Exploring Cancer Genetics: Course Outline for Enthusiasts
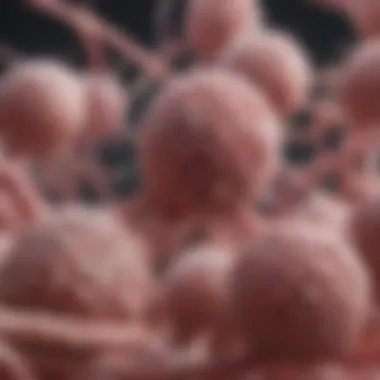
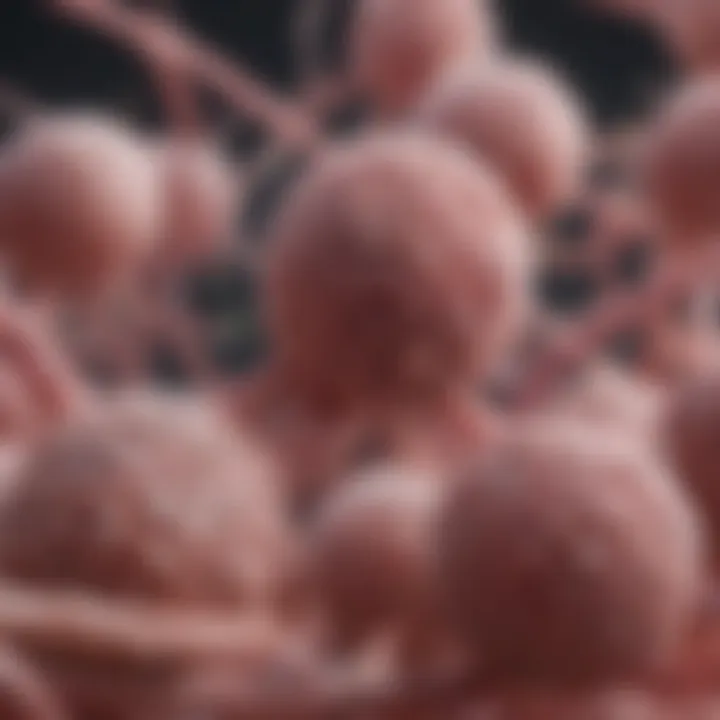
Intro
Cancer genetics is a critical field in understanding how genetic factors contribute to the development and progression of various cancers. This comprehensive course outline serves to illuminate the complex interplay between genetics and oncology.
By examining both foundational concepts and the most recent advancements, this outline aims to educate and inform an audience keen on exploring these themes in depth. As research in cancer genomics progresses rapidly, it is essential to grasp not just the current scientific knowledge but also the historical context that shaped our understanding.
Background and Context
Overview of the Research Topic
Cancer genetics focuses on the study of how genes influence cancer risk, its progression, and the response to treatments. The genome consists of numerous genes that can mutate, leading to uncontrolled cell growth. This outline will explore genetic susceptibilities, hereditary cancers, and advances in genomics. Various facets of cancer research, such as personalized medicine, gene editing technologies, and biomarker discovery, will also be highlighted.
Historical Significance
The exploration of cancer genetics has a substantial historical context. In the early 20th century, researchers started to identify the link between genetics and cancer. Pioneers like Theodor Boveri proposed the chromosomal basis of cancer in 1914. Significant milestones followed, including the discovery of oncogenes and tumor suppressor genes in the 1970s and 1980s.
"Understanding the genetic basis of cancer allows for breakthroughs in targeted therapies and encourages the pursuit of personalized treatment protocols."
Key Findings and Discussion
Major Results of the Study
The field of cancer genetics has led to major findings that have changed treatment landscapes. Identifying specific genetic mutations in cancers has enabled development of targeted therapies. For instance, HER2-positive breast cancer is treated with trastuzumab, pharmaceuticals tailored to unique genetic profiles.
Detailed Analysis of Findings
Research indicates that about 5-10% of all cancers are hereditary. Genes such as BRCA1 and BRCA2 are significant in breast and ovarian cancer risks. Furthermore, genetic testing has become vital in early detection and preventive strategies. This information not only aids in personalizing treatments but also provides insights into familial roles in cancer susceptibility.
Preamble to Cancer Genetics
Cancer genetics represents a vital field of study that bridges genetics and oncology. Understanding cancer through a genetic lens offers insights into the underlying mechanisms that drive cancer development, treatment response, and patient prognosis. As the field of genetics advances, the implications for cancer research and therapy become increasingly significant. The evolution of cancer genetics involves analyzing an array of genetic factors that contribute to tumorigenesis and may assist in creating personalized treatment strategies. Thus, exploring cancer genetics not only enhances our comprehension of the disease itself but also improves diagnostic and therapeutic approaches.
Definition and Importance of Cancer Genetics
Cancer genetics focuses on the role of genetic mutations and polymorphisms in the development of cancer. By defining cancer genetics, we recognize that numerous types of cancer are influenced by hereditary factors, environmental exposures, and somatic mutations. The identification of specific genetic markers associated with various cancer types has transformed the landscape of cancer treatment and management.
Understanding cancer genetics is essential because it helps identify individuals at increased risk for certain cancers. Genetic testing can offer insights that guide decisions about screening and preventive measures. Moreover, knowledge of specific genetic mutations can inform the choice of targeted therapies, leading to more effective and personalized treatment plans. The crucial intersection between genetics and cancer underscores the need for continued research in this area, as it holds the potential to advance both prevention and treatment.
Overview of Cancer Mechanisms
To fully appreciate the impact of genetics on cancer, it is critical to understand the basic mechanisms that underlie tumorigenesis. Cancer typically arises from a series of genetic alterations that disrupt normal cellular processes. These alterations can affect several areas:
- Cell growth regulation: Mutations in genes that control cell division can lead to uncontrolled cell proliferation.
- DNA repair mechanisms: Defects in genes responsible for fixing DNA errors can accumulate damage, launching carcinogenesis.
- Programmed cell death: Dysregulation of apoptosis can permit abnormal cells to survive and divide, contributing to tumor formation.
In summary, the interplay of these mechanisms provides a foundation for comprehending how genetic alterations lead to cancer's diverse phenotypes. By mapping out these pathways, researchers can identify potential targets for therapy and develop more effective treatment modalities.
Basic Genetic Principles
Understanding basic genetic principles is crucial in the study of cancer genetics. This section lays the groundwork for comprehending how genes influence the development and progression of cancer. It encompasses essential topics such as DNA structure and function, as well as gene expression and regulation.
Fundamentally, genetics provides insight into the biological systems that govern cellular processes. By exploring these basic principles, one can appreciate how alterations at the genetic level can lead to abnormal cell behavior, a hallmark of cancer. This understanding is especially relevant for students, researchers, and professionals involved in oncology and genetic counseling.
DNA Structure and Function
DNA, or deoxyribonucleic acid, is often termed the blueprint of life. Its structure is organized as a double helix, consisting of nucleotides that comprise a phosphate group, a sugar, and a nitrogenous base. There are four types of bases: adenine, thymine, guanine, and cytosine. The sequence of these bases encodes the genetic information used in the growth, development, and functioning of organisms.
The function of DNA extends beyond mere storage of information. It serves as a template for the synthesis of RNA, and subsequently proteins, through processes known as transcription and translation. This protein synthesis is vital for cell structure and function, making DNA integral to maintaining normal cellular activities. Understanding the nuances of DNA is critical for identifying mutations that can contribute to cancer.
In cancer genetics, mutations may occur in the DNA sequence due to various factors, including environmental influences or inherited genetic predispositions. These changes can lead to the malfunctioning of key regulatory genes, prompting uncontrolled cell division.
"DNA structure provides the building blocks that determine cellular behavior. Any disruption can result in significant pathological outcomes."
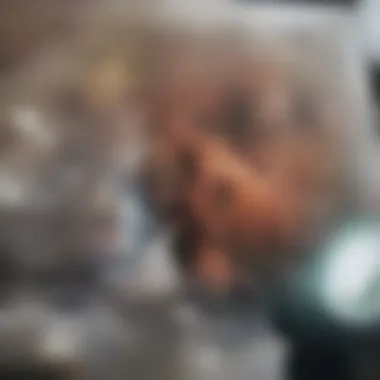
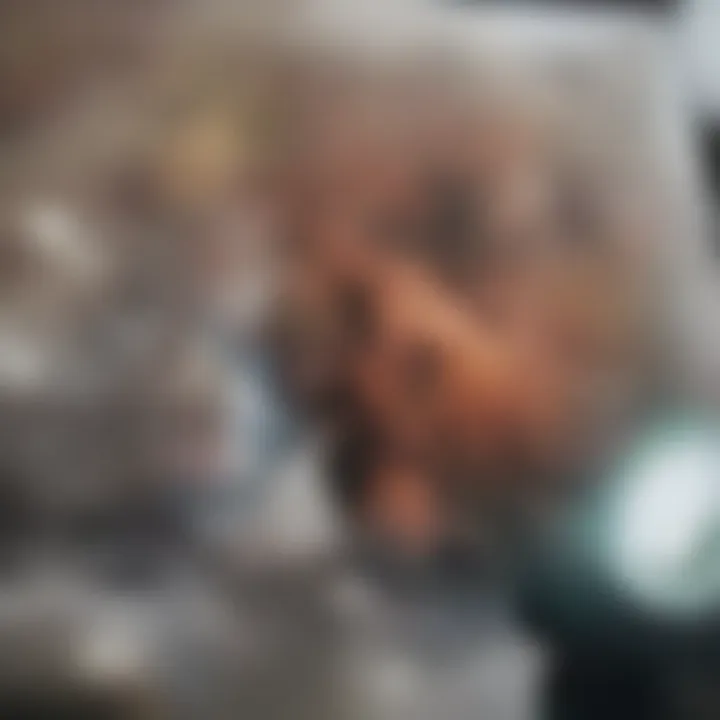
Gene Expression and Regulation
Gene expression refers to the process through which information from a gene is used to synthesize functional products, primarily proteins. This process is tightly regulated, allowing cells to respond to various internal and external cues. The regulation occurs at multiple levels, including transcriptional, post-transcriptional, translational, and post-translational.
Key players in this regulation include transcription factors, enhancers, and repressors, which interact with the gene’s promoter region to increase or decrease gene expression. Furthermore, epigenetic modifications—such as DNA methylation and histone modification—can influence gene expression without altering the underlying DNA sequence. Such changes are particularly relevant in the context of cancer, as aberrant regulation of gene expression can lead to oncogene activation or tumor suppressor gene silencing.
In sum, the study of gene expression and regulation not only illuminates the normal functioning of cells but also highlights the potential pathways through which cancer can arise. Understanding these concepts equips researchers and clinicians with the knowledge needed to develop targeted therapies and interventions in cancer treatment.
Types of Cancer and Their Genetic Basis
Understanding the types of cancer and their genetic basis is highly important in the study of cancer genetics. Cancer is not a single disease but rather a group of diseases characterized by uncontrolled cell growth. Genetic alterations play a vital role in the development and progression of these diseases. Therefore, it's crucial to recognize how different cancers can originate from distinct genetic causes. This section will explore inherited cancer syndromes as well as sporadic cancers resulting from mutations, providing a comprehensive understanding of their significance.
Inherited Cancer Syndromes
Inherited cancer syndromes are genetic conditions that increase an individual's risk of developing specific types of cancer. These syndromes often arise from mutations in certain genes passed down through families. Notable examples include the BRCA1 and BRCA2 genes, associated with breast and ovarian cancers, as well as the Lynch syndrome, linked to colorectal and endometrial cancers.
The study of these syndromes provides insights into how particular genetic factors influence cancer susceptibility. Understanding inherited cancer risk is vital for both patients and healthcare providers. It enables targeted screening and preventive strategies, such as increased surveillance or prophylactic surgeries for those at higher risk. Additionally, genetic counseling plays an important role in informing families about their potential risks and available testing.
Important Note: Individuals with a family history of cancer should consider genetic testing. It can reveal inherited mutations that may not only affect themselves but also their relatives.
Sporadic Cancers and Mutations
Sporadic cancers, in contrast, occur due to genetic mutations that are not inherited but rather acquired during a person's lifetime. These mutations can arise from various factors including environmental exposures, lifestyle choices, or even natural aging processes. Examples of sporadic cancers include most cases of lung, breast, and prostate cancers.
The understanding of sporadic cancers is crucial for developing effective prevention and treatment strategies. Distinguishing between inherited and sporadic cancers helps researchers identify which genetic mutations may inform clinical decisions. For instance, identifying actionable mutations can guide targeted therapies that significantly improve patient outcomes. Furthermore, studying sporadic cancers helps researchers discover potential biomarkers that may aid in early detection and diagnosis, thus enhancing treatment success.
Genomic Technologies in Cancer Research
Genomic technologies serve as a cornerstone in cancer research today. They provide critical insights into the genetic underpinnings of various cancer types. Understanding these technologies is essential for researchers and clinicians alike, as they enable the identification of mutations, gene expression profiles, and pathways involved in tumorigenesis. In this rapidly advancing field, genomic technologies offer remarkable benefits for diagnosis, treatment, and personalized medicine.
Next-Generation Sequencing
Next-generation sequencing (NGS) represents a significant advancement in genomic technologies. Unlike traditional sequencing methods, NGS allows the simultaneous sequencing of millions of DNA fragments. This capability facilitates a thorough examination of entire genomes or targeted gene panels, offering a comprehensive view of the genetic landscape of tumors.
Key aspects of NGS include:
- Speed: The rapid processing time reduces the duration needed to generate genomic data.
- Cost-effectiveness: Advancements in technology have led to decreased costs, making large-scale sequencing projects more feasible.
- High throughput: Researchers can analyze vast amounts of information at once, leading to a richer dataset for understanding cancer biology.
The implications of NGS in cancer research are profound. Not only does it aid in identifying somatic mutations, but it also enhances our ability to detect and analyze rare variants in tumors.
Genomic Profiling and Biomarkers
Genomic profiling is the analysis of genetic variations that are associated with cancer. This method is crucial for the identification of biomarkers, which can serve as indicators of disease presence and progression.
Biomarkers can be used for:
- Diagnosis: Certain genetic markers can assist in recognizing specific cancer types.
- Prognosis: Profiling can provide insights into the likely course of the disease, helping in predicting outcomes for patients.
- Therapeutic decisions: Identifying actionable mutations helps in selecting targeted therapies tailored to individual patients.
"Genomic profiling transforms our approach to cancer treatment, allowing for a more individualized strategy."
With the continuous improvement of genomic technologies, the integration of genomic profiling into routine clinical practice is on the rise. This progression promises to refine our understanding of cancer and improve outcomes for patients by aligning treatment with their unique genetic profile.
Molecular Pathways in Cancer
Molecular pathways are integral to our understanding of cancer. They involve a series of biochemical interactions that regulate the growth, survival, and proliferation of cells. These pathways can become dysregulated, leading to uncontrolled cell division and cancer progression. Thus, comprehending molecular pathways in cancer is crucial for developing effective treatments and therapies. The implications of these pathways extend not only to therapeutic interventions but also to preventative strategies.
Oncogenes and Tumor Suppressor Genes
Oncogenes and tumor suppressor genes represent two essential categories of genes involved in cancer biology.
- Oncogenes are mutated forms of normal genes (proto-oncogenes) that promote cell growth and division. When mutated, they can lead to uncontrolled cell proliferation. Examples of oncogenes include KRAS and MYC, which play significant roles in various cancers such as pancreatic and breast cancer.
- Tumor suppressor genes, on the other hand, typically function to restrain cell growth, induce apoptosis, and promote DNA repair. When these genes are inactivated through mutations, the cell cycle can proceed unchecked, leading to tumor growth. The most well-known tumor suppressor gene is TP53, which is frequently mutated in a range of cancer types.
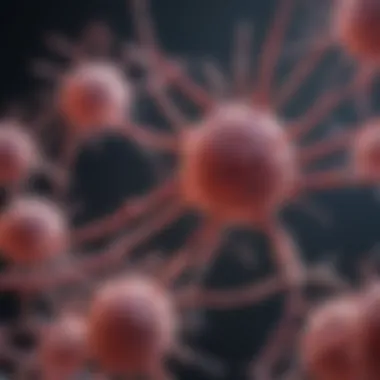
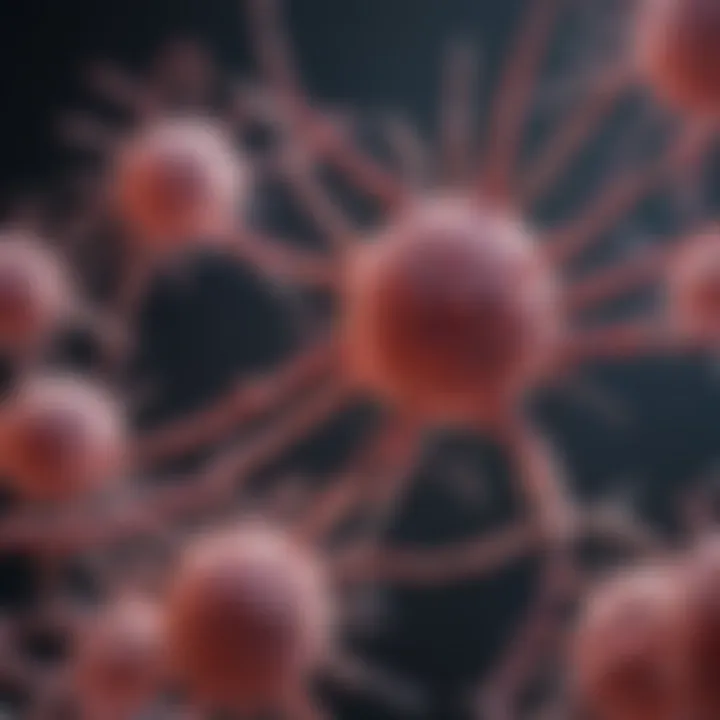
Understanding the interplay between oncogenes and tumor suppressor genes is crucial for grasping how cancers develop and progress. This knowledge is fundamental as it forms the basis for targeted therapies aimed at correcting these genetic malfunctions.
Signaling Pathways and Their Role in Tumorigenesis
Signaling pathways are critical for a variety of cellular functions. In the context of cancer, these pathways often become aberrant.
- Growth Factor Signaling: Growth factors bind to their receptors, triggering a cascade of intracellular signals that promote proliferation. If this pathway is hyperactivated, it can lead to uncontrolled cell growth.
- Apoptotic Pathways: These pathways control programmed cell death. Dysregulation can result in cancer cells evading apoptosis, thereby contributing to tumor survival.
- Cell Cycle Regulation: This involves checkpoints that ensure cell division occurs accurately. Failures in these checkpoints can lead to genomic instability and cancerous growth.
- DNA Damage Response: Signaling pathways detect and repair DNA damage. Ineptitude in this response can result in mutations accumulating, increasing cancer risk.
"Understanding molecular signaling pathways is crucial for elucidating the mechanisms behind tumour progression and targeted therapy development."
In summary, molecular pathways in cancer encompass the interactions of diverse genes and signals that govern cellular behaviors. By examining oncogenes, tumor suppressor genes, and their associated signaling pathways, researchers can unravel the complexities of cancer biology. This exploration not only enhances our scientific understanding but also drives forward the development of innovative therapies, paving the way to more personalized and effective cancer treatments.
Epigenetics and Cancer
Epigenetics is a crucial field of study in cancer genetics, focusing on how gene expression is regulated without alteration of the underlying DNA sequence. This discipline uncovers the layers of complexity that influence whether genes are turned on or off. Understanding epigenetic mechanisms is essential for developing effective cancer therapies and improving patients’ outcomes.
The significance of epigenetics lies in its ability to explain how environmental factors can affect gene expression and ultimately contribute to cancer development. Unlike genetic mutations, which are permanent changes to the DNA sequence, epigenetic modifications can be reversible. This offers promising avenues for targeted therapies that aim to correct these modifications in cancerous cells.
Many cancers have specific epigenetic signatures that distinguish them from non-cancerous tissues. Through the study of these signatures, researchers are able to identify potential biomarkers for early detection and prognosis.
Understanding Epigenetic Modifications
Epigenetic modifications primarily include DNA methylation, histone modification, and non-coding RNA molecules.
- DNA Methylation: This process involves adding a methyl group to the DNA molecule. Hypermethylation of tumor suppressor genes can prevent their expression, leading to unregulated cell growth and division.
- Histone Modification: Proteins called histones package the DNA into a compact structure. Modification of histones through acetylation or methylation can alter how tightly the DNA is wound. This, in turn, affects gene accessibility for transcription.
- Non-coding RNAs: These are RNA molecules that do not code for proteins but play a significant role in regulating gene expression at the transcriptional and post-transcriptional levels. Certain non-coding RNAs have been implicated in various cancer types, serving as potential therapeutic targets.
In summary, understanding these epigenetic modifications is vital because they provide insights into the mechanisms underlying cancer progression and can help in developing novel strategies for therapy.
Epigenetic Therapies in Cancer Treatment
Epigenetic therapies are emerging as a prominent approach in cancer treatment. By correcting abnormal epigenetic changes, these therapies aim to reactivate silenced genes or modify the expression of genes that contribute to cancer. There are several strategies being explored:
- DNA Methylation Inhibitors: Drugs like azacitidine and decitabine are designed to inhibit the methylation process, thereby reactivating tumor suppressor genes.
- Histone Deacetylase Inhibitors: These drugs target enzymes responsible for removing acetyl groups from histones. By inhibiting these enzymes, histone acetylation increases, which can lead to the activation of silenced genes critical for preventing tumor growth.
- Targeting Non-coding RNAs: Therapies aimed at modulating the expression of specific non-coding RNAs show promise in reestablishing normal gene function in cancer cells.
"The future of cancer therapy may well rest on understanding the intricate balance of epigenetic modifications that sustain or suppress tumor growth."
These therapies can potentially change the landscape of treatment for various cancers, providing options for patients who do not respond to traditional therapies. By focusing on the epigenetic aspects of cancer, researchers and clinicians hope to enhance the precision and effectiveness of cancer treatments.
Cancer Genomics and Personalized Medicine
Cancer genomics focuses on understanding the genetic factors that contribute to cancer development. This discipline plays a significant role in personalizing medicine, allowing for tailored treatment approaches based on an individual’s genetic makeup. The intersection of genomics and personalized medicine represents a shift from a one-size-fits-all approach to more targeted strategies, enhancing the effectiveness of cancer therapies.
Tailoring Treatment Plans Based on Genetic Information
One of the core tenets of personalized medicine is the concept of tailoring treatment plans using detailed genetic information obtained from patients. By analyzing the specific mutations and alterations present in a tumor's DNA, clinicians can choose targeted therapies that are more likely to be effective. For instance, patients with breast cancer can be screened for mutations in the BRCA1 or BRCA2 genes. If these mutations are present, they may benefit from targeted treatments such as PARP inhibitors that specifically act on such genetic profiles.
The benefits of this approach are significant:
- Increased Efficacy: Treatments designed with genetic data in mind can lead to better patient outcomes.
- Reduced Side Effects: Personalized therapies often come with fewer side effects compared to traditional treatments, which can affect non-cancerous cells.
- Cost-Effectiveness: By targeting therapies, patients may avoid ineffective treatments, reducing overall healthcare costs.
However, personalizing treatment does come with challenges. Not every patient can be matched with an effective targeted therapy, and the landscape of cancer genomics is constantly evolving. Continuous research is fundamental to identify new biomarkers that can inform treatment options.
Ethical Considerations in Genetic Testing
As with the advancements in cancer genomics, there are ethical concerns regarding genetic testing. Informed consent is paramount when it comes to testing for genetic mutations. Patients need to fully understand the implications of genetic findings, not only for their treatment but also for their family members, who may share similar genetic risks.
Some key ethical considerations include:
- Privacy and Data Security: Safeguarding genetic data is crucial to protect patients from discrimination or misuse of their genetic information.
- Access to Testing: Equitable access to genetic testing is necessary. Disparities in healthcare can lead to unequal treatment opportunities.
- Psychological Impact: Disclosure of genetic risks can cause emotional distress. Genetic counseling should accompany testing to support patients through their emotional and psychological responses.
"Understanding the ethical implications of genetic testing is as important as the science itself. It drives how society adopts and integrates these advancements in healthcare."
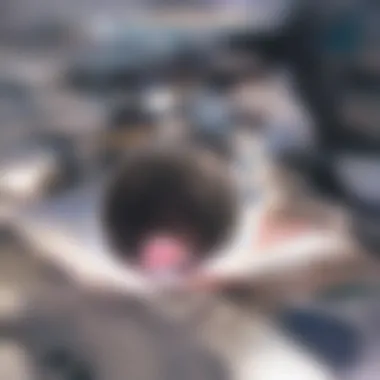
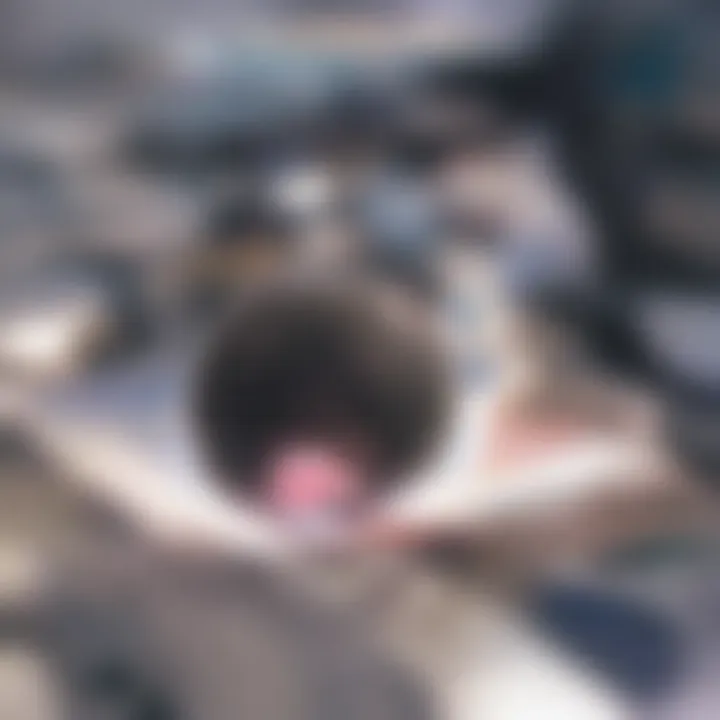
In summary, cancer genomics and personalized medicine represent a transformative era in cancer treatment. As we continue to explore and expand on genetic insights, the need for ethical frameworks will only grow, ensuring that advancements are not just scientific but also humane.
Current Research Trends in Cancer Genetics
The exploration of cancer genetics is continually evolving, reflecting the complexities found within the human genome and its interaction with environmental factors. Current research trends in cancer genetics are significant because they enhance our understanding of how genetic variations contribute to cancer development and progression. By tracking these trends, researchers can identify new pathways for diagnosis, treatment, and prevention. The depth of knowledge garnered from studies in this area not only benefits oncologists but also informs public health strategies aimed at reducing cancer incidence.
Innovative Approaches and Technologies
Innovative approaches and technologies are at the forefront of the research landscape in cancer genetics. Precision medicine is improving rapidly due to advancements in genomic sequencing, which allow for more accurate identification of genetic mutations associated with specific cancers. One potent example is the use of CRISPR technology, which facilitates targeted gene editing. Researchers can now modify genetic sequences to explore the impact of specific mutations on cancer characteristics.
Moreover, artificial intelligence plays a crucial role in analyzing vast amounts of genetic data. Algorithms can detect patterns that may go unnoticed by human researchers, leading to new insights about cancer mechanisms. Other technologies such as liquid biopsies are being developed to obtain genetic information from blood samples, offering a non-invasive method to monitor cancer progression and response to therapy.
Through innovation, we unlock possibilities for understanding specific genetic drivers of tumors, leading to more focused treatments.
Collaborative Research Efforts
Collaborative research efforts are essential in cancer genetics due to the multifactorial nature of cancer. Multi-institutional studies combine resources from various research centers to increase the sample size, thereby improving the reliability of findings. Such collaborations can include partnerships between universities, hospitals, and biotechnology firms. This collective approach allows for sharing of knowledge, methodologies, and technological resources.
An example of successful collaboration is the International Cancer Genome Consortium, which focuses on mapping the genomic changes in multiple cancer types. This consortium exemplifies how collaborative efforts not only help identify cancer drivers but also establish standardized methodologies for genomic studies globally.
In addition, interdisciplinary partnerships between geneticists, clinicians, and bioinformaticians foster a holistic understanding of cancer. These collaborations pave the way for translating genomic discoveries into clinical applications, ensuring that patients benefit from the latest research findings in real-time.
The trends presented here signify a shift toward a more integrated, research-focused approach in understanding cancer genetics. They encourage a mindset of collaboration, innovation, and application, which are necessary to tackle the complexities of cancer.
Case Studies in Cancer Genetics
Case studies play a crucial role in the field of cancer genetics. They provide tangible examples that showcase how genetic factors influence cancer development and patient outcomes. Through these detailed analyses, we can gain insights into the complexities of various cancer types and their genetic underpinnings. The examination of real-world cases enables researchers and students to connect theoretical knowledge with practical applications. This understanding is vital for advancing both research and clinical practices in oncology.
Analysis of Specific Cancer Types
When analyzing specific cancer types within case studies, it is important to look at various factors. This includes understanding the genetic mutations associated with each cancer, the population affected, and the clinical implications of these genetics. For example, breast cancer has well-studied genetic mutations such as BRCA1 and BRCA2. These mutations significantly increase the risk of developing breast and ovarian cancers. Another example is colorectal cancer, where mutations in genes like APC and KRAS have been identified.
Case studies in these areas demonstrate the variations in genetic alterations across different populations. Such diversity can inform targeted therapies and personalized medicine approaches.
Key points to analyze include:
- Mutation Analysis: Review of specific mutations related to cancer development.
- Environmental Factors: Consider how lifestyle or environmental factors may impact genetic predispositions.
- Treatment Responses: Understanding how genetic background can affect responses to specific cancer treatments.
Lessons Learned from Genetic Research
The lessons learned from genetic research are numerous and significant. They highlight both the potential and the limitations of genetic insights in cancer treatment. One major takeaway is the concept of personalized medicine. By understanding a patient’s unique genetic profile, clinicians can tailor treatments that offer better efficacy and reduced side effects.
From various case studies, researchers have also recognized the potential of genetic testing in high-risk populations. For instance, families with known hereditary cancer syndromes can benefit from genetic counseling and proactive management strategies.
However, it is also essential to consider the ethical implications of genetic research. Issues such as data privacy and the psychological impact of genetic knowledge must be addressed. Thus, the interpretation and application of findings from case studies ultimately contribute not just to medical advances, but also to a broader understanding of genetics within a societal context.
"Case studies serve as a bridge between research and clinical practice, grounding genetic theories in the lived experiences of patients."
Future Directions in Cancer Genetics
The field of cancer genetics is rapidly evolving, influenced by technological advancements and novel research findings. It is critical to explore future directions in this area as it has profound implications for understanding disease mechanisms and improving patient outcomes. The relevance of focusing on future directions lies in its potential to guide research priorities, inform clinical practices, and enable personalized medicine approaches. As we venture further into the genomic era, the integration of emerging technologies into cancer genetics will likely reshape our understanding and treatment of various cancer types.
Emerging Technologies and Their Impact
Emerging technologies are key drivers in the evolution of cancer genetics. They allow researchers and clinicians to gain deeper insights into the genetic underpinnings of cancer. Some specific technologies that are shaping the future include:
- Single-cell sequencing: This technology permits the analysis of individual cells, revealing cellular heterogeneity that can influence treatment responses.
- CRISPR-Cas9 gene editing: Widely recognized for its potential in therapeutic applications, CRISPR allows for precise modifications to DNA, facilitating better understanding of cancer gene function.
- Artificial Intelligence (AI) and machine learning: These technologies enhance data analysis capabilities, identifying patterns in large-scale genomic data, which can lead to new predictive models for cancer behavior and therapy responses.
The implications of these technologies are vast. For instance, single-cell sequencing can advance our grasp of tumor microenvironments, thus improving the development of targeted therapies. Meanwhile, AI can assist in predictive analysis, optimizing treatment plans based on a patient’s specific genetic profile.
"The use of emerging technologies in cancer genetics stands to unlock new avenues for both research and treatment, emphasizing the necessity for continuous investment in these innovations."
Integration of Genetic Data in Clinical Practice
The integration of genetic data into clinical practice represents a significant shift in oncology. Personalized medicine uses genetic insights to tailor treatments to individual patients. This approach takes into account the unique genetic makeup of the tumors and the patients, improving therapeutic efficacy and reducing unnecessary side effects.
Considerations for integrating genetic data include:
- Data management: Efficient systems need to be established to handle and analyze vast amounts of genetic data. Proper data management ensures that relevant information is accessible to healthcare providers in practice.
- Clinical guidelines: Developing clear guidelines is essential for the application of genetic information in patient care. These guidelines must balance scientific knowledge with clinical applicability, ensuring that genetic data informs real-world treatment plans.
- Training for health professionals: As genetics becomes more embedded in cancer treatment, healthcare professionals require ongoing education regarding genetic interpretation and its implications for patient care.