Exploring Light's Role in Scientific Research
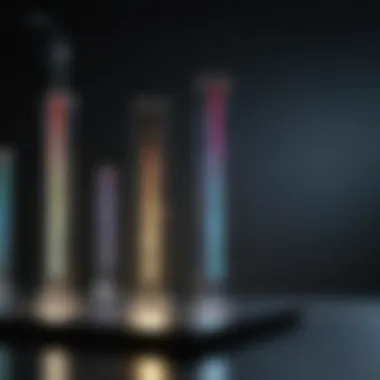
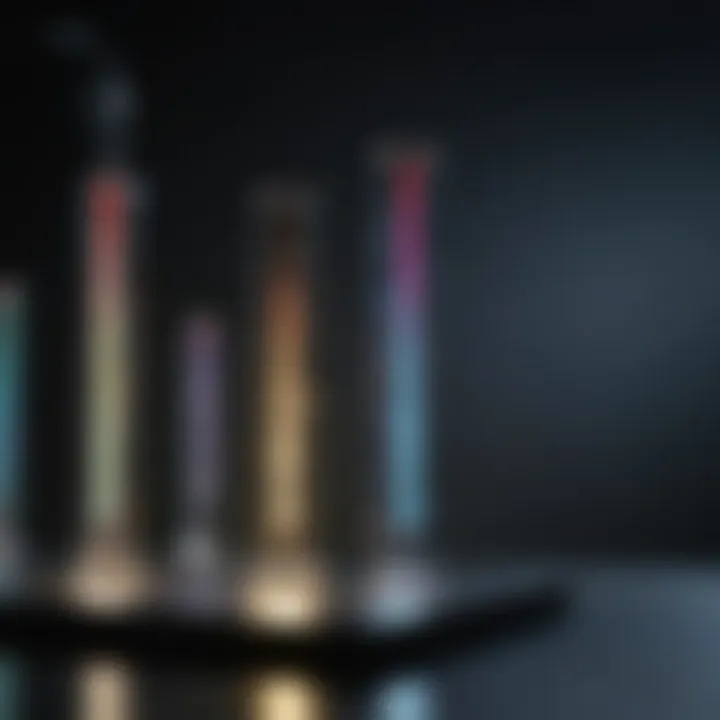
Intro
Light plays a crucial role in the understanding and advancement of various scientific fields. Its applications range from fundamental research to practical methodologies that pave the way for significant discoveries. Examining the application of light in scientific research provides insight into its multifaceted role in enhancing our comprehension of the natural world.
In this article, we will explore how light is utilized as an essential tool across disciplines such as biology, chemistry, physics, and earth sciences. The following sections will guide you through the historical context, key findings, and ongoing trends shaping the use of light in research environments. This overview aims to meet the interests of students, researchers, educators, and professionals who seek to deepen their knowledge in this vital area of study.
Background and Context
Overview of the Research Topic
The study of light application in research examines various ways in which light interacts with matter. This encompasses both the measurement and manipulation of light to explore diverse phenomena. Light has become indispensable due to its unique properties, such as wavelength and frequency. These properties allow scientists to delve into intricate details of biological processes and chemical reactions among others.
Historical Significance
Historically, the use of light in scientific inquiry can be traced back to early experiments with optics and astronomy. Scientists like Isaac Newton laid the groundwork for understanding light's nature and behavior. Over time, techniques such as spectroscopy emerged. Spectroscopy has enabled researchers to analyze materials based on their interaction with different light frequencies.
By the late 19th and 20th centuries, advancements in laser technology expanded the applications of light in research. This led to new methodologies in microscopy and photonics, where light plays a central role in gathering, manipulating, and analyzing data. Today, interdisciplinary approaches harness various types of light, enabling breakthroughs that were previously unattainable.
"The application of light in scientific research has evolved dramatically, influencing the way we perceive and investigate our universe."
Key Findings and Discussion
Major Results of the Study
The findings surrounding light application reveal significant advancements in several fields:
- In biology, techniques like fluorescent microscopy allow for the observation of cellular components with high resolution.
- In chemistry, laser-induced fluorescence spectroscopy is used to identify chemical species with remarkable sensitivity.
- In physics, experiments involving coherent light, such as those using lasers, have been pivotal in understanding quantum systems.
Detailed Analysis of Findings
The exploration of light's capabilities has proven tremendously valuable in generating new insights. For instance, in biological research, light can tag specific cellular proteins, providing clearer visualization of complex interactions within cells. Similarly, in chemistry, different wavelengths can stimulate reactions that are otherwise difficult to observe, revealing otherwise hidden aspects of chemical behavior. This intersection of light and innovative methodologies highlights not only the versatility of light but also its necessity for advancing scientific knowledge.
By synthesizing the information presented, researchers can cultivate a more nuanced understanding of how light is integral to scientific research. As this article continues to unfold, the intricate relationships between light and scientific inquiry will become clearer, illuminating the path for future advancements.
Prolusion to Light Application
Light plays a crucial role in scientific research, serving as both a tool and a subject of inquiry. The significance of understanding light application is multifaceted, encompassing its various forms and the methodologies that utilize it across different scientific disciplines. This section delves into the conceptual framework surrounding light application and its historical context.
Conceptual Framework
At the core of light application lies its ability to interact with matter, influencing everything from biological processes to chemical reactions and physical experiments. Understanding this interaction is essential for researchers, educators, and students alike. Light can be categorized into two major types: natural and artificial. Natural light, like that from the sun, initiates fundamental processes such as photosynthesis in plants. In contrast, artificial light, which includes incandescent bulbs and lasers, enables new research methodologies and experimental setups.
These different forms of light not only affect outcomes in experiments but also determine the accuracy of data collection, analysis, and interpretation. Therefore, establishing a solid framework for understanding light application is vital for any scientific inquiry.
Historical Perspective
The history of light application in science traces back to early civilizations. The study of light dates to ancient Greek philosophers who pondered its nature and behaviors. The inception of optics by scholars such as Euclid laid foundational principles, which later evolved through the work of pioneering figures like Isaac Newton and Albert Einstein.
In the 19th and 20th centuries, advancements in technology led to significant breakthroughs in how light is used. The invention of the microscope transformed biological research, enabling scientists like Antonie van Leeuwenhoek to discover microorganisms. Additionally, innovations in spectroscopy provided chemists with new tools to analyze molecular structures. Through these periods, the understanding of light became increasingly sophisticated, influencing various scientific areas deeply.
"The application of light in research has transitioned from simple observation to complex analysis, reflecting its vital importance in the scientific method."
In summary, the exploration of light application is critical, offering insights into its varied roles across multiple fields. Understanding its conceptual and historical frameworks is the first step towards comprehending its modern applications and future directions.
Types of Light in Research
Understanding the types of light used in research is essential for grasping its applications across disciplines. Light serves as both a tool and a variable in many experiments, influencing outcomes and providing data essential for scientific inquiry. Differentiating between natural and artificial light sources allows researchers to select appropriate methods for their specific needs. Natural light can often yield insights into biological systems, while artificial light, with its controlled outputs, is indispensable for precision in various experimental frameworks.
Natural Light
Natural light, stemming from the sun, plays a critical role in several branches of scientific research. It is most prominently applied in biological studies, particularly those examining photosynthesis. Plants rely on sunlight to convert carbon dioxide and water into glucose, a fundamental process in the ecosystem. Researchers have utilized natural light to monitor growth patterns, examine flowering times, and investigate the effects of light quality on plant physiology.
Using natural light also invites variables; cloud cover and seasonal changes can alter light availability. This is a significant consideration when planning experiments. Researchers must factor in these elements to ensure comprehensive and reliable results. Field studies with natural light present both unique advantages and challenges that scientists must navigate effectively.
Artificial Light
Artificial light offers a broad spectrum of possibilities within scientific research. It enables researchers to create consistent conditions, manipulate light parameters, and conduct experiments without relying on external environmental factors. Below are three primary types of artificial light utilized in research:
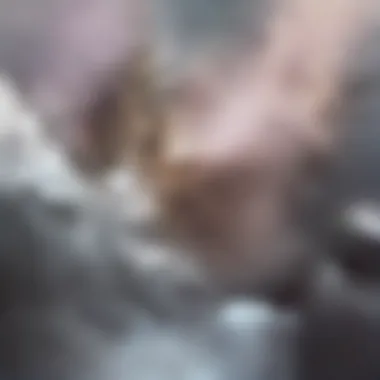
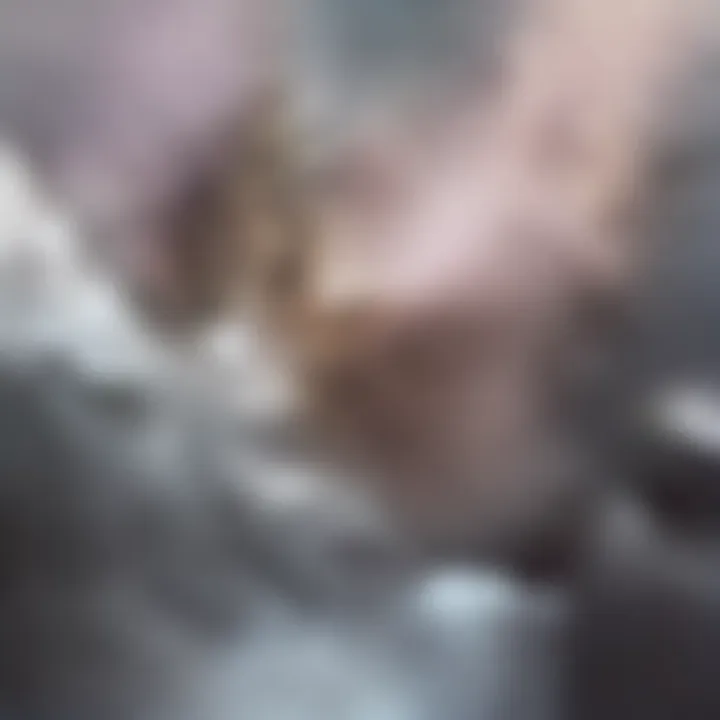
Incandescent Light
Incandescent light, generated by heating a filament, has a simple and effective design. This type of light is common in laboratory settings due to its ease of use and historical significance. Incandescent bulbs produce a warm color temperature, which can be beneficial for certain biological experiments, such as plant growth studies focusing on spectrums that favor specific growth stages.
However, incandescent light is not very energy efficient. Their lifespan is shorter compared to modern alternatives, which raises considerations regarding both cost and sustainability in research environments. Despite these drawbacks, researchers still widely use incandescent light in applications where a consistent light output is essential.
LED Light
Light Emitting Diodes (LEDs) are becoming increasingly prominent in scientific research due to their energy efficiency and versatility. LEDs can emit specific wavelengths of light, allowing researchers to tailor applications to their experimental needs. For example, in plant research, LEDs can be configured to emit light best suited for photosynthesis.
LED lights have a long lifespan and produce less heat than incandescent bulbs, minimizing risks to sensitive biological specimens. Nonetheless, they may have higher initial costs. The ongoing advancements in LED technology continue to enhance their effectiveness and expand their applications.
Lasers
Lasers represent one of the most precise forms of artificial light used in scientific research. They produce coherent light, allowing for focused applications in fields such as spectroscopy and microscopy. Lasers enable researchers to examine samples at a microscopic level, providing insights into materials and biological structures that would be difficult to obtain through conventional light sources.
A unique feature of lasers is their ability to reach specific wavelengths, improving the accuracy of measurements and enabling detailed analysis. Lasers are incredibly useful but can be expensive and require careful handling. Safety considerations surrounding their use are paramount, and proper training is necessary for all laboratory personnel.
The choice of light type directly impacts experimental outcomes and must be aligned with the research goals.
In summary, the types of light used in research, both natural and artificial, serve distinct roles in scientific inquiry. Adapting to the precise needs of an experiment is crucial, as the right choice can lead to significant advancements in understanding various fields of study.
Applications of Light in Different Sciences
The use of light in scientific research has transformed various fields significantly. This section explores how different sciences apply light in unique ways. By utilizing various light forms, researchers achieve more precise measurements, enhance visualizations, and facilitate complex analyses. Each discipline leverages light for specific purposes, yielding valuable insights that support advancements in knowledge and technology. The applications demonstrate not only utility but also the foundational role of light in scientific discovery.
Biological Research
Photosynthesis Studies
Photosynthesis is a fundamental biological process where light serves as the primary energy source. This study focuses on how plants and certain microorganisms convert light into chemical energy. A key characteristic of photosynthesis studies is that they provide insights into ecosystem dynamics and energy flow.
The use of light in these studies allows for the observation of plant responses under different light conditions, lending itself to various experiments. Photosynthesis research has a significant impact on understanding climate change, biodiversity, and food production. It is a popular choice in research due to its relevance and the urgency of food security amid growing populations.
A unique feature of photosynthesis studies is the ability to utilize different light wavelengths to enhance plant growth. However, one disadvantage involves the complexity in replicating natural conditions accurately in laboratory settings. Nonetheless, using light effectively leads to meaningful discoveries in biodiversity and sustainability.
Microscopy Techniques
Microscopy techniques utilize various light sources to magnify and visualize small specimens. This aspect makes microscopy vital for biological research. The ability to study cells, tissues, and microorganisms in detail has transformed biology.
In this context, the key characteristic of microscopy is its capacity to visualize structures that are otherwise invisible. Popular methods like fluorescence microscopy exploit specific light wavelengths to illuminate samples, revealing intricate details about living cells. This enables the study of processes such as cell division and organism development.
Microscopy techniques have unique features, including the ability to analyze live specimens in real time. However, they can become costly and may have limitations in resolution and sample preparation. Despite this, the advantages it provides in understanding biological processes outweigh the challenges, making it essential in research.
Chemical Analysis
Spectroscopy
Spectroscopy is a method used to analyze the interaction between light and matter. This specific aspect is crucial for understanding the molecular structure and composition of substances. Its key characteristic rests in its versatility across different chemical contexts, allowing for the study of solids, liquids, and gases.
The reason spectroscopy is a popular choice in research is due to its high sensitivity and ability to provide precise quantitative measurements. This technique often utilizes various light sources, including lasers, for enhanced precision. Unique to spectroscopy is its application across diverse fields, from environmental monitoring to astrobiology.
However, a disadvantage is the requirement for highly controlled environments, as external factors can influence results. Despite this, spectroscopy remains a powerful tool for gaining insights into chemical properties and reactions, validating its integral role in the scientific inquiry.
Chromatography
Chromatography separates components of a mixture based on their interactions with stationary and mobile phases. This specific aspect is relevant to chemical analysis, enabling the identification and quantification of substances. A key characteristic is its ability to handle complex mixtures efficiently, which is essential in many chemical investigations.
Chromatography is beneficial due to its ability to provide high-resolution separations. Different types, such as gas chromatography and high-performance liquid chromatography, utilize light detection methods to enhance sensitivity. This makes it invaluable in quality control and forensic applications.
Unique features of chromatography include its versatility and adaptability to different substances. However, it may require substantial time for the separation process, which can be limiting. Regardless, its advantages in resolving complex mixtures establish it as a cornerstone of chemical analysis.
Physical Experiments
Optics Experiments
Optics experiments focus on the behavior of light in various media and its interacting phenomena. The specific aspect here is understanding light propagation, reflection, and refraction. A key characteristic of optics experiments is the direct application of theory in practical settings.
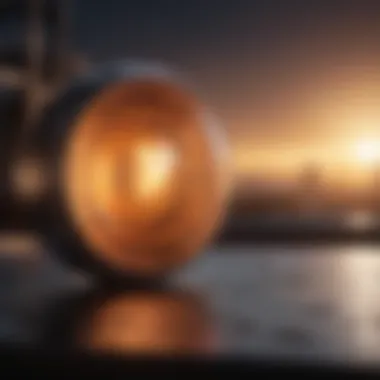
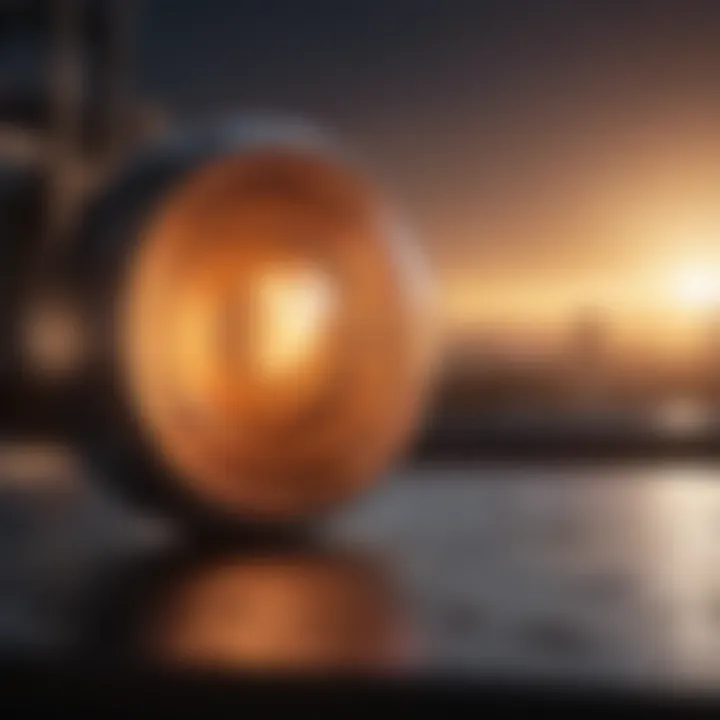
These experiments are widely embraced in physics due to their fundamental role in understanding wave phenomena. They provide insights into the principles that underlie much of modern technology. The unique feature is their applicability in everything from designing lenses to studying fundamental particles.
The disadvantage includes potential alignment complexities and environmental dependencies that may affect outcomes. Nevertheless, the foundational knowledge and discovery potential from optics experiments maintain its significance in research.
Quantum Physics Applications
Quantum physics applications explore light's behavior at a quantum level. The specific aspect involves phenomena like wave-particle duality and quantum entanglement. A key characteristic is the challenge it presents to classical intuitions about light and matter interactions.
This area is revered for its contributions to advanced fields like quantum computing and photonic technologies. A unique feature is its predictive power, facilitating discoveries like lasers and quantum algorithms. However, the mathematical complexity can be a barrier for understanding.
Despite this, the advantages stemming from quantum physics applications have far-reaching implications, reinforcing its critical role in advancing scientific frontiers.
Earth Science Exploration
Remote Sensing
Remote sensing utilizes light to gather information about the Earth's surface. This specific aspect is crucial for monitoring environmental changes and geological formations. The key characteristic is its ability to collect data over large areas quickly.
Remote sensing is vital in today's research landscape due to its application in disaster management, urban planning, and climatology. Its unique feature lies in the integration of different light wavelengths to assess land use, vegetation health, and atmospheric conditions. However, the expense associated with satellite technology and data interpretation can pose challenges.
Regardless, the wealth of information remote sensing provides justifies its expansive use in earth sciences and environmental studies.
Environmental Monitoring
Environmental monitoring examines light's interaction with ecosystems to gauge overall health. This specific aspect is integral to understanding ecosystems' responses to natural and anthropogenic changes. A key characteristic is its ability to synthesize information from multiple sources, including satellite data and ground surveys.
This method is a popular choice in research, as it helps track pollution levels, climate change effects, and biodiversity loss. Its unique feature is the use of interdisciplinary approaches, combining biology, chemistry, and physics for comprehensive assessments.
However, challenges may arise in obtaining timely data and maintaining accuracy over time. Nevertheless, the insights gained from environmental monitoring are invaluable, supporting efforts toward conservation and sustainable practices.
Methodologies for Light Application
The methodologies for light application in scientific research are vital for ensuring the precise and effective use of light across various disciplines. These methodologies span the planning, execution, and analysis phases of research. Understanding how to properly design experiments utilizing light can significantly enhance the accuracy of outcomes. Moreover, they provide researchers with the tools needed to leverage the unique properties of light for their specific objectives.
Experimental Setup
The experimental setup is the foundation of any research project involving light. It involves designing the physical arrangement in which light is utilized. Researchers must consider several factors when setting up their experiments. This includes the source of light, the materials being studied, and the intended measurements.
For example, in biological research, using lasers in microscopy requires careful alignment and calibration. Precise positioning ensures that the right amount of light illuminates the sample for optimal imaging. Furthermore, the choice of light filters can dramatically affect results by only allowing certain wavelengths to pass through.
A well-thought-out setup can minimize variables that might skew results and lead to inaccurate conclusions.
Data Collection Techniques
Data collection is a critical phase in light application research. Various techniques are employed, depending on the discipline and objectives of the study. For instance, in chemical analysis, spectroscopy is often used to collect data on how substances absorb or emit light. This allows chemists to determine the composition of materials.
In biological studies, techniques such as flow cytometry rely on light to analyze cellular characteristics. Fluorescent markers can tag specific molecules to provide more detailed data. Each technique comes with its benefits and limitations. Selecting the right one is crucial for achieving reliable and actionable data.
Analysis and Interpretation
After collecting data, the interpretation phase involves analyzing the information in context with the research questions. This requires a solid understanding of both the methodologies used and the phenomena being studied.
Data analysis often involves statistical methods to determine significance and reliability. For example, researchers might use regression analysis to understand relationships between light exposure and biological response. Understanding how to interpret these results is equally important. Misinterpretation can lead to erroneous conclusions, affecting future research and applications.
"The application of light in experimental settings is not just about measurement; it is about understanding the implications of those measurements in the broader context of scientific inquiry."
Overall, the methodologies for light application are integral to conducting thorough and credible research. They encompass an array of practices that ensure researchers can manipulate and study light as a tool for discovery.
Advancements in Light Technology
Advancements in light technology represent a significant frontier in the realm of scientific research. The pace of discovery and innovation is making light one of the most versatile tools available to researchers. This section delves into two main areas: Innovations in Lighting and Integration with Digital Tools. Both are instrumental in enhancing the methodologies and applications of light across various scientific disciplines.
Innovations in Lighting
The progress in lighting technology has led to the development of new tools that profoundly impact scientific research. Innovations like LED and laser systems have redefined how properties of light can be harnessed. LEDs are particularly notable due to their energy efficiency and longevity. Their spectral specificity enables precise applications in biological studies, like phototherapy and plant growth.
Moreover, laser technology continues to push boundaries, especially in applications that require focus and intensity. Laser-induced fluorescence, for instance, has transformed imaging techniques in cellular biology by allowing for high-resolution studies of cellular processes.
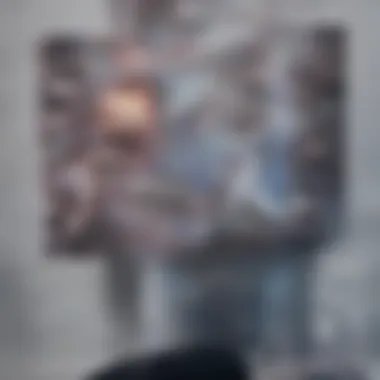
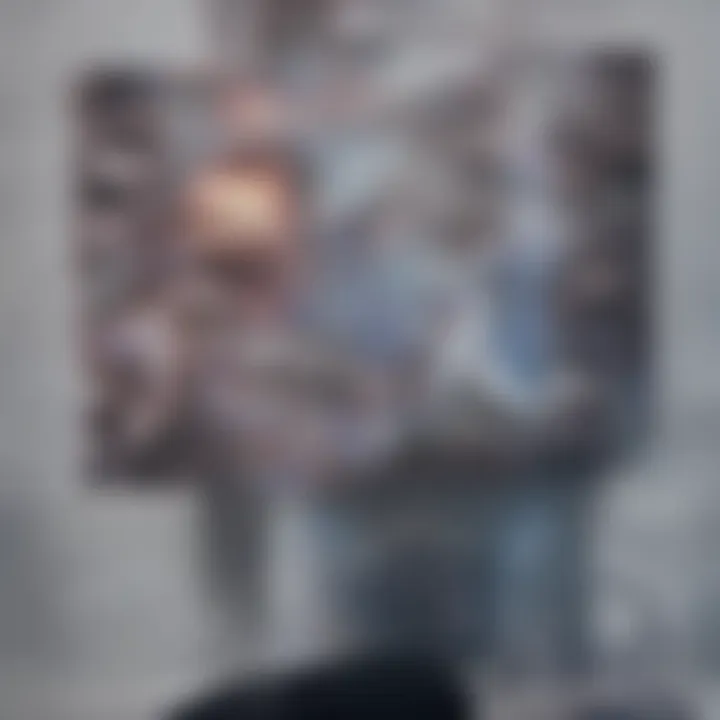
- Benefits of Innovations:
- Increased energy efficiency.
- Greater precision in measurement and application.
- Versatility across different scientific domains.
"The evolution of lighting technology is not just about illumination; it is enabling discoveries that were previously out of reach."
Integration with Digital Tools
The convergence of light technology and digital tools has produced a powerful synergy that enhances experimental capabilities. Advanced imaging systems and software are now able to analyze light data in real-time, providing immediate feedback to researchers. This integration streamlines processes and enhances data accuracy.
For example, software that utilizes artificial intelligence (AI) is increasingly being used to interpret complex data from light-based experiments. Machine learning algorithms can detect patterns that human analysts may miss, leading to faster and more robust conclusions from research.
- Key Considerations:
- Effective training and understanding of digital tools are essential for maximizing the benefits of this integration.
- Compatibility between different types of light tools and software must be ensured to facilitate seamless data flow.
Challenges and Limitations of Light Application
The application of light in scientific research offers myriad benefits. However, it also faces certain challenges and limitations that can impact its effectiveness and reliability. Understanding these limitations is crucial for researchers and educators, as it shapes the methodology and interpretation of results across different disciplines. The challenges can stem from technical constraints as well as interpretative difficulties that may arise during studies. These factors are vital for optimizing light use and accurately assessing its role in scientific inquiry.
Technical Constraints
Technical constraints are often a primary concern when applying light in research settings. Such limitations may restrict the experiments and affect outcomes. Some of these constraints include:
- Equipment Limitations: Research often relies on specialized instruments designed for specific light applications, such as photometers and spectrometers. Not having access to advanced or calibrated equipment can yield inaccurate results.
- Intensity Variation: The inconsistency in light intensity can pose challenges. Variations may occur due to environmental conditions or equipment settings, leading to unreliable data.
- Wavelength Specificity: Different scientific studies demand specific wavelengths of light. The inability of some sources to produce desired wavelengths can limit experiments. This is evident in fields like spectroscopy, where precise wavelengths are crucial.
These constraints highlight the need for researchers to carefully consider equipment and conditions under which light will be applied in their experiments. Addressing these technical challenges can enhance reliability and reproducibility in research findings.
Interpretative Difficulties
Interpretative difficulties present additional challenges when applying light in scientific research. Misinterpretation of data can lead to erroneous conclusions, which may misguide further studies. Several key elements contribute to these difficulties:
- Complex Interactions: Light interacts with materials in complex ways, sometimes producing unexpected results. Researchers may misinterpret these interactions or overlook influencing factors.
- Data Analysis: Analyzing data affected by light application can be intricate. Researchers must be proficient in understanding data algorithms and statistical methods used to draw conclusions. Misunderstanding these can skew results and interpretations.
- Environmental Influences: External factors, such as atmosphere and surrounding substances, can distort light propagation. These influences can lead to misinterpretation of how light impacts experimental variables.
Understanding these interpretative challenges is essential for producing valid scientific outcomes. Researchers must develop methodologies that account for these issues to ensure accurate data interpretation.
"The challenges of light application require critical assessment of both equipment and environmental factors."
In summary, while light application in scientific research is invaluable, it is important to acknowledge and mitigate the associated challenges. By addressing technical constraints and interpretative difficulties, researchers can improve the robustness of their studies. This ongoing evaluation is necessary for advancing knowledge across diverse scientific fields.
Future Directions in Light Research
The exploration of light application in scientific research is constantly evolving. Recognizing future directions in this domain can greatly influence various scientific disciplines. Innovations in light application not only enhance research methodologies but also open pathways for groundbreaking discoveries.
Emerging Trends
In recent years, several trends have emerged, changing the landscape of light application in research. Noteworthy trends include:
- Development of Advanced Light Sources: New technologies in light sources, such as ultrafast lasers and high-intensity LED systems, are pushing the limits of what is possible in various fields. These sources offer improved precision and control, enabling researchers to conduct more accurate measurements.
- Quantum Light Technologies: Quantum computing and quantum optics are gaining traction. Here, light plays a crucial role, especially in the development of quantum communication systems. Researchers are now exploring the potential of entangled light states to create faster and more secure transmission methods.
- Integration of Artificial Intelligence: AI-driven techniques are becoming common in analyzing light data. Machine learning algorithms help in interpreting complex datasets derived from light applications in imaging and spectroscopy, thus increasing the efficiency of research processes.
These trends highlight the importance of staying updated on technological advancements in light application, as they can substantially influence the course of scientific study.
Interdisciplinary Approaches
Future research will likely emphasize interdisciplinary collaboration. The convergence of various scientific fields can lead to more comprehensive studies of light application. Important considerations include:
- Collaboration Between Fields: Partnerships between physicists, biologists, and chemists can greatly enhance research outcomes. For example, integrating biology and physics can improve optical imaging techniques, providing clearer insights into cellular structures and processes.
- Shared Resources: Developing shared equipment and platforms among different research teams allows for cost-effective and efficient use of resources. For instance, specialized light microscopy facilities can be utilized by various departments.
- Holistic Understanding of Light: An interdisciplinary approach fosters a more holistic understanding of light. Recognizing its impact across sciences ensures advances are made not only in theoretical frameworks but also in practical applications.
"The integration of diverse expertise leads to richer insights and innovative solutions in light research."
Overall, the future of light application in research is promising. As researchers navigate these emerging trends and interdisciplinary collaborations, they may unlock new potentials in understanding the complexities of natural phenomena.
Finale
The conclusion of this article emphasizes the pivotal role light plays across various scientific domains. It integrates all previous discussions by synthesizing key insights and highlighting the innovations that grow from understanding light applications. Researchers must recognize that light is not merely an environmental factor but an essential tool that influences experimental design and outcome.
Summary of Insights
Throughout this article, we explored how light serves as both a medium and a method in scientific inquiry. From biological research, where it aids in photosynthesis studies and microscopy techniques, to chemical analysis through spectroscopy and chromatography, light's utility is vastly demonstrated. Different types of light, such as natural and artificial forms, each bring unique advantages.
The technological advancements in light applications enhance research capabilities. Using LEDs and lasers has led to stronger methodologies in experimental setups, data collection, and interpretation. Additionally, hurdles like technical constraints and interpretative challenges remind us that while light can illuminate countless phenomena, it can also obscure understanding under certain conditions.
"Light is a unifier in research, bridging disciplines and fostering collaboration toward discovery."
Final Thoughts
In considering future directions, the emerging trends signal a shift toward interdisciplinary approaches. For example, biological and chemical disciplines can merge through the application of light in analytical techniques. Understanding how light interacts in various contexts will drive further discoveries and innovations. Continually expanding our comprehension of light application enriches all fields of science and enhances our ability to tackle complex challenges. As researchers navigate these intersections, they must also stay attune to ethical considerations and sustainability, ensuring that light as a tool fosters a positive impact on scientific progress.
The importance of light applications in research cannot be overstated. It holds the potential to unravel many mysteries of the natural world, facilitating deeper understanding and broader perspectives in scientific discourse.