial distribution of electrochemical reactions and provide critical insights into material properties and biological processes. Selecting the appropriate scanning mode can significantly influence the quality of data obtained, impacting both analysis and applications.
Feedback Mode
Feedback mode is one of the most widely used scanning methods in SECM. In this mode, the probe typically measures the current generated by a redox reaction occurring near the surface of the sample. The feedback from the probe is directly proportional to changes in the local concentration of redox-active species. This allows for the mapping of electrochemical activity on a surface at high spatial resolution.
The importance of feedback mode lies in its ability to provide real-time insights into reaction dynamics. Researchers can visualize areas of varying electrochemical activity, which is vital in fields such as corrosion studies, surface modification and catalysis. The precision of feedback mode enables the detection of subtle changes in local concentration, making it significant for the examination of heterogeneous surfaces.
"Feedback mode exhibits the unique ability to reveal the complex interactions between electrochemical processes and the surface topography of materials."
However, some challenges accompany the feedback mode. It can be susceptible to noise from the environment, which may compromise data quality. Moreover, the selection of the appropriate probe and the distance between the probe and the sample require careful optimization. Improper settings can lead to inaccurate localization of activity.
Distant Measurement Mode
Distant measurement mode, or non-feedback mode, operates under different principles than feedback mode. Here, the probe measures the current without significantly influencing the local concentration of reactants. This mode is particularly helpful when mapping the diffusion fields of species and understanding broader electrochemical behaviors over larger distances.
The key advantage of distant measurement mode is its ability to examine regions where local interactions are minimal. Researchers can gather information about the bulk characteristics of materials rather than just surface electrochemical properties. This is significant for applications in material science, where understanding the behavior of materials at the macro-level can lead to innovations in design and application.
In summary, both scanning modes play crucial roles in SECM. Feedback mode excels in providing localized data on electrochemical activity, while distant measurement mode facilitates a broader perspective on material behaviors. Choosing the correct mode depends largely on the specific research objective and the critical questions that need answering.
Applications of SECM
The applications of Scanning Electrochemical Microscopy (SECM) span various fields, making it an invaluable tool for researchers and professionals. SECM allows for real-time assessment of electrochemical reactions and surface features at the nanoscale. Its versatility is its principal strength. The maneuverability of the technique accommodates numerous scientific inquiries, from materials science to biological studies, thus enhancing its position in contemporary research.
Materials Science Applications
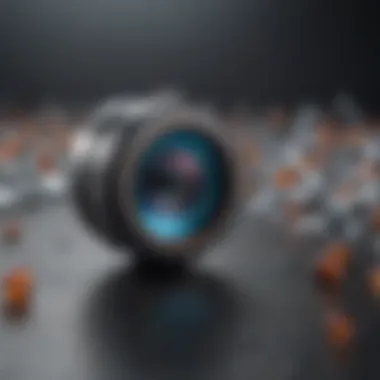
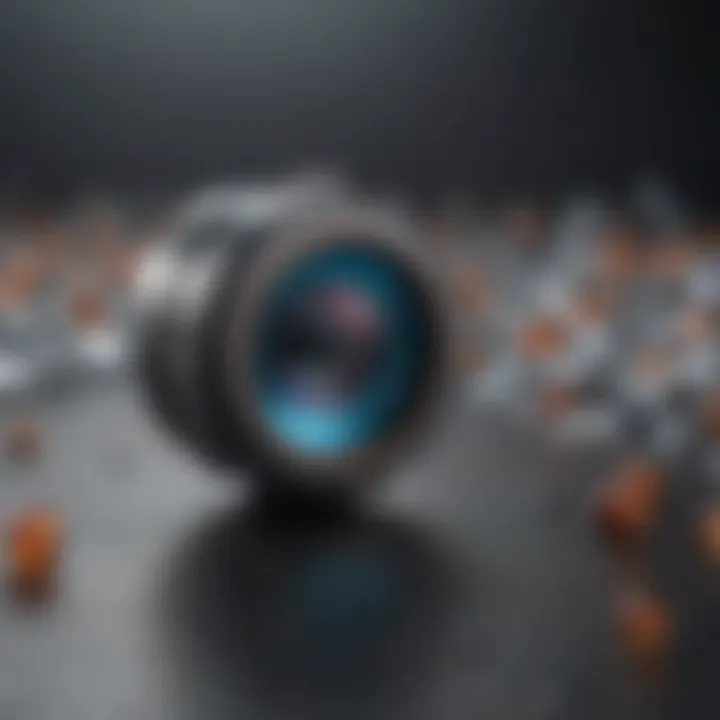
In materials science, SECM plays a crucial role in characterizing surface properties and reactions of materials. It provides insights into various phenomena such as corrosion, film growth, and surface modifications. For example:
- SECM can evaluate electrode materials, helping identify ideal compositions for energy storage systems.
- It also allows for the analysis of solid electrolytes, which are essential for next-generation batteries.
- Mapping of conductive pathways in materials can facilitate the development of more efficient photovoltaic devices.
By using SECM, researchers can examine the electrochemical activity of materials in real-time, giving them the ability to understand the processes that impact material performance. This knowledge can guide innovations in the design and application of new materials.
Biological Systems and Cellular Analysis
The application of SECM in biology is increasingly significant. It enables examination of cellular processes and electrochemical events at the micron and nanoscales. Some notable uses include:
- Cellular Metabolism: SECM can monitor metabolic processes in cells by detecting the concentration of specific analytes.
- Drug Delivery: The technique can investigate the effects of drugs on cellular electrochemical properties, providing insights into drug action and distribution.
- Tissue Engineering: SECM aids in evaluating the electrochemical behavior of biomaterials used in tissue scaffolds, promoting better integration with living tissues.
Furthermore, SECM offers the capability to investigate localized electrochemical activity, thus contributing significantly to the understanding of cellular functions. This can lead to advancements in treatment strategies and therapeutic applications.
Environmental Studies
Environmental science can greatly benefit from the applications of SECM. The technique aids in the detection and characterization of pollutants at low concentrations. Key aspects include:
- Monitoring Heavy Metals: SECM enables detection of heavy metals in soil and water samples, allowing for timely assessment of contamination levels.
- Studying Water Quality: The technique can track electrochemical indicators of water quality, such as dissolved oxygen levels and pH variations.
- Microbial Interactions: SECM helps investigate the electrochemical interactions between microbial communities and their environments, which is essential for bioremediation strategies.
By harnessing SECM's capabilities in environmental studies, researchers can develop effective monitoring systems to protect ecosystems and promote sustainability.
SECM's diverse applications highlight its potential as a critical tool in advancing scientific knowledge and technological innovations.
Interpreting SECM Data
Interpreting data from Scanning Electrochemical Microscopy (SECM) is a critical component of utilizing this powerful technique. Understanding the data enables researchers to draw meaningful conclusions about electrochemical processes at the nanoscale. This section outlines the essential elements involved in data interpretation, the associated benefits, and the considerations researchers must keep in mind.
Data Analysis Techniques
A range of data analysis techniques are employed in interpreting SECM results. These methods help to clarify the information obtained during experiments and allow researchers to visualize complex electrochemical events. Below are some prominent techniques used:
- Current Mapping: This technique involves visualizing current responses across the scanned area, revealing the distribution of reactants and products.
- Potential Mapping: By plotting the local potential variations, researchers can identify areas of interest regarding redox activity.
- Signal Processing: Tools such as Fourier transform and wavelet analysis enhance the signal to noise ratio, allowing for more precise interpretations of the electrochemical signals.
- Modeling Approaches: Computational methods simulate the expected SECM data under various conditions, facilitating comparison with experimental results. This modeling serves both to validate findings and generate hypotheses for future research.
The combination of these techniques can yield invaluable insights into the characteristics and behaviors of materials at the micro and nanoscale.
Challenges in Data Interpretation
While the techniques mentioned previously provide a solid framework for analysis, several challenges in data interpretation persist. These challenges can complicate the understanding of SECM data and require careful consideration. Some of the notable obstacles include:
- Noise and Artifacts: External factors can introduce noise into the measurements. This can obscure important signals and lead to misinterpretations if not adequately addressed. Advanced filtering techniques may be necessary to minimize these effects.
- Electrode Drift: Variations in the position of the scanning electrode during measurements can result in inaccuracies. Maintaining a stable setup is crucial to achieve consistent results.
- Limited Resolution: There is often a trade-off between the resolution of the scans and the responsiveness of the system. Finding the optimal parameters for specific experiments can be a significant challenge.
- Reaction Kinetics: Understanding the kinetics of chemical reactions studied with SECM requires a solid grasp of transport phenomena, which may complicate data interpretation.
Addressing these challenges is essential for researchers who wish to leverage SECM effectively. A deep understanding of both the analysis techniques and the potential obstacles can empower scientists to extract valid conclusions from their data.
"The quality of data interpretation can greatly influence the outcomes of SECM studies, impacting the advancement of knowledge in electrochemical processes."
In summary, navigating the complexities of interpreting SECM data requires expertise and careful attention. By mastering data analysis techniques and acknowledging potential challenges, researchers can significantly enhance the value of their findings.
Comparative Techniques to SECM
In the realm of electrochemical analysis, Scanning Electrochemical Microscopy (SECM) holds a unique position. However, understanding its capabilities often necessitates a comparative analysis with other techniques. Such an examination provides valuable insights into the strengths and shortcomings of SECM, ultimately informing researchers about which method suits their needs best. This section delves into two notable comparative techniques: Differential Interference Contrast Microscopy and Atomic Force Microscopy. Each of these methods brings distinct attributes to the table, contributing to their significance in specific research contexts.
Differential Interference Contrast Microscopy
Differential Interference Contrast Microscopy (DIC) is a technique known for its ability to enhance contrast in unstained biological samples, making it useful in observing live cells. This method creates a contrast based not on absorption but on differences in optical path length, which is particularly beneficial when studying intricate cellular structures.
- Advantages of DIC:
- It offers high resolution, allowing researchers to observe fine structural details.
- The technique does not require fluorescent labeling, preserving the sample's original state.
- It's effective in real-time imaging, enabling monitoring of dynamic biological processes.
Nevertheless, DIC also has its limitations. It can be sensitive to specimen thickness and the refractive index. Unlike SECM which can provide quantitative electrochemical data, DIC primarily delivers morphological information. This means that for comprehensive analysis, combining methods might be necessary.
Atomic Force Microscopy
Atomic Force Microscopy (AFM) is another pivotal technique, providing high-resolution imaging at the nanoscale. AFM utilizes a cantilever with a sharp tip to scan the sample's surface, measuring forces that occur between the tip and the sample, thus generating topographical maps.
- Benefits of AFM:
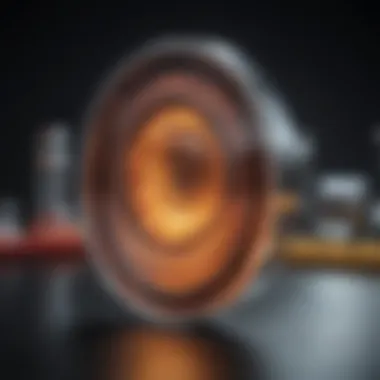
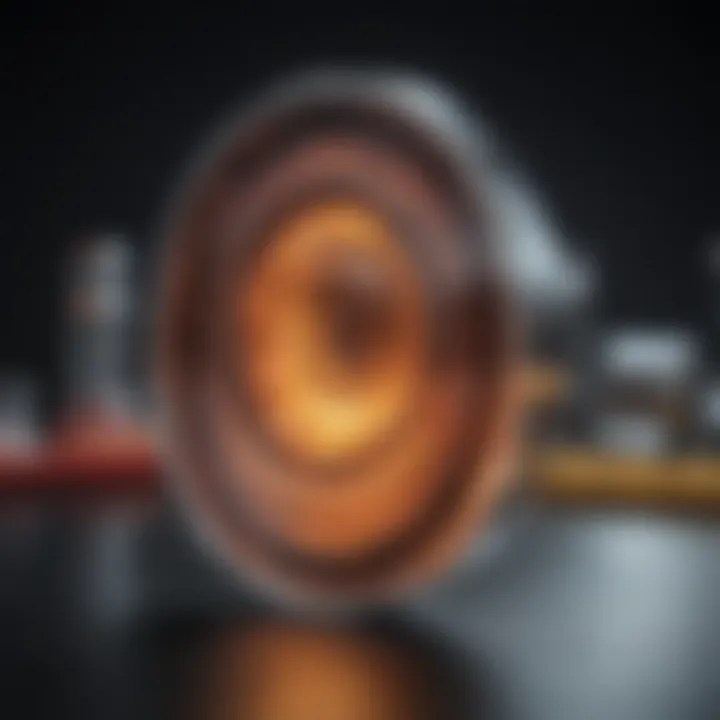
- It offers 3D imaging with nanometer resolution, ideal for topographic studies.
- It can measure various forces, such as van der Waals and electrostatic forces, providing comprehensive physical characterization.
- It works on a wide array of materials, including both conductive and non-conductive surfaces.
However, AFM's complexity and need for precise calibration can pose challenges, especially for beginners. Furthermore, unlike SECM, it does not provide direct insights into electrochemical reactions. This distinction highlights the importance of complementary techniques.
"The integration of different imaging techniques enriches scientific understanding, allowing researchers to exploit the strength of each method."
In summary, while both Differential Interference Contrast Microscopy and Atomic Force Microscopy possess valuable capabilities, their application often depends on the specific goals of the investigation. Researchers should weigh the advantages and limitations of these techniques against the distinct merits of SECM to determine the most appropriate methodology for their studies.
Advantages and Limitations of SECM
Understanding the advantages and limitations of Scanning Electrochemical Microscopy (SECM) is vital for those engaged in its application. This section elucidates specific elements that define the technique's strengths and recognized constraints within the scientific community.
Strengths of the Technique
SECM presents several advantages, making it a preferred method for many researchers. One significant strength lies in its spatial resolution. With the ability to achieve nanoscale measurements, SECM helps in visualizing electrochemical processes with great precision. This capability is particularly useful in fields like materials science and biological studies, where understanding surface interactions at the nanoscale is crucial.
Another advantage is the versatility of SECM. It can sweep across a variety of environments, enabling the analysis of solid, liquid, and gaseous systems. The method also allows for different scanning modes, which enhances its utility in diverse study contexts. Additionally, SECM can be combined with other techniques, providing comprehensive data that enrich experimental outcomes.
The ability to dynamically monitor reactions in real-time represents a third strength. Through feedback mechanisms, SECM can provide immediate insights into electrochemical changes. This real-time monitoring capacity is influential in material development and process optimization.
"SECM is not just a tool; it is an essential instrument for understanding complex chemical phenomena at an unprecedented scale."
Recognized Limitations
While SECM offers numerous advantages, it is not without limitations. One major concern is its sensitivity to environmental conditions. Factors such as temperature fluctuations and humidity can influence measurements, often leading to variability in results. As a result, strict control of experimental conditions becomes necessary to ensure accuracy.
Another limitation is the requirement for skilled operation. Effective use of SECM demands substantial expertise, particularly in parts like calibration and data interpretation. For novice users, the technical demand might represent a steep learning curve, which could hinder experimental progress.
Additionally, SECM can be constrained by poor reproducibility under certain conditions. Inconsistent preparation of samples or varying electrode properties may yield unreliable results. This unpredictability poses significant barriers in scenarios where consistent outputs are essential.
Future Directions in SECM Research
The field of Scanning Electrochemical Microscopy (SECM) is rapidly evolving as advancements in technology and methodology open new avenues for research and applications. This section outlines the significant future directions of SECM research, highlighting the importance of integrating SECM with other analytical techniques, advancements in instrumentation, and potential novel applications. These developments not only enhance the capabilities of SECM but also contribute to a broader understanding of electrochemical processes across various domains.
Integration with Other Analytical Techniques
Integrating SECM with complementary analytical methods can significantly expand its utility and the depth of insights obtained. One primary benefit is the ability to correlate electrochemical data with structural, optical, or spectral information. Techniques such as Atomic Force Microscopy (AFM) and Raman Spectroscopy can be combined with SECM to provide a multi-faceted view of the sample under examination. This holistic approach enables researchers to study materials and biological systems in detail, enhancing the reliability of the conclusions drawn from the combined data.
Moreover, the combination of SECM with chemical imaging techniques could lead to real-time monitoring of processes, enabling a more dynamic understanding of electrochemical reactions. With increased sensitivity and resolution, integrating diverse methodologies will allow for the investigation of heterogeneous systems and complex biological interactions more efficiently. This trend is critical for advancing research in nanoelectronics, biomedicine, and environmental science.
Advancements in Instrumentation
Continuous improvements in instrumentation are essential for pushing the boundaries of SECM capabilities. Innovations in microfabrication techniques and materials science are enabling the development of advanced microelectrodes with tailored properties. These electrodes can provide finer spatial resolution and enhanced selectivity for target analytes, aiding in more precise measurements.
Furthermore, the automation of SECM setups is also becoming a focal point. Automated systems can facilitate high-throughput screening and data collection, making SECM a more viable choice for large-scale studies. This development addresses a common limitation of traditional SECM setups, which often require extensive manual intervention.
Another significant advancement is the integration of machine learning algorithms for data analysis. Utilizing these algorithms can streamline the interpretation of complex electrochemical data, allowing researchers to derive meaningful insights faster. This is particularly relevant as the volume of data generated by SECM continues to grow.
Potential Novel Applications
There is a wealth of untapped potential within SECM that researchers are beginning to explore. One promising area is its application in studying living cells and their microenvironments. SECM can provide insights into cellular metabolism and interaction with electroactive substrates, contributing to advancements in drug delivery and therapeutic strategies.
Additionally, SECM has the potential to play a crucial role in the development of energy storage systems. By examining the electrochemical processes at play in batteries and supercapacitors, researchers can optimize material properties and enhance performance.
Nanotechnology is another field poised to benefit from SECM. The technique can facilitate the characterization of nanoscale materials and devices, helping scientists understand their electrochemical properties more comprehensively.
The exploration of novel applications for SECM reflects a growing interest in applying this powerful technique to solve pressing scientific challenges.
Finale
The conclusion serves as a crucial element in this article, encapsulating the insights gained throughout the exploration of scanning electrochemical microscopy (SECM). In this section, the impact and relevance of the topics discussed are synthesized, emphasizing their importance in both scientific inquiry and practical applications.
Summary of Key Insights
Throughout the article, we have delved into the foundational principles and intricate methodologies that underpin SECM. One of the main insights is its ability to probe electrochemical processes at nanometer scales, which facilitates a better understanding of materials and their interactions in various environments. The diverse applications detailed include advancements in materials science, biology, and environmental studies, highlighting SECM's versatility. These insights underscore how important SECM is for research aimed at solving complex challenges in contemporary science.
- SECM enhances spatial resolution for electrochemical investigations.
- Its applications are widespread, influencing various disciplines.
- Understanding electrochemical processes is critical for technological advancements.
Final Thoughts on SECM's Impact
Looking ahead, the impact of SECM goes beyond its current capabilities. It has the potential to integrate with other analytical techniques, leading to innovations that could redefine our understanding of chemical and biological systems. The advancements in instrumentation and the exploration of novel applications suggest a promising future for SECM.
"SECM is not merely a tool but a gateway to discovering new phenomena in electrochemistry, making significant contributions to both academia and industry."