Exploring Energy Storage Mechanisms and Processes
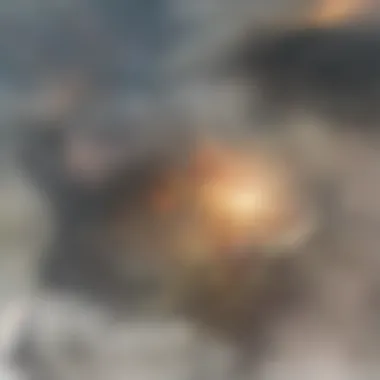
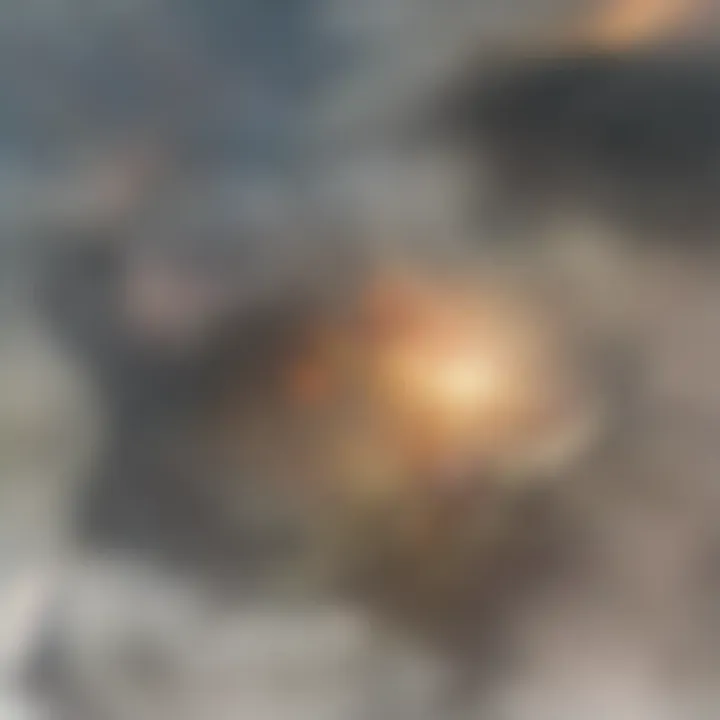
Intro
Energy storage is a fundamental aspect of both nature and technology. Understanding how energy is captured and retained can provide insights into biological processes, promote technological advancements and even inform sustainable practices. In this section, we will introduce the critical mechanisms involved in energy storage, setting the stage for a deeper exploration of diverse systems.
Background and Context
Overview of the Research Topic
Energy exists in various forms, including kinetic, thermal, chemical, and potential energy. The ability to store this energy allows for its utilization at a later time, which is essential for both living organisms and human-made systems. This article aims to dissect the mechanisms behind these storage processes, revealing how energy can be conserved and transformed.
Historical Significance
Historically, the understanding of energy storage began with the study of natural phenomena. For example, ancient civilizations harnessed solar energy long before formal study. The invention of the battery in the late 18th century by Alessandro Volta marked a significant leap in technological energy storage. Since then, advancements have continued, leading to modern innovations such as lithium-ion batteries and renewable energy storage systems.
Key Findings and Discussion
Major Results of the Study
The investigation of energy storage mechanisms can be grouped into biological, chemical, physical, and technological systems. Each method provides unique advantages and limitations influenced by the context in which they are used. Here are some key findings:
- Biological Systems: Organisms utilize processes like photosynthesis to convert solar energy into chemical energy stored in glucose. Other mechanisms include fat storage, where excess energy is stored in lipid molecules.
- Chemical Systems: Batteries and fuel cells are prime examples of chemical energy storage. They convert chemical energy into electrical energy when needed.
- Physical Systems: Capacitors store energy in an electric field but usually discharge quickly. Mechanical systems like flywheels also fall under this category, leveraging rotational energy.
- Technological Systems: Innovations such as grid-scale energy storage allow for the management of supply and demand in energy markets, crucial for integrating renewable energy sources.
Detailed Analysis of Findings
Each storage mechanism reveals underlying principles that govern energy retention. For biological systems, metabolic pathways illustrate how organisms efficiently harness and store energy.
In chemical systems, the relationship between energy density and efficiency becomes vital in choosing suitable storage technologies. The efficiency of energy systems impacts overall resource management and sustainability. Physical and technological systems often face challenges related to scale and infrastructure, which influences their practical applications.
"Understanding the mechanisms of energy storage is essential for advancements in science, technology, and sustainability."
The exploration of these themes prepares us to further analyze how energy storage systems operate, their practical applications, and future implications for society.
As we delve into biological and technological systems next, we will shed light on the intricacies that define how energy storage functions on both macro and micro levels.
Preamble to Energy Storage
Energy storage is fundamental in our modern world. It facilitates the capture and retention of energy for later use. This is increasingly important as we transition to a more sustainable future. With growing reliance on renewable energy sources, the need for effective energy storage systems becomes evident. These systems equalize supply and demand, ensuring that energy is available when needed. They help counteract the intermittent nature of renewable sources such as solar and wind.
Definition of Energy Storage
Energy storage refers to the various processes and technologies that allow energy to be preserved for future use. This can involve physical, chemical, or biological methods. Each method has its own principles and applications. At its core, energy storage addresses a basic need: to store excess energy produced at one time for consumption at another.
For instance, batteries convert electrical energy into stored chemical energy. When needed, this stored energy can be released back into the electrical system. Other forms include pumped hydro storage, which uses gravitational potential energy by moving water to a higher elevation, and thermal storage, which captures heat for later use. The variety of methods demonstrates the versatility and necessity of energy storage in maintaining energy balance across various applications.
Importance of Energy Storage Systems
Energy storage systems play a critical role in various sectors. Here are some key points outlining their importance:
- Integration of Renewables: They enable smoother integration of renewable energy sources into the grid. Without storage, surplus energy generated during peak production cannot be utilized.
- Grid Stability: Storage systems help maintain grid stability. When demand spikes, energy can be released quickly to stabilize the supply.
- Cost Efficiency: Over time, energy storage can lead to cost savings. By storing energy during low-demand periods, it can mitigate the higher costs associated with peak demand.
- Enhancing Reliability: Energy storage enhances the reliability of power supply, especially in regions prone to blackouts or natural disasters.
- Decarbonization Efforts: In the context of climate change, energy storage systems reduce reliance on fossil fuels, making renewable energy more practical.
"Energy storage is no longer just a luxury; it's a necessity for a sustainable energy future."
In summary, understanding energy storage systems is critical for grasping the challenges and opportunities presented by our shifting energy landscape. This knowledge is pivotal for students, researchers, educators, and professionals who seek to engage deeply with modern energy challenges.
Natural Energy Storage Mechanisms
Natural energy storage mechanisms play a vital role in the sustainability of ecosystems and the efficiency of energy retention systems. They provide insights into how energy is captured, stored, and utilized in various forms, significantly influencing both biological and geological processes. Understanding these mechanisms helps researchers develop innovative solutions to modern energy challenges by mimicking natural systems.
Biological Energy Storage
Photosynthesis as an Energy Storage Process
Photosynthesis serves as a crucial process where light energy is transformed into chemical energy stored as glucose. This transformation is fundamental for nearly all life forms on Earth, creating an energy foundation for ecosystems. The unique feature of photosynthesis is its ability to convert sunlight, a renewable source, directly into a usable form of energy, which is not only beneficial but essential for sustaining life.
Despite its advantages, photosynthesis is limited by environmental factors such as light intensity and temperature, which can affect its efficiency. However, its role as a primary energy storage mechanism lays the groundwork for other forms of energy use by living organisms.
Glycogen Fuel Reserves
Glycogen acts as a major energy storage polysaccharide in animals, primarily found in the liver and muscles. When the body requires energy, glycogen breaks down into glucose, providing a quick energy source for cellular functions. Its key characteristic lies in its rapid mobilization, which supports high-energy demanding activities like exercise.
One significant advantage of glycogen storage is its readily accessible energy supply. However, it is limited in quantity and can deplete quickly during intense physical activity. Additionally, excess intake of carbohydrates can lead to an increase in fat instead of glycogen, revealing a drawback in its storage mechanisms.
Fat Storage in Animal Cells
Fat storage in animal cells is primarily in the form of triglycerides, serving as a long-term energy reserve. This method of energy storage is efficient, as fats contain more than double the energy per gram compared to carbohydrates or proteins. The abundance of fatty acids allows animals to store excess energy effectively.
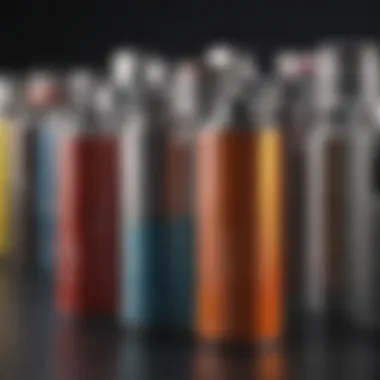
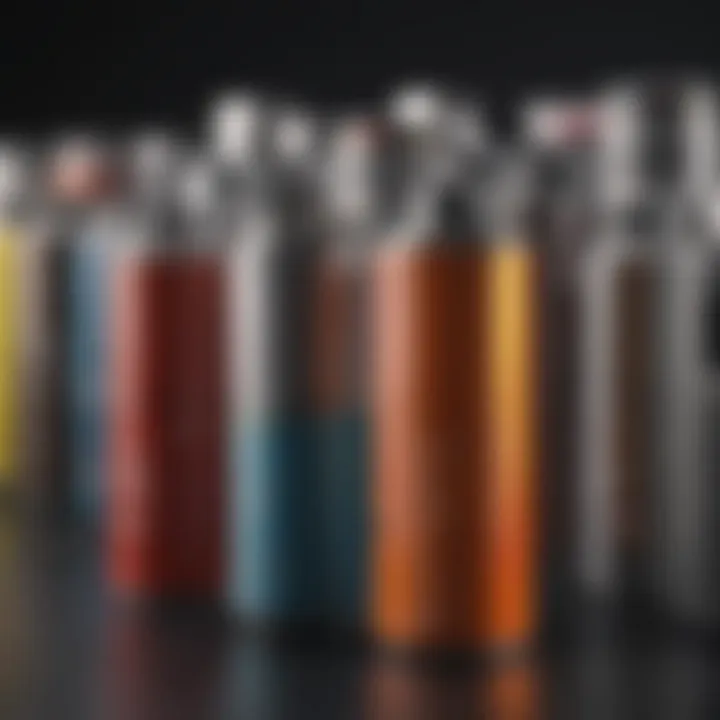
However, while fat serves as a valuable energy source during prolonged periods without food, its mobilization is slower compared to glycogen. This characteristic can be a disadvantage during immediate energy needs, showing that while fat is a beneficial storage mechanism, it comes with its own set of limitations.
Geological Energy Storage
Hydrocarbon Reservoirs
Hydrocarbon reservoirs are geological formations that store natural gas and oil. These reservoirs are crucial for meeting the world's energy demands. The key characteristic of hydrocarbon reservoirs lies in their ability to store large quantities of energy in a compact form, making extraction feasible.
However, reliance on hydrocarbon reservoirs carries environmental risks, such as pollution and greenhouse gas emissions. There's an ongoing concern about the sustainability of fossil fuels and a pressing need for cleaner energy alternatives. While beneficial in terms of energy output, the drawbacks of hydrocarbons raise significant questions about their future use in energy systems.
Geothermal Energy Storage
Geothermal energy storage leverages the Earth's internal heat for energy. This method is notably efficient, providing a stable energy source unaffected by weather or time of day. The key feature of geothermal energy is its low environmental impact since it generates minimal emissions.
One of the principal advantages of this type of storage is its reliability for baseload power. Nevertheless, geographical limitations can pose challenges to widespread implementation. Not every region possesses the necessary geothermal resources, which restricts its universal applicability.
Aquifer Thermal Energy Storage
Aquifer thermal energy storage utilizes underground aquifers to store thermal energy, either by heating or cooling water that is then retained for later use. It is an innovative solution for managing energy loads, especially in hot or cold climates. A significant characteristic of this method is its potential scalability, as many regions have accessible aquifers.
Advantages of aquifer storage include low operating costs and minimal environmental disruption. However, risks like groundwater contamination can arise, requiring careful management. Thus, while aquifer thermal energy storage presents many benefits, it also demands considerable oversight to prevent potential hazards.
Chemical Energy Storage
Chemical energy storage plays a crucial role in our society. It offers efficient methods to capture energy and hold it for later use. Different systems allow for a range of applications, from powering devices to supporting renewable energy sources. Understanding these mechanisms helps us realize their impact on our everyday lives and future technologies.
Batteries and Electrochemical Cells
Lead-Acid Batteries
Lead-acid batteries are one of the earliest forms of rechargeable batteries. They are widely used in various applications, particularly in automotive starters. Their key characteristic is the ability to deliver high surge currents, which is vital for starting engines. The technology also has advantages in its simplicity and cost-effectiveness.
However, lead-acid batteries have limitations. They are heavy and have a shorter lifespan compared to newer technologies. Furthermore, environmental concerns arise from lead pollution.
Lithium-Ion Technologies
Lithium-ion technologies have gained popularity for their superior energy density and lightweight. This characteristic makes them the preferred choice for portable electronics and electric vehicles. Their unique feature lies in the ability to charge quickly and offer longer cycle lives.
Nevertheless, lithium-ion batteries face challenges, including higher costs and safety concerns linked to thermal runaway. But ongoing research aims to address these issues, making them more reliable and efficient.
Emerging Battery Technologies
Emerging battery technologies, including solid-state and flow batteries, promise significant advancements. They aim to address limitations of conventional batteries by improving safety and extending energy storage capabilities. The key aspect here is innovation, with a focus on increasing energy density and reducing costs.
While these technologies bring new solutions, they are often still in experimental phases. Scaling them for commercial use presents challenges but also offers exciting potential features and advantages that could reshape how we store energy.
Fuel Cells
How Fuel Cells Work
Fuel cells operate through electrochemical processes that convert chemical energy directly into electricity. This method results in high efficiency compared to traditional combustion engines. The unique feature of fuel cells is that they use hydrogen and oxygen to produce electricity and water as a byproduct. This characteristic makes them an environmentally friendly option.
However, hydrogen production remains a hurdle, especially when produced from fossil fuels. Most fuel cells require pure hydrogen, which can limit widespread application depending on fuel supply.
Applications of Fuel Cells
The applications of fuel cells are diverse, ranging from transportation to stationary power generation. They provide clean energy solutions for vehicles and backup power for homes and businesses. Their key advantage is the ability to generate electricity on-demand without harmful emissions.
However, integrating fuel cells into existing systems can be costly. Infrastructure for hydrogen fuel is still developing. This presents challenges in terms of availability and accessibility.
Advantages of Fuel Cell Technology
Fuel cell technology holds several advantages. They are more efficient than internal combustion engines and can operate continuously when a fuel source is available. The unique aspect of fuel cells is their ability to provide a reliable power supply while reducing carbon footprints.
But the disadvantages include relatively high production costs and maintenance needs. Overall, despite challenges, fuel cell technology is a promising avenue for sustainable energy solutions.
Physical Energy Storage Solutions
Physical energy storage solutions play a crucial role in managing energy supply and demand. These systems provide a means to store energy in a form that can be utilized when demand exceeds supply. They can balance fluctuations in energy generation, particularly in renewable sources like wind and solar. By effectively harnessing stored energy, we can enhance grid stability, reduce operational costs, and increase energy efficiency.
Capacitance and Supercapacitors
Basic Principles of Capacitance
Capacitance refers to the ability of a system to store electric charge. This storage occurs when an electrical potential is applied across two conductive plates separated by an insulating material. A key characteristic of capacitance is that it allows for rapid charge and discharge, making capacitors ideal for applications where quick energy release is necessary. This ability renders capacitors a beneficial choice for various electronic circuits, including filtering and timing applications.
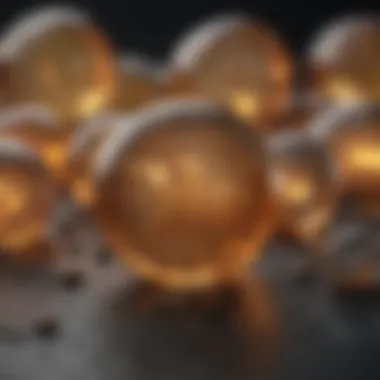
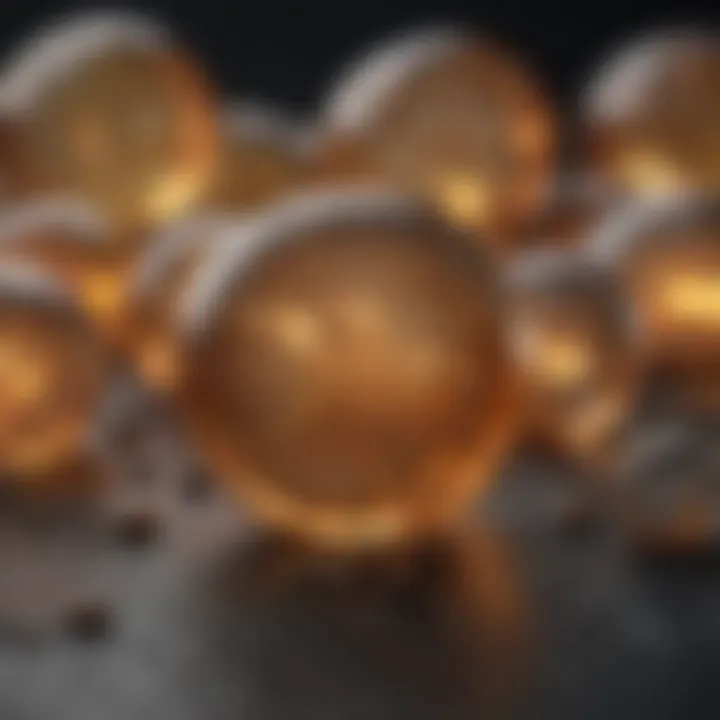
A unique feature of capacitance is the dielectric materials used, which contribute to the overall efficiency of energy storage. However, while capacitors can store energy quickly, they generally have a lower energy density compared to other storage methods, limiting their ability to provide sustained power.
Supercapacitor Advantages and Limitations
Supercapacitors, or ultracapacitors, are advanced capacitive devices that combine characteristics of both electrochemical capacitors and traditional batteries. One significant advantage of supercapacitors is their ability to charge and discharge rapidly, providing a high power output suitable for applications requiring quick bursts of energy.
However, supercapacitors have limitations, primarily in energy density. Despite being superior in charging speed, supercapacitors do not hold as much energy per unit mass compared to batteries. This characteristic influences the applications they are best suited for, particularly in hybrid systems where their rapid response can complement slower charging batteries.
Mechanical Energy Storage
Pumped Hydro Storage
Pumped hydro storage is a well-established technology leveraging gravitational potential energy. It involves pumping water to a higher elevation during periods of low demand. When energy is required, the stored water is released to flow back down through turbines, generating electricity. A key characteristic of pumped hydro storage is its ability to provide large-scale energy storage solutions efficiently, making it a popular choice for balancing grid supply and demand.
The unique feature of this system is its considerable capacity to store bulk energy. The disadvantages include the need for suitable geographical features and potential environmental impacts on local ecosystems.
Flywheel Energy Storage
Flywheel energy storage systems store energy in the form of rotational kinetic energy. When energy is supplied, the flywheel accelerates, storing energy in its mass's movement. This system stands out due to its fast response time and durability, offering a long cycle life compared to batteries.
Despite the many advantages, flywheel systems can be limited by mechanical losses and require a high-speed rotational component that needs careful design to prevent failure.
Gravity-Based Energy Storage
Gravity-based energy storage works similarly to pumped hydro but often utilizes solid weights. Energy is stored by lifting these weights, and released when they descend, activating generators. A major advantage is the simplicity of the technology, which can be implemented in diverse environments. Furthermore, it can be designed to function on a smaller scale compared to traditional pumped hydro systems.
The challenge lies in the system's efficiency and the material limitations for safely lifting and lowering heavy weights. Nonetheless, it represents an innovative approach towards physical energy storage, demonstrating potential as a viable solution in an energy-conscious world.
"Understanding physical energy storage mechanisms is essential for sustainable energy management, enabling us to seamlessly integrate diverse energy supplies into the grid."
In summary, physical energy storage solutions, including capacitance devices and mechanical systems, present various methods for capturing and utilizing energy effectively. Identifying their advantages and limitations helps inform their applications and optimize energy management strategies.
Thermal Energy Storage
Thermal energy storage plays a crucial role in energy management by allowing energy to be stored in the form of heat for later use. This technology is especially vital in incorporating renewable energy sources like solar and wind into the energy grid. Using thermal storage not only enhances the stability of energy systems but also reduces waste and increases efficiency in energy consumption.
Phase Change Materials
Mechanism of Phase Change
Phase change materials (PCMs) are substances that absorb or release heat during phase transitions, for example, from solid to liquid and vice versa. As they change phase, these materials can store or release large amounts of energy. This characteristic makes them highly effective for thermal energy storage. PCMs can help manage temperature fluctuations in buildings and industrial processes. Their ability to maintain consistent temperatures can also lead to energy savings in heating and cooling systems.
The unique feature of PCMs is their high latent heat capacity, allowing them to store more energy without needing significant space. The main advantage is their efficiency in storing energy, but they may have disadvantages like limited thermal conductivity or degradation over time.
Applications of Phase Change Materials
Phase change materials see diverse applications across multiple sectors. They are widely used in building materials, such as walls, ceilings, and floors, to enhance energy efficiency. Furthermore, they find use in thermal packs for temperature-sensitive goods during transportation.
A key characteristic of using PCMs is their capability to stabilize indoor temperatures in residential and commercial buildings. While they are effective, challenges include their initial costs and the need for precise integration into systems to maximize benefits.
Sensible Heat Storage
Methods of Sensible Heat Storage
Sensible heat storage relies on raising the temperature of a substance to store energy. This method is straightforward and efficient, using materials like water, rock, or even sand to absorb heat. When the stored heat is needed, the temperature of the substance is decreased, releasing the energy back into the environment.
The benefits of this approach are its simplicity and low cost, as many materials used are readily available. However, there are limitations related to the volume required for significant energy storage, as a large mass of material may be necessary to achieve meaningful heat retention.
Economic Considerations
Economic factors play a critical role in assessing sensible heat storage systems. Initial investment costs, operational costs, and maintenance expenses can vary significantly, impacting overall feasibility.
The main advantage of sensible heat storage is its comparatively low-cost construction and operation. However, the effectiveness of the system is significantly influenced by the local energy prices and the potential return on investment from energy savings. Moreover, large-scale installations could require substantial capital investments, making a detailed economic analysis essential before implementation.
Emerging Technologies in Energy Storage
Emerging technologies in energy storage are instrumental in redefining how we capture and utilize energy. These advancements offer improved efficiency, sustainability, and adaptability for various applications. Traditional energy storage systems often face limitations such as energy density, cost, and cycle life. Thus, exploring innovative solutions becomes essential, especially for integrating renewable energy sources.
Solid-State Batteries
Benefits of Solid-State Technology
Solid-state batteries present a significant advancement over conventional lithium-ion counterparts. Their primary benefit lies in enhanced safety. Unlike liquid electrolyte batteries, solid-state designs minimize risks related to leakage and combustion. They are also capable of greater energy density, which means they can store more energy in a smaller space. This characteristic is pivotal for sectors requiring compact energy storage, such as electric vehicles and portable electronics.
Moreover, solid-state batteries exhibit longer cycle life. Their components are less prone to wear over time, leading to improved longevity. With lower degradation rates, users can expect more reliable performance without frequent replacements. This aspect aligns well with the growing demand for sustainable energy solutions.
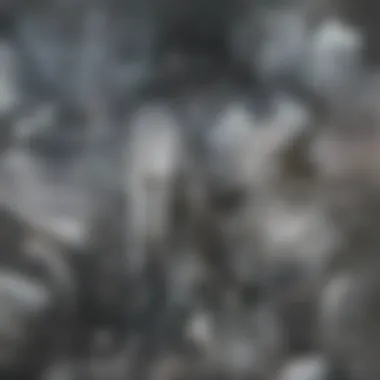
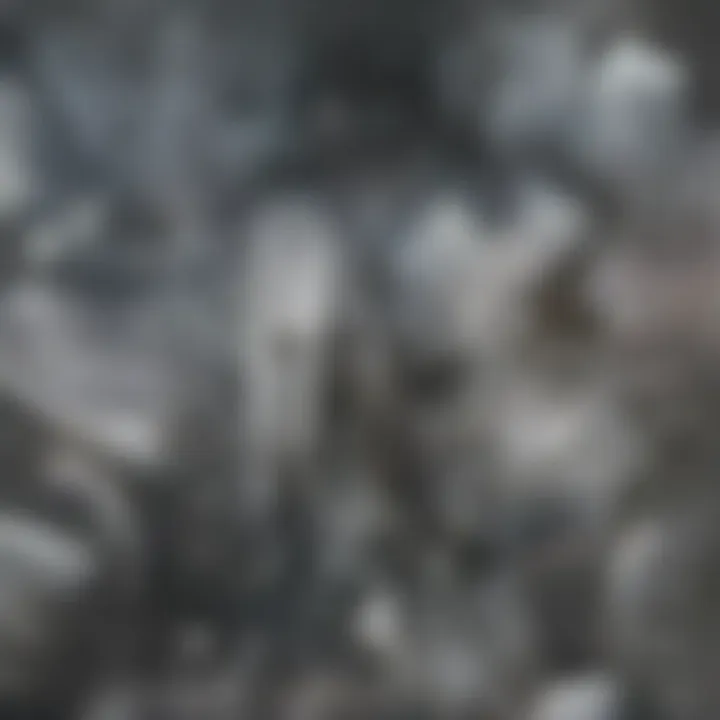
Current Research Trends
Current research trends focus on developing materials and optimizing battery architecture for solid-state systems. New electrolyte materials such as sulfide and ceramic conductors show promise in enhancing ionic conductivity. This development is vital for improving charge-discharge rates, which is a critical factor in energy storage performance.
Additionally, research aims to scale up production techniques. Innovations in manufacturing processes could lower costs, making solid-state batteries more accessible to consumers. The adoption of solid-state technology is particularly beneficial for the renewable energy sector, where efficient energy storage is crucial. However, challenges remain in achieving a balance between performance and cost-effectiveness.
Nanotechnology in Energy Storage
Nanomaterials for Energy Applications
Nanotechnology plays a transformative role in energy storage by allowing engineers to design materials at the nanoscale. This method can significantly enhance the performance of batteries and supercapacitors. A key characteristic of nanomaterials is their increased surface area, which improves charge storage capacity and speeds up the transport of ions. Consequently, they can deliver faster charging times and higher energy outputs.
The flexibility of nanomaterials also aids in creating lightweight devices, which is especially advantageous in automotive and portable electronic applications. These attributes make nanomaterials a popular choice in ongoing energy storage advancements. However, the relationship between cost and performance continues to be an area that requires careful consideration.
Potential Future Innovations
The potential future innovations stemming from nanotechnology applications in energy storage are compelling. Research continues to explore advanced nanocomposites that can offer even better performance metrics. Developing hybrids that combine different nanomaterials is a focal point, as this could lead to batteries that are both efficient and stable under varied conditions.
Furthermore, sustainability concerns are prompting exploration into biodegradable nanomaterials. This shift could address environmental issues associated with conventional energy storage technologies. Innovations like these can invite enthusiasm and scrutiny alike. The balance of performance, sustainability, and economic viability will dictate the success of these breakthroughs.
"The future of energy storage technology is intertwined with advancements in materials science and nanotechnology."
Integrating such emerging technologies into everyday applications is essential. These innovations are not merely enhancements; they signify a transition towards a more efficient and sustainable energy future.
Societal Implications of Energy Storage
Energy storage is an essential component of modern society, playing a significant role in addressing global challenges. The mechanisms and processes involved in energy storage not only provide the ability to capture energy for future use but also contribute to broader societal goals. This section discusses the implications of energy storage for climate change and the future of energy technologies. It emphasizes the necessity of integrating energy systems with environmental policies and economic considerations to achieve sustainability.
Energy Storage and Climate Change
Climate change represents one of the most pressing issues of our time. Energy storage is pivotal in supporting the transition toward renewable energy sources.
Role in Renewable Energy Integration
Renewable energy sources like solar and wind are intermittent; they do not produce energy continuously. Energy storage systems fill this gap by capturing surplus energy generated during peak production times and releasing it when demand increases. A key characteristic of this integration is the ability to stabilize the power grid. Effective energy storage can mitigate fluctuations in supply and demand. This is beneficial for maintaining a reliable energy source in various sectors, from residential to industrial.
- Unique Features: Energy storage systems enable a smoother transition to renewables by allowing energy to be stored and used when needed, increasing the overall efficiency of energy systems.
- Advantages: This adaptability to changing energy outputs is crucial for encouraging investments in renewable technologies.
- Disadvantages: However, the initial costs of these storage systems can be high, which may hinder their widespread adoption.
Mitigating Greenhouse Gas Emissions
The relationship between energy storage and greenhouse gas emissions is substantial. By facilitating greater use of low-emission and renewable energy sources, energy storage systems can help reduce reliance on fossil fuels.
- Key Characteristic: The integration of effective energy storage can lead to significant decreases in carbon emissions, which is increasingly recognized as essential in combating climate change.
- Unique Features: These systems provide the capability to transition energy sources, reducing the overall carbon footprint of energy production and consumption.
- Advantages: As nations strive to meet climate goals, energy storage systems will likely play an essential role in aligning energy strategies with emission targets.
- Disadvantages: Nonetheless, the environmental impact of the materials used in energy storage technologies also needs consideration, as they can contribute to the overall environmental footprint if not managed responsibly.
The Future of Energy Storage Technologies
As the world changes, so does the technology behind energy storage. Looking ahead, there exist clear trends that are shaping the future of energy storage technologies.
Trends in Global Energy Policy
Governments across the globe are adopting new policies aimed at promoting energy storage as part of their energy transition strategies. This trend is characterized by mandates for renewable energy integration and support for innovative technologies.
- Key Characteristic: Policies are often designed to incentivize investments in energy storage technologies, recognizing their role in achieving national and international energy goals.
- Unique Features: Such policies not only aim to enhance energy security but also to stimulate economic growth through job creation in new energy sectors.
- Advantages: There is a growing recognition of energy storage as a significant contributor to energy resilience.
- Disadvantages: However, policies need careful drafting to balance technological innovation with economic viability, which may be challenging for some regions.
Economic Viability of New Technologies
The economic aspects are another critical consideration that influences the future of energy storage.
- Key Characteristic: As technologies advance and production processes improve, the costs associated with energy storage are expected to decrease, making these systems more accessible.
- Unique Features: The viability of new technologies can promote energy independence and reduce energy bills for consumers.
- Advantages: This economic aspect can drive increased research and development investment in energy storage solutions.
- Disadvantages: Nonetheless, disparities in technological access exist, particularly in developing regions, which could widen inequalities in energy access and economic growth.
Culmination
The conclusion is a crucial segment of this article that encapsulates the essence of energy storage mechanisms. Reflecting on the various systems discussed throughout the document, it is important to understand how they interconnect and the relevance they hold in todayβs society. Energy storage is not merely a scientific endeavor; it is a practice that influences economic, environmental, and social paradigms.
Summary of Key Points
In this article, several essential themes and key points emerged:
- Diversity of Energy Storage: Energy storage encompasses a range of systems from biological methods like photosynthesis to technological developments such as lithium-ion batteries.
- Impact on Climate: The mechanisms of energy storage play a direct role in addressing climate change, enabling the integration of renewable energy sources into existing power structures.
- Technological Advancements: Innovations, particularly in the realm of solid-state batteries and nanotechnology, are enhancing the efficiency of energy storage systems.
- Economic Considerations: The viability of these technologies is heavily influenced by global energy policies and market demands, shaping investment and future development.
These points underline the multifaceted nature of energy storage and its critical role in modern society.
Future Perspectives on Energy Storage
Looking forward, the evolution of energy storage technologies presents numerous possibilities and considerations. Here are some future perspectives:
- Integration with Smart Grids: The advancement of smart grids will enable more efficient energy management using several storage methods. This integration promises improved reliability and optimization of energy resources.
- Research and Innovation: Ongoing research into alternative materials and processes, like solid-state batteries, will likely lead to breakthrough discoveries. The potential reduction in costs and improvements in safety are key focuses in this domain.
- Global Adoption: As awareness of climate issues and energy independence grows, more nations will turn to innovative energy storage solutions. Policies that favor renewable energy adoption will also enhance the growth trajectory of storage technologies.
Understanding these future trends is vital for stakeholders in energy sectors. Companies, governments, and researchers alike must adapt to this evolving landscape to effectively harness the benefits of energy storage solutions.
"The future of energy storage is not just about limiting emissions but also about creating a more resilient and efficient energy framework for all.'"