Enhancing Solar Cell Efficiency: Key Insights and Strategies
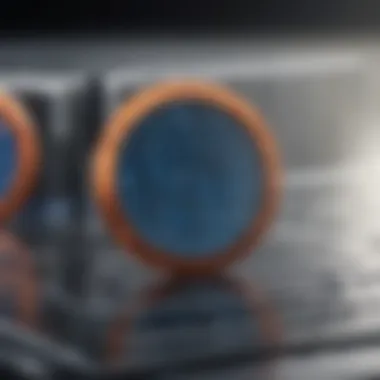
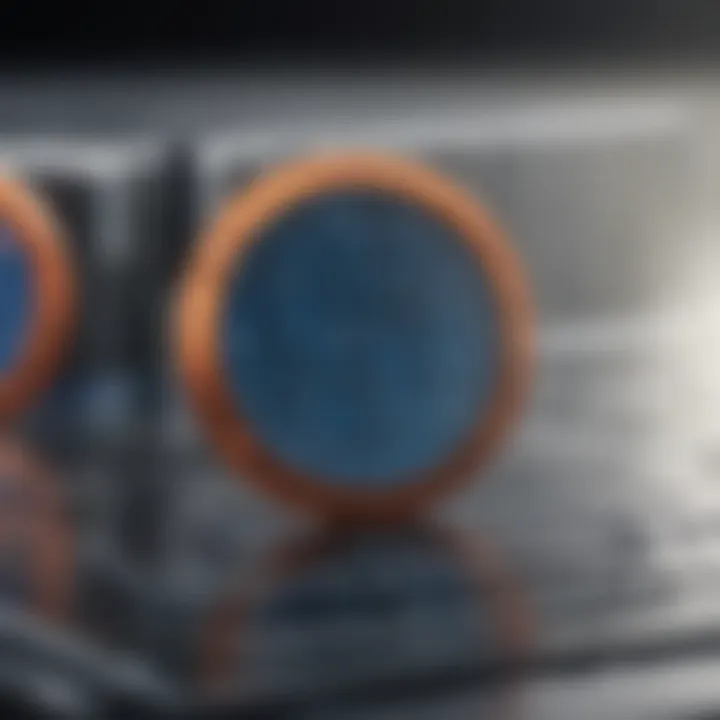
Intro
Solar energy has gained significant traction over the years, becoming a focal point in discussions about sustainable energy solutions. As the world grapples with the challenges of climate change and finite fossil fuel resources, improving the efficiency of solar cells emerges as a pressing concern for scientists and engineers alike. This section sets the stage for exploration into a variety of strategies and insights that are shaping the future of solar technology.
Background and Context
Overview of the Research Topic
The quest for maximizing solar cell efficiency encompasses numerous avenues of study and innovation. Researchers are continually looking for ways to improve solar panel design, materials, and technology that can enhance energy conversion rates. Scholars advocate that by optimizing these factors, we can not only make solar energy a more viable alternative but also contribute significantly to the global effort aimed at reducing greenhouse gas emissions.
Historical Significance
Historically, the journey of solar energy dates back to the 19th century when scientists first discovered the photovoltaic effect. Over the decades, various technological advancements transitioned solar energy from a niche technology to a key player in energy production. Major milestones include the introduction of silicon-based solar cells in the 1950s, which laid the groundwork for the modern solar industry. Current findings, such as the development of perovskite solar cells and bifacial designs, highlight just how far we've come—and they foreshadow the potential advancements that lie ahead.
"Improving the efficiency of solar cells is not just about technology—it's a multi-dimensional challenge that intersects with economics, environmental science, and social issues."
Key Findings and Discussion
Major Results of the Study
Recent research underscores several promising strategies for enhancing the efficiency of solar cells. Key findings reveal that:
- Material Innovations: Shifts from traditional silicon to emerging materials like perovskites or organic photovoltaics show an impressive spike in performance.
- Enhanced Design Techniques: Advances such as bifacial panels, which collect sunlight from both sides, maximize light absorption and improve overall efficiency.
- Integrated Energy Systems: Combining solar cells with battery storage or smart grid technology can significantly improve energy yield during non-peak hours.
Detailed Analysis of Findings
Each of these areas offers a treasure trove of possibilities for enhancing solar efficiency. For instance, the exploration of perovskite materials has shown that they can be produced at a lower cost, with a potential for higher efficiency compared to traditional materials. Furthermore, bifacial panels have been found to increase energy production by up to 30% in some conditions due to their ability to capture reflected sunlight.
Also noteworthy is the role of public policy and investment in driving these technologies forward. Increased government incentives for solar adoption and research can create an ecosystem conducive to innovation. Overall, the intersection of advanced material science, engineering improvements, and supportive policy frameworks serves as a summary of the pathways needed to achieve maximum solar cell efficiency.
Understanding Solar Cell Efficiency
Grasping solar cell efficiency isn't just a scientific exercise; it's a vital part of harnessing renewable energy effectively. As the world pivots toward greener solutions, understanding how to maximize this efficiency becomes paramount. By delving into efficiency, we lay the groundwork for better energy conversion, cost-effectiveness, and ultimately, a sustainable future. The intricacies of solar cell performance can dictate how much energy can be captured from sunlight, and that plays a central role in enhancing overall energy systems.
Defining Efficiency in Solar Technology
Efficiency in solar technology essentially refers to the ratio of sunlight converted into usable electrical energy. To put it simply, it's the percentage of solar energy that a solar cell can convert into electricity. If a solar panel has an efficiency of 20%, that means 20% of the sunlight striking it generates electricity we can harness. Understanding this ratio is critical for evaluating different solar technologies and materials. Moreover, efficiency is often influenced by multiple factors, including sunlight intensity, temperature, and the composition of the materials used in the solar cells.
There are several ways to analyze efficiency:
- Conversion Efficiency: This is a standard measure of how well the solar panel converts sunlight into electricity.
- Energy Yield: This measures the energy output over time in relation to the input of solar energy.
- Temperature Coefficient: Efficiency fluctuates with temperature, and knowing how a specific panel reacts to heat can inform decisions.
The push for improvement in these metrics has led to innovative designs and materials, all aimed at boosting this efficiency rating.
The Role of Photovoltaics
Photovoltaics (PV) are the heart and soul of solar technology; they're essentially the gateway that converts sunlight into electricity. At its core, a photovoltaic cell is made from semiconductor materials, most commonly silicon. When sunlight hits the cell, it excites electrons in the material, generating a flow of electricity. This direct conversion process is what differentiates photovoltaics from other heat-based solar technologies.
The advantages of photovoltaics can’t be overstated:
- Simplicity in Design: Photovoltaic systems can be integrated easily into existing structures, from homes to large-scale solar farms.
- Scalability: From small installations to massive solar plants, the technology can be scaled to meet varying energy demands.
- Environmentally Friendly: PV offers a clean energy source that drastically cuts down on carbon emissions when compared to fossil fuels.
But photovoltaics are not without challenges. Factors such as installation costs, efficiency under different weather conditions, and energy storage capabilities play a significant role in their overall effectiveness. Therefore, mastering how photovoltaics work is essential for anyone looking to enhance solar energy systems or educate others about its benefits.
"Understanding solar cell efficiency is not merely a numbers game; it's about envisioning a sustainable energy future."
As we continue to assess and improve solar technologies, recognizing the nuances of solar cell efficiency will be critical for any future innovations.
Fundamentals of Solar Energy Conversion
Understanding the fundamentals of solar energy conversion is pivotal for grasping how we can leverage sunlight to generate power effectively. This topic forms the backbone of solar technology, as it allows us to examine the processes involved in transforming sunlight into electricity. By decoding these mechanisms, researchers and practitioners can isolate the variables that influence efficiency, leading to improved designs and greater energy yields. There are several specific elements we must delve into.
First, the mechanism of energy conversion is essential. This includes how photovoltaic cells absorb photons and convert the energy into electrical current. Understanding these mechanisms can inform strategies to enhance the performance of solar cells in real-world applications.
Next, the influence of the light spectrum cannot be overlooked. Not all wavelengths of light provide the same amount of energy in the conversion process. Each type of solar cell is designed to capture specific wavelengths more effectively, which means knowing how these factors interrelate is crucial for maximizing energy conversion efficiencies.
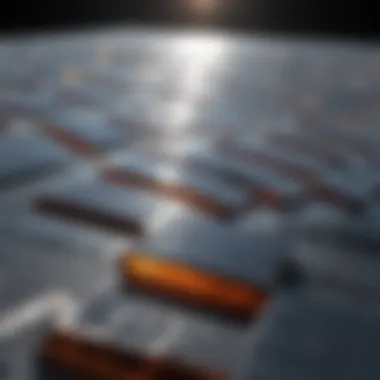
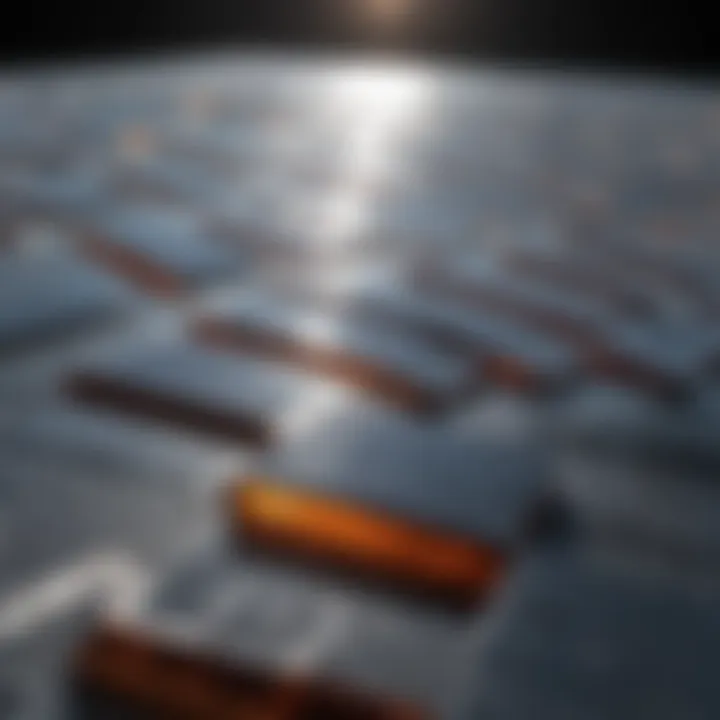
Ultimately, the fundamentals of solar energy conversion illustrate the relevance of material science and engineering practices in making solar technology more efficient.
Mechanisms of Energy Conversion
When we talk about mechanisms of energy conversion, we are stepping into the intricate ballet between sunlight and semiconductor materials. At its core, the process involves three steps:
- Photon Absorption: When sunlight hits a solar cell, photons are absorbed by the semiconductor material, usually silicon. This energy excites electrons, giving them enough energy to escape their atomic bonds.
- Electron Movement: Once freed, these energized electrons begin to flow through the material. This movement generates an electric current. The creative arrangement of layers within the cell, often including dopants, can direct the flow of these electrons.
- Electric Current Generation: The resulting flow of electrons, or current, can then be harnessed to power devices or feed into the electric grid.
Understanding these mechanisms in depth helps engineers tweak designs for better conductivity or reduce energy losses due to heat. Furthermore, enhanced knowledge can lead to the development of new materials that can augment or even replace traditional silicon in solar cells.
Influence of Light Spectrum
The light spectrum plays a significant role in determining how effectively solar cells convert sunlight into usable electricity. Not all light is equal, and solar cells are engineered to optimal performance based upon specific wavelengths of light.
- Visible Light Efficiency: Most conventional solar cells are designed to capture visible light, which comprises the majority of sunlight that reaches the surface of the Earth. However, only certain wavelengths in this spectrum will be effectively converted into electricity.
- Infrared and UV Capturing: Some advanced solar technologies are now attempting to utilize ultraviolet and infrared light, extending their operational efficiency. This involves materials that can respond to these frequencies either by direct absorption or through methods such as upconversion, where low-energy photons are converted into higher-energy ones.
- Solar Cell Types: Different types of solar cells respond variably across the spectrum. For instance, multi-junction solar cells can harness energy from multiple wavelengths, significantly improving their efficiency when compared to single-junction systems.
Understanding how light spectrum influences energy conversion is not only important for crafting better solar cells but can also aid in the selection of optimal installation sites where light conditions are most favorable. This thoughtful consideration can lead to significant improvements in energy yields across an array of applications.
"A deeper understanding of solar energy conversion mechanisms enables innovative advancements that can change the future of energy consumption."
By tackling these fundamentals, we lay a solid groundwork for further advancements in solar technology, ultimately leading to enhanced efficiency and sustainability in energy production.
Materials Impacting Solar Cell Performance
In the ever-evolving landscape of solar technology, the selection of materials plays a pivotal role in determining not just the efficiency but also the overall performance of solar cells. Each material interacts uniquely with light and influences factors like absorption, charge transport, and stability. Thus, understanding how these materials work together forms the crux of solar innovation.
The benefits of using high-quality materials are manifold. They can significantly enhance the photovoltaic effect, providing more electricity from the same amount of sunlight. However, certain considerations must also be taken into account, including cost, availability, and environmental impact.
"The materials we choose do not just dictate performance but also tell a story of sustainability and future resilience in energy production."
Silicon as a Dominant Material
Silicon has long been hailed as the backbone of the solar industry. Silicon solar cells account for over 90% of the market share, and for good reason. The properties of silicon—its abundance, stability, and relatively high efficiency—render it an ideal choice.
One of the pressing benefits of silicon is its effectiveness in converting sunlight into electricity, particularly when used in crystalline forms such as monocrystalline and polycrystalline cells. Monocrystalline panels tend to be more efficient and have a longer lifespan, while polycrystalline offers a more cost-effective yet slightly lower-performance alternative.
In addition, advancements in silicon technology, like passivated emitter rear cell (PERC) designs, are continuously pushing the boundaries of efficiency. This approach captures additional light absorption and reduces electron recombination, contributing to a marked increase in energy output. However, there’s a catch: while silicon excels in performance, it also comes with certain manufacturing challenges, notably energy-intensive production processes.
Emerging Materials: Perovskites
Perovskites have emerged onto the solar scene quite dramatically. With a unique crystal structure, these materials allow for a tantalizing combination of efficiency and low-cost manufacturing. Their potential for near-optimal light absorption and exciting charge transport properties have caught the eyes of researchers worldwide.
The efficiency of perovskite solar cells has surged in recent years, breaking records and challenging traditional silicon cells with efficiencies exceeding 25%. Nevertheless, their long-term stability remains a concern. The ability of perovskites to withstand environmental stressors such as humidity and temperature fluctuations is something that researchers are actively trying to enhance.
Additionally, the production of lead-based perovskites raises questions regarding environmental safety. Researchers are exploring lead-free alternatives, which, while promising, still require time to mature.
Role of Organic Photovoltaic Cells
Organic photovoltaics (OPVs) bring a unique flair to the solar material spectrum. Composed of carbon-based materials, these cells have the advantage of being lightweight, flexible, and easily integrated into various surfaces, from building-integrated photovoltaics (BIPV) to wearables. One of the standout features of OPVs is their potential for low-cost fabrication via printing techniques, which can minimize production costs substantially.
However, the efficiency of organic solar cells hasn’t quite reached the levels of silicon or perovskite yet, typically hovering around 10% to 15%. Yet, they shine in niche applications where flexibility and weight are key considerations.
The environmental footprint of organic materials is another critical factor, as many are derived from abundant sources and often biodegradable. This paves a way for a more sustainable approach to solar technology.
Through understanding the strengths and weaknesses of these various material types, we can better appreciate the complex landscape of solar energy technology and its future.
Innovative Designs in Solar Technology
In the realm of solar energy, innovative designs play a pivotal role in advancing efficiency and embracing the future of renewable power. As technology progresses, the focus shifts towards optimizing solar cells not only in terms of electricity production but also with respect to their adaptability and aesthetic integration. This section dives into groundbreaking concepts like bifacial solar panels, integrated solar cell systems, and tracking systems for solar panels, shedding light on how these advancements contribute to harnessing solar power more effectively.
Bifacial Solar Panels
Bifacial solar panels are like a breath of fresh air in a field often dominated by traditional designs. These panels are unique in that they can capture sunlight from both their front and back sides, effectively doubling their potential energy output. This capability is thanks to their transparent backing, which allows the panels to utilize reflected sunlight from surfaces beneath them, be it snow, sand, or water.
The benefits of bifacial technology are numerous:
- Increased Energy Production: By absorbing light from both sides, these panels can produce more energy, especially in reflective environments.
- Reduced Land Use: Their higher efficiency makes them a viable option for space-limited installations, allowing more energy generation in less area.
- Durability: Typically, they boast a longer lifespan due to their robust design and can withstand harsher conditions.
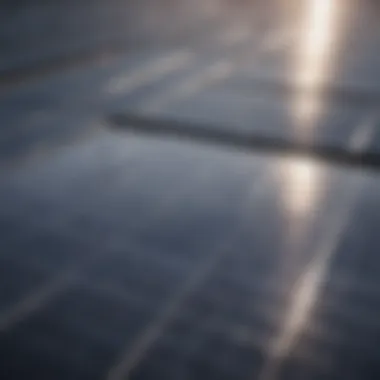
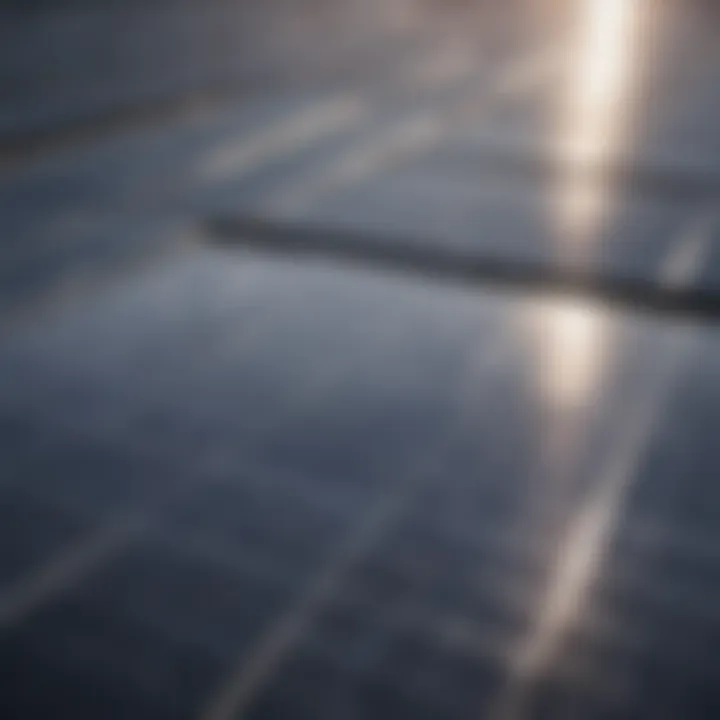
However, it's not all sunshine and rainbows. One must consider the initial cost and the need for suitable mounting systems and reflective grounds to maximize their potential. Managing these aspects is crucial for effective implementation in both urban and rural settings.
Integrated Solar Cell Systems
Integrated solar cell systems represent a harmonious marriage between technology and architecture. This concept involves embedding solar cells into building materials, such as roof tiles or windows, turning entire structures into active energy generators. This innovation not only optimizes space but also enhances the aesthetic appeal of buildings, often being more visually pleasing than bulky traditional panels.
Key features of integrated solar cell systems include:
- Seamless Aesthetics: They blend effortlessly into surfaces, making solar energy accessible without compromising the visual integrity of a structure.
- Energy Independence: By generating energy on-site, buildings can reduce reliance on external power sources, promoting sustainability.
- Minimal Installation: Due to their integration, these systems can often be installed during the construction phase, saving time and costs on retrofitting.
Despite these advantages, there are challenges as well. The efficiency of integrated cells tends to lag behind traditional panels, and careful material selection is essential to ensure that performance does not diminish. Moreover, upfront costs can be higher, which might deter some property developers.
Tracking Systems for Solar Panels
Tracking systems are akin to a spotlight following a performer on stage; they ensure solar panels are always oriented optimally towards the sun. By mechanically adjusting the angle of installation throughout the day, these systems can significantly enhance energy capture compared to fixed installations.
The benefits of employing tracking systems include:
- Enhanced Efficiency: Studies show that a single-axis tracker can boost production by up to 25%, while dual-axis trackers can increase it even further.
- Adaptability in Different Climates: They can optimize energy harvest in various climates, making them suitable for diverse geographical locations.
- Higher Performance in Lower Light: By maintaining an optimal angle, these systems capture more light during dawn and dusk, when sunlight is typically weaker.
However, they come with caveats; they require ongoing maintenance and can be more expensive upfront due to the additional machinery involved. For projects with a long-term outlook or those situated in areas with high solar potential, the investment can pay off significantly over time.
In summary, moving away from conventional frameworks toward innovative designs greatly enhances solar cell efficiency. By embracing technologies like bifacial panels, integrated systems, and clever tracking mechanisms, we are not just adapting to new needs but pioneering a future where solar energy is more effective and widespread, paving the way for sustainable energy solutions.
Improving Solar Cell Efficiency Through Engineering
Engineering plays a pivotal role in the quest to enhance solar cell efficiency. The developments within this field not only aim to increase the effectiveness of solar cells in converting sunlight into usable energy, but they also contribute to the overall sustainability of solar technology. By fine-tuning various engineering aspects, researchers can unlock new levels of performance.
Precision in Engineering Techniques
Improvements in engineering techniques can significantly influence the functionality of solar cells. These methods, such as nanostructuring and the application of antireflective coatings, yield substantial enhancements. Incorporating advanced materials and innovative designs into solar cell production leads to more efficient energy capture, minimizing losses and maximizing output.
Benefits of Enhanced Engineering
- Increase in Conversion Efficiency: Superior engineering techniques result in higher energy conversion rates. This means more of the sun’s energy is transformed into usable electricity.
- Cost Effectiveness: Although initial investment in advanced engineering solutions may be high, the long-term savings due to increased efficiency can be noteworthy. In terms of resource allocation, optimized engineering can lead to reduced operational costs.
- Broader Applications: With improved efficiency, solar cells can be deployed in a wider range of environments, making solar power a more viable option for various applications, including residential, commercial, and industrial sectors.
Nanostructuring Techniques
Nanostructuring techniques are among the most exciting frontiers in enhancing solar cell efficiency. By manipulating materials at a nanoscale level, engineers can create structures that interact more favorably with light, thus improving performance.
Understanding Nanostructuring
At the core of nanostructuring lies the ability to create minute patterns that can significantly impact how solar cells absorb light. This includes techniques such as:
- Nanowire Arrays: These structures enhance light absorption and can trap photons more efficiently.
- Quantum Dots: Incorporating these particles into solar technology can allow for better light conversion properties and tunable bandgaps.
By structuring materials on such a small scale, researchers can achieve surface roughness that increases the likelihood of light interacting with the cell. This optimizes the absorption of solar energy, translating into better overall efficiency.
"Nanostructuring represents not just an evolution, but a revolution in solar cell technology."
Antireflective Coatings
Antireflective coatings are crucial elements in the engineering of solar cells, designed primarily to minimize light reflection at the surface of the solar panel. If light reflects off the surface rather than being absorbed, efficiency suffers dramatically.
The Role of Antireflective Coatings
These coatings work by adjusting the refractive index of the surface, allowing incident sunlight to pass through more readily. Various materials can be used for these coatings, with silicon nitride and titanium dioxide being popular choices. Their design contributes significantly to managing how much light is absorbed versus how much is lost due to reflection.
Advantages of Using Antireflective Coatings
- Boosted Light Absorption: With less light reflected, more energy can enter the solar cell, enhancing its performance.
- Improved Aesthetic Qualities: These coatings also provide a uniform finish that can be more visually appealing for installations, especially in residential areas.
- Durability: High-quality antireflective coatings can also protect the solar cell from environmental degradation, extending its lifespan.
In essence, engineering enhancements, especially through nanostructuring and effective use of antireflective coatings, play a critical part in the ongoing endeavor to maximize the efficiency of solar cells. Through these methods, the solar energy sector is moving closer to achieving optimal performance.
Environmental and Economic Considerations
In the broader scope of solar energy adoption, the Environmental and Economic Considerations play a pivotal role. The intertwining of ecological awareness and economic viability significantly shapes the landscape of solar technology. Not only are these factors essential for understanding the sustainability of solar cells, but they also influence policy decisions and market trends. The pressing need to curtail carbon emissions, coupled with the quest for energy independence, makes this an indispensable discussion for stakeholders ranging from consumers to policymakers.
Lifecycle Assessment of Solar Technology
The lifecycle assessment (LCA) of solar technology provides a comprehensive overview of the environmental impacts associated with all stages of solar cell life—from raw material extraction to end-of-life disposal. This assessment is crucial for determining how solar energy compares to traditional energy sources. Here are some elements to consider:
- Raw Material Extraction: The mining of materials such as silicon, silver, and cadmium can lead to significant environmental degradation. Even though solar panels offer cleaner energy during use, the footprint left behind during manufacturing can be detrimental.
- Manufacturing Process: Energy consumed during the fabrication of solar cells is something to scrutinize. Advances in manufacturing techniques can mitigate these effects, using less energy and producing fewer wastes.
- Installation and Operation: The efficiency during installation and how the panels are maintained over time can influence overall energy output and effectiveness. If managed poorly, these factors can also bring additional costs.
- End-of-Life Management: Decommissioning solar panels can pose an environmental challenge if not handled properly. Recycling strategies and policies are imperative to minimize waste and recover valuable materials. By incorporating effective end-of-life solutions, we can significantly lower the net environmental impact.
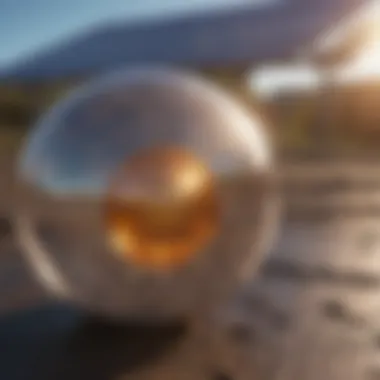
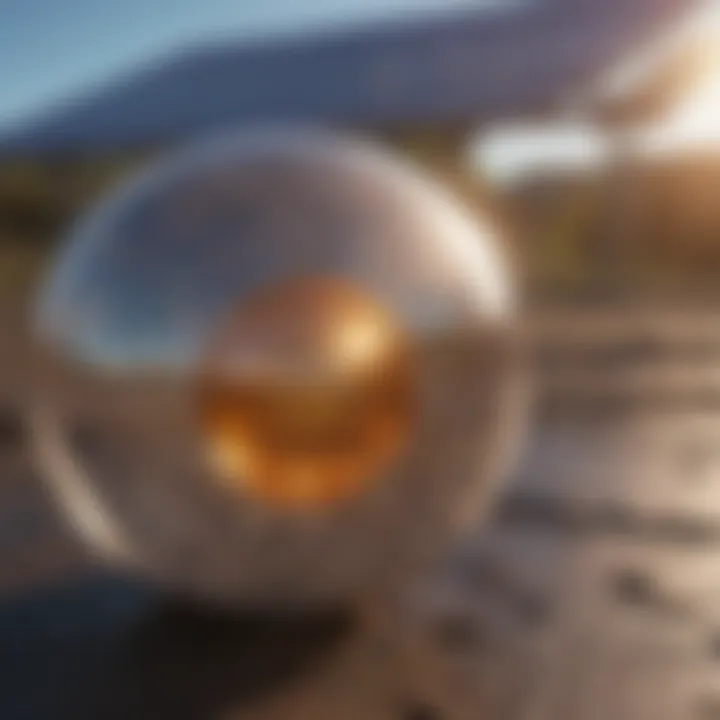
An insightful quote from the International Renewable Energy Agency highlights this aspect well:
"The transition towards sustainable energy is as much about stewardship of resources as it is about innovation."
Cost vs. Efficiency in Solar Panels
The age-old debate between cost and efficiency in solar panels is a vital consideration for anyone looking to invest in solar technology. It's not merely about choosing the cheapest option but understanding the long-term implications of those choices.
- Initial Costs: The upfront cost of solar panels can be daunting for many consumers. However, higher-efficiency models may require less space and fewer installations, affecting the overall investment in the long run.
- Operational Efficiency: Panels that initially appear more expensive might offer better energy return on investment due to enhanced performance in lower light conditions or higher conversion rates. Assessing operational efficiency can lead to better returns and sustainability.
- Maintenance Costs: Even cost-effective solar panels might require more frequent repairs or replacements, especially those on less established technology. Evaluating total lifecycle costs helps in making a well-informed decision.
- Incentives and Financing Options: Various governmental incentives exist to offset the initial investment in solar. These can significantly improve the cost-to-efficiency ratio by making higher-quality options more accessible.
Case Studies in Enhanced Efficiency
When it comes to enhancing solar cell efficiency, the exploration of real-world applications cannot be overlooked. Case studies provide a window into how theoretical concepts are applied practically, which often leads to breakthroughs that theoretical models alone cannot predict. These examples also serve as powerful illustrations of the benefits, challenges, and implications of adopting advanced solar technologies. Studying what has worked and what hasn’t is crucial for researchers and industry professionals aiming to refine their approaches and achieve better outcomes in solar energy solutions.
Successful Implementations Worldwide
Looking around the globe, several areas exemplify successful implementations of solar cell technology designed to maximize efficiency. Here are some notable cases:
- Germany's Energy Transition: Germany has become a frontrunner in solar energy adoption. By integrating large-scale solar farms and encouraging residential solar panel installations through favorable policies, the country has seen significant increases in energy efficiency. Notably, the 10-megawatt solar array in Freiburg demonstrates how regional collaboration can enhance energy production.
- California’s Solar Initiative: The California Solar Initiative, launched in 2006, encourages homeowners and businesses to invest in solar technology. By providing financial incentives and educational support, California has successfully increased solar panel installations, leading to reduced energy costs while emphasizing efficiency through innovative materials and designs.
- China's Solar Power Expansion: China has flooded the market with affordable solar modules through expanded production capacity. Projects like the Longyangxia Dam Solar Park demonstrate the effective mix of hydropower and solar to bolster efficiency in energy production, with designs allowing maximum sunlight capture throughout the day.
These cases underscore how comprehensive strategies can yield fruitful results by combining effectiveness with community and governmental support.
Failures and Lessons Learned
While positive case studies provide valuable insights, failures also contribute to a deeper understanding of solar cell efficiency impacts. Here are some lessons learned:
- Thin-Film Solar Panels in the USA: Several thin-film solar energy projects in the U.S. faltered due to inability to scale for lower costs. High manufacturing expenses combined with competition from crystalline silicon cells made these initiatives less viable, emphasizing the need for cost-effectiveness alongside efficiency.
- High-Profile Installations in Abu Dhabi: The Noor Abu Dhabi solar plant, while initially celebrated, faced challenges regarding performance efficiency that fell short of expectations. This case highlights the importance of rigorous performance testing prior to implementation and adaptability in project designs.
- Failures in Bifacial Projects: Some bifacial solar energy projects did not achieve performance results as anticipated. In certain locations, ground reflectivity and installation angles were not optimized, leading to inefficiencies. This stresses the need for thorough site analysis before project initiation.
By analyzing these failures, industry professionals gain insights into what pitfalls to avoid and how adaptive strategies can mitigate risks.
"The road to solar cell optimization is paved with both successes and missteps; each case provides lessons that guide future innovations."
In summary, investigating case studies allows us to appreciate the complex landscape of solar cell efficiency. Successful implementations offer pathways of progress, while failures shed light on potential drawbacks. Each instance brings us one step closer to realizing enhanced efficiency in solar technologies.
Future Directions in Solar Energy Technology
As the world steadily shifts toward renewable sources of energy, understanding the future directions in solar energy technology is pivotal. This exploration reveals innovative paths that promise to enhance not only the efficiency but also the accessibility of solar cells. With the pressure of climate change mounting, it’s imperative to consider how advancements in this field can provide sustainable solutions that align with global energy demands.
Advancements on the Horizon
The solar energy sector is on the cusp of several groundbreaking advancements, ripe for discussion. One such development is the push towards bifacial solar panels that capture sunlight from both sides, ultimately increasing energy absorption. Unlike traditional panels, these allow utilization of reflected light from the ground, contributing to a higher output without requiring a large footprint.
Another exciting area is the integration of perovskite materials into solar technology. These newly researched materials potentialy reach efficiencies surpassing their silicon counterparts while maintaining lower production costs. The removal of elaborate manufacturing processes seen with silicon may reshape how solar modules are constructed and installed.
Additionally, transparent solar cells are slowly gaining traction. Imagine windows that not only let in light but also convert sunlight into energy—a dual function that occupies no additional space. The development of these panels can transform buildings and urban areas into energy-generating structures, enhancing overall energy efficiency in cities.
Role of Artificial Intelligence in Optimization
Artificial intelligence (AI) is set to revolutionize solar energy, bringing along significant optimizations for solar cell performance. By using machine learning algorithms, researchers can predict the performance of solar cells under varying conditions. This predictive capability is invaluable for tailoring designs that maximize the energy harvest.
Moreover, AI can enhance energy management systems that control the distribution and storage of solar energy. With the integration of AI, the efficiency with which solar energy is stored and utilized can be improved, reducing waste and increasing reliability in energy supply.
Furthermore, AI-driven maintenance predictions are gaining attention. By utilizing sensors and data analytics, systems can forecast failures before they occur, significantly reducing downtime. This proactive approach decreases repair costs and ensures more continuous energy generation.
In summary, the future directions in solar energy technology consists of innovative advancements and the integration of artificial intelligence, both of which hold the promise of maximizing solar cell efficiency. As technology evolves, so too do the opportunities for integrating solar solutions into our daily lives, driving us towards a more sustainable future.
Culmination: The Path Ahead for Solar Cell Efficiency
The journey toward optimizing solar cell efficiency is far from over; it’s more like a winding road filled with opportunities and challenges. In this article, we’ve taken a deep dive into various strategies and insights that can enhance the efficacy of solar technologies. Looking ahead, we recognize the importance of continual innovation, investment, and integration of new ideas to push the boundaries of solar cell performance.
As we consider the future, several specific elements emerge that warrant attention. First, the quest for efficiency is not purely a technical endeavor; it’s also about marrying economics with science. The increasing demand for renewable energy sources means that the financial viability of solar projects is paramount. Finding ways to reduce costs while improving efficiency can make solar technology appealing to a broader audience, from residential homeowners to industrial giants.
Moreover, as the solar industry matures, there’s a real emphasis on sustainability beyond just energy conversion. Lifecycle assessments are becoming essential in evaluating the long-term impacts of solar technologies. This not only includes the carbon footprint during production but also the materials' recyclability and the ecological effects post-decommissioning. Therefore, focusing on sustainable materials and designs should be at the forefront of R&D efforts.
In addition, advancements in materials such as perovskites and organic photovoltaics offer fresh avenues for enhanced efficiency. These materials are proving to be more efficient than traditional silicon-based options while being potentially less expensive. As research progresses, it’s crucial that policies evolve to support these innovations, creating an ecosystem that encourages growth and adoption.
The role of technology in data analysis and optimization cannot be overstated. Integrating artificial intelligence and machine learning offers the potential to streamline processes from design to production, enabling smarter decision-making that enhances overall efficiency. By harnessing real-time data, manufacturers can adapt and refine their methods in a way that wasn't possible before.
"Embracing the future of solar technology means not just keeping our eyes on the horizon, but also being ready to change course based on new information and innovations."
Finally, collaboration across disciplines—entrepreneurs, engineers, researchers, and policymakers—is vital. Each stakeholder has a unique perspective that can contribute to more comprehensive solutions. Workshops, conferences, and forums should be platforms for sharing insights and best practices, paving the way for partnerships that can drive the solar industry forward.
In summary, the path ahead for solar cell efficiency is both exciting and complex. Addressing the challenges while leveraging new technologies and materials holds the key to unlocking the full potential of solar energy. By focusing not only on efficiency but also on sustainability and collaboration, we can shape a brighter, more renewable future.