CRISPR-Cas9 and Sigma: Advancements in Gene Editing
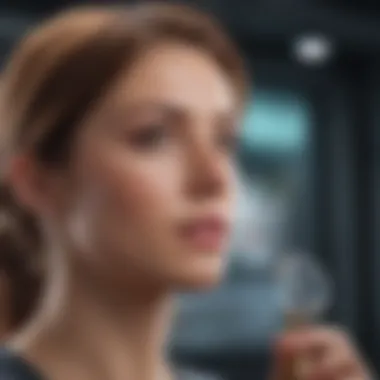
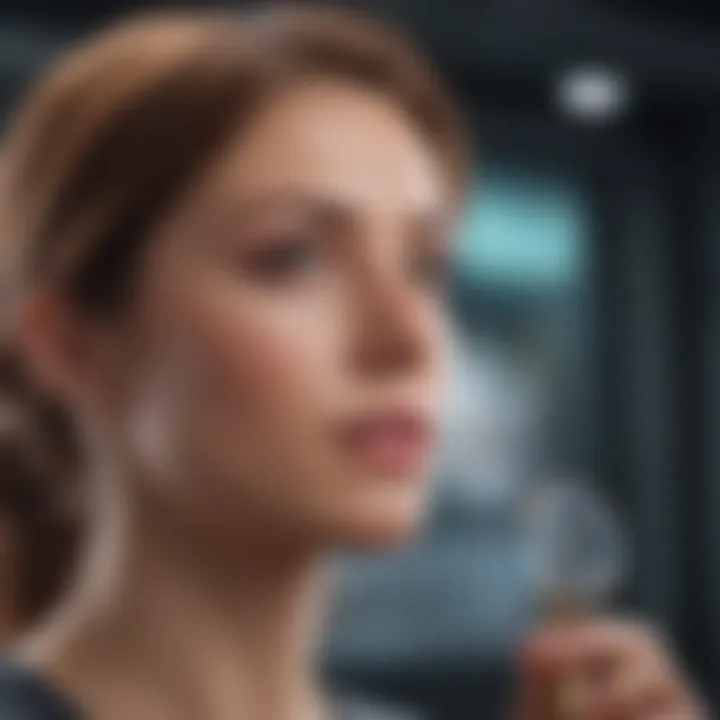
Intro
The landscape of genetic engineering has undergone a seismic shift with the introduction of powerful tools like CRISPR-Cas9 and sigma factors. Engineers of the biological world, these technologies open up new avenues for altering the very essence of life. CRISPR-Cas9, in particular, has been a game changer since its introduction, allowing targeted gene editing with exceptional accuracy. Sigma factors, often less talked about, play a vital role in how gene expression gets regulated, proving their relevance in the broader genetic modification discourse.
In this exploration, we aim to unpack the mechanisms, significance, and implications of CRISPR-Cas9 and sigma factors. The article will delve into the historical context of these innovative technologies, their applications across various scientific fields, and the ethical considerations that arise from their use. By stitching together these threads, we provide a comprehensive tapestry of how modern genetics is reshaping the future of biotechnology, medicine, and agriculture.
Prelude to CRISPR-Cas9
As we step into the realm of genetic engineering, it’s impossible to overlook the magnanimous impact of CRISPR-Cas9 technology. This innovation has pushed the envelope of what we previously thought possible in the world of molecular biology. With its ability to make precise cuts in DNA, it has transformed research, clinical applications, and even agriculture. The importance of understanding CRISPR-Cas9 goes beyond mere academic interest; it’s about grasping a paradigm shift that carries profound consequences for humanity.
Defining CRISPR Technology
At its core, CRISPR-Cas9 is a revolutionary gene-editing tool. The term CRISPR stands for Clustered Regularly Interspaced Short Palindromic Repeats, a snapshot of bacterial defense mechanisms. When paired with Cas9, which is a nuclease enzyme, CRISPR offers a way to cut DNA at targeted locations. This dual-entity functions remarkably similar to a pair of molecular scissors, slicing through the DNA sequence with exceptional precision. The significance of this technology lies not just in the act of cutting but in what happens next—the ability for researchers to add, remove, or alter segments of DNA. This presents countless possibilities ranging from correcting genetic disorders to enhancing agricultural traits in crops.
Historical Development of CRISPR
The historical journey of CRISPR is a testament to how curiosity fuels scientific discovery. CRISPR technology, as we know it today, had its genesis in the early 2000s, when scientists pieced together the intricacies of bacterial immune responses. What started as a way to understand how bacteria fend off viral intruders evolved into a tool for gene editing by 2012, when Jennifer Doudna and Emmanuelle Charpentier published their landmark paper. This was the tipping point that heralded a new era in genetics.
The functionality and simplicity of CRISPR-Cas9 allow it to be implemented across a multitude of species. By enabling targeted genetic modifications, it has become an invaluable asset in laboratories worldwide. Researchers have subsequently harnessed this technology to tackle issues ranging from sustainable agricultural solutions to innovative medical treatments. As we move forward, grasping the foundations of CRISPR-Cas9 sets the stage for deeper discussions about its mechanisms, applications, and the ethical considerations that accompany such power.
“The future of biotechnology will be remarkably shaped by how we navigate the possibilities of CRISPR-Cas9; it could be the key to sustainable solutions in medicine and agriculture.”
Mechanism of CRISPR-Cas9
Understanding the mechanism of CRISPR-Cas9 is pivotal in fully grasping its impact on gene editing and molecular biology. This technology operates with precision, allowing scientists to make specific alterations to DNA sequences, thus enabling ambitious research endeavors ranging from disease therapy to agricultural enhancements. The section delves into the integral elements that underpin the CRISPR-Cas9 system, identifying its key components and detailing how these elements collectively execute the gene-editing process. Moreover, it highlights the benefits these mechanisms carry, such as versatility and efficiency, as well as the considerations researchers must keep in mind to leverage them effectively.
Key Components of CRISPR-Cas9
The functionality of CRISPR-Cas9 is deeply rooted in its constituent parts, essentially acting as the toolkit for gene editing. Here we discuss two fundamental components: Cas9 nuclease and guide RNA.
Cas9 Nuclease
Cas9 nuclease is the muscles behind the operation of CRISPR-Cas9. This enzyme is responsible for making cuts in the DNA at specific locations. One key characteristic that sets Cas9 apart is its dual functionality; not only does it induce breaks in the DNA strands, but it also possesses a level of specificity that many other tools do not. Such precision significantly reduces unwanted off-target effects, thereby rendering it a popular choice for gene-editing applications.
A notable feature of Cas9 is its programmable nature, which is critical in customizing its target site through the guide RNA. While this offers immense potential for tailoring treatments or enhancements, researchers need to be cautious, as incorrect targeting could lead to adverse effects or unintended mutations in an organism's genome.
Guide RNA
In the CRISPR-Cas9 framework, the guide RNA serves as the GPS, directing Cas9 to the appropriate genomic location. An essential aspect of the guide RNA is its ability to be designed to complement any desired DNA sequence, allowing scientists to target specific genes with an incredible degree of accuracy. Its versatility is what makes it such a valuable tool in the editing arsenal; researchers can easily switch out one guide sequence for another to adapt to their needs quickly.
However, there are considerations that come with this power. For instance, the stability of guide RNA can affect the efficiency of gene editing. If the guide RNA degrades too quickly or does not bind effectively, the whole process can falter. Hence, understanding the dynamics of guide RNA with respect to Cas9 amounts to a balancing act where optimization is key.
The DNA Cutting Process
Once the guide RNA directs Cas9 to its target location, the cutting process kicks off. This step is crucial, as it marks the beginning of any subsequent repairs and edits to the genome. The Cas9 enzyme creates a double-strand break in the DNA, a critical intervention that opens the door for changes to be made in the genetic material.
This cutting activity is not only defined by where it occurs, but also by how efficiently it happens. The precision of the cut and the timing plays a significant role in ensuring that any modifications made will have the intended effects. The reality is that if the cut is made inaccurately, it can lead to not only faulty modifications but also may produce harmful consequences.
Repair Mechanisms Post-Cleavage
After the DNA has been cleaved, the cell's natural repair mechanisms come into play, which are vital to the successful implementation of CRISPR-Cas9 as a gene editing strategy.
Non-Homologous End Joining
One of the primary pathways for repairing double-strand breaks is known as Non-Homologous End Joining (NHEJ). This mechanism acts as a quick fix, where the two ends of the DNA break are joined together without the need for a template. This feature makes it a fast, convenient option for cells. However, the downside is that NHEJ can be error-prone, leading to insertions or deletions that can disrupt gene function.
Key characteristics of NHEJ:
- Rapid action - It quickly repairs breaks without requiring homologous sequences.
- Potential for mutations - Errors may arise, introducing unwanted alterations into the genome.
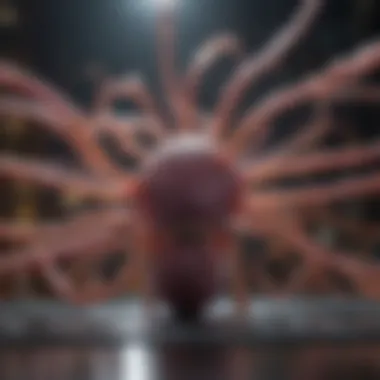
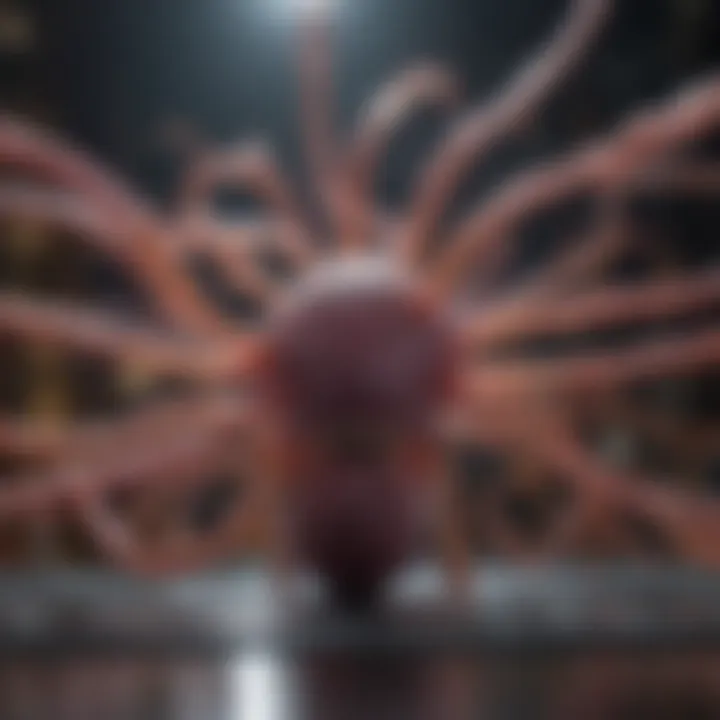
Homing Repair
In contrast to NHEJ, homing repair operates via a more intricate template-dependent process. This approach requires a homologous sequence as a guide to ensure accurate repair. This precision positions homing repair as a desirable option for corrections that demand last-minute finesse.
However, the necessity for a homologous template adds a layer of complexity; finding a suitable template can prolong the editing process and limit its applicability in certain situations.
This interplay between rapid and precise repairs creates a dynamic environment within the CRISPR-Cas9 ecosystem, illustrating the importance of understanding which repair mechanism to utilize based on the intended outcome.
"The intricate dance between Cas9’s precise cutting and the following DNA repair mechanisms determines the success of gene editing endeavors, influencing both experimental results and therapeutic applications."
Applications of CRISPR-Cas9
The significance of CRISPR-Cas9 in the arena of gene editing cannot be understated, as it paves the way for innovative medical, agricultural, and biotechnological advancements. Its capacity to modify genes with pinpoint accuracy not only turns longstanding theories into remarkable realities but also raises profound questions about ethics and the boundaries of scientific inquiry. Let’s delve into the varied applications of CRISPR-Cas9, highlighting specific elements that make it a game-changer.
Biotechnology Advancements
Gene Therapy
Gene therapy stands at the pinnacle of CRISPR applications, showcasing its ability to tackle genetic diseases at their root. Unlike traditional treatments that merely address symptoms, gene therapy is geared toward correcting the faulty genes causing the illness. A standout feature of this approach is its specificity—CRISPR can directly target and edit the problematic sections of DNA.
One unique aspect of gene therapy using the CRISPR system is its adaptability; for instance, different delivery mechanisms, such as viral vectors or lipid nanoparticles, can be employed depending on the specific condition being treated. This flexibly lends itself to the precision needed in clinical settings and expands the range of treatable disorders, from sickle cell disease to muscular dystrophy.
However, it's not without its challenges. Ethical concerns loom large regarding potential off-target effects and long-term consequences of genetic modifications, which creates a balancing act between innovation and caution.
Pharmaceutical Developments
Pharmaceutical developments have seen a radical shift with the integration of CRISPR technology, which allows for targeted drug discovery and the development of personalized treatments. The standout characteristic here is the efficiency with which researchers can glean insights into drug interactions and gene functionalities. With CRISPR, scientists can generate disease models faster and cheaper than ever, hence, speeding up the entire drug development pipeline.
A notable feature within this domain is also the capability for high-throughput screening— researchers can test thousands of compounds in a matter of days. This rapid-fire approach not only helps identify promising drug candidates earlier but also narrows down possibilities by determining which genes are essential for a given condition.
But there are drawbacks to consider, such as the complexity of regulatory paths for new drugs and the potential for unintended genetic consequences, demanding rigorous testing before any treatments reach the market.
Agricultural Innovations
Crops with Enhanced Traits
In the realm of agriculture, CRISPR offers groundbreaking solutions through the development of crops with enhanced traits. This method allows for precisely editing plant genes to promote beneficial characteristics—like drought resistance or increased yield capacity. This tailored approach stands in contrast to traditional breeding methods, which can be time-consuming and less predictable.
A unique aspect of enhanced traits is how quickly they can be generated. Rather than waiting a generation for natural mutations to occur, scientists can introduce favorable traits in a matter of months. This not only accelerates crop improvement but also addresses food security issues effectively.
Yet, the potential for genetically modified organisms (GMOs) to face public scrutiny poses challenges. While these benefits shine brightly, they come alongside debates about environmental impacts and biodiversity.
Plant Resistance to Diseases
Plant resistance to diseases is another profound application of CRISPR technology, with an emphasis on creating varieties that can withstand pathogens. By editing genes responsible for plant health, CRISPR can enhance the resilience of crops against pests and viral infections.
The standout characteristic of this application lies in the dual advantage of boosting productivity while reducing the need for chemical pesticides. This means healthier ecosystems and potentially lower farming costs for growers. The unique feature of such plants is that they can be improved without introducing foreign DNA, making the regulatory process more straightforward in some regions.
However, challenges persist, including the unpredictability of how these edited genes interact with the wider agricultural environment, which necessitates ongoing research and dialogue within farming communities.
Impacts on Medicine
The intersection of CRISPR technology and medicine is profoundly transformative. Its capacity to facilitate personalized medicine changes how diseases are understood and treated. With the ability to target specific genes, CRISPR offers up new possibilities for addressing previously untreatable conditions, which may reshape the healthcare landscape.
In summary, the applications of CRISPR-Cas9 spread across biotechnology, agriculture, and medicine, revealing its multifaceted nature. Each sector grapples with both the promise and the ethical implications of gene editing, urging continuous reflection and dialogue as we move forward into this uncharted territory.
"In a world of rapid technological advancement, the careful navigation of innovation and ethics will be paramount."
By dissecting each of these applications, we gain clarity on not only the capabilities of CRISPR-Cas9 but also the responsibilities that accompany such powerful tools.
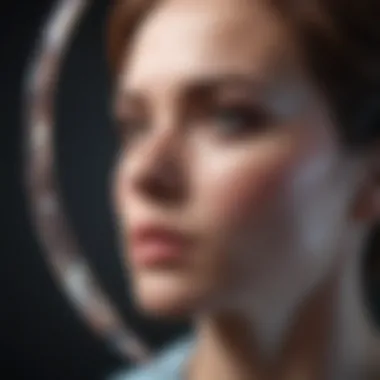
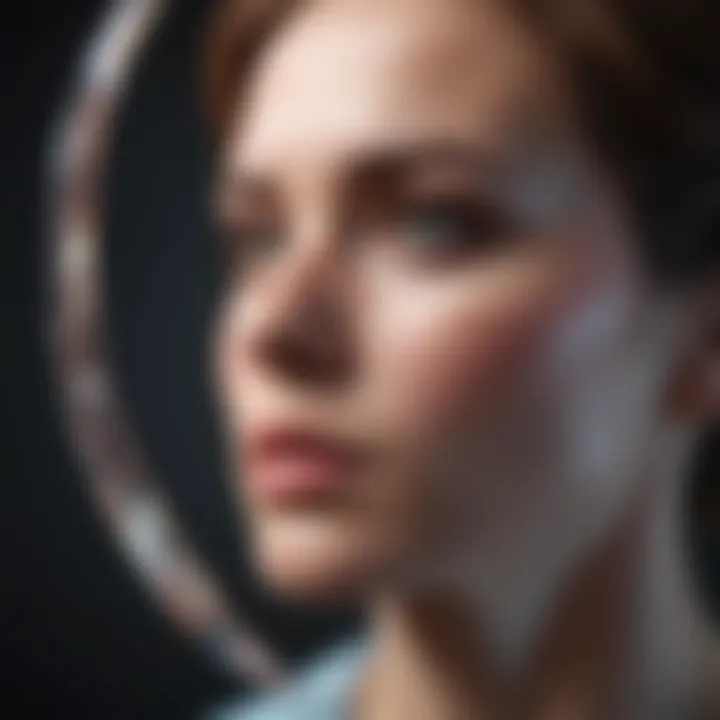
Prelims to Sigma Factors
In the intricate field of genetics, sigma factors hold significant importance, primarily because they orchestrate the process of transcription in bacteria. They are essential proteins that guide RNA polymerase to specific promoter regions on the DNA, influencing which genes are expressed at any given time. Understanding sigma factors thus offers valuable insights which complement our grasp of CRISPR technology.
Recently, the exploration of sigma factors in relation to CRISPR-Cas9 has emerged as a key area of study, shedding light on their joint potential in gene editing. With CRISPR revolutionizing the ability to precisely cut and modify genes, the regulation of those modifications is equally critical. Thanks to sigma factors, researchers can fine-tune gene expression, thereby amplifying or diminishing the effects of CRISPR interventions.
This section delves into the essential roles that sigma factors play in gene regulation and classifies the various types that exist. By elucidating the mechanisms behind transcription regulation and the diverse sigma factors available, we allow for a deeper understanding of how these elements integrate with CRISPR technology to enhance genetic research and its applications.
Role of Sigma Factors in Gene Regulation
Sigma factors are pivotal in controlling gene expression in prokaryotes. They act as subunits that attach to RNA polymerase, enabling this enzyme to recognize the right promoters and initiate transcription. During processes like stress responses and developmental changes, sigma factors provide flexibility and adaptability to bacteria by altering which genes are expressed in response to changing environments.
In their role, sigma factors can respond to various environmental signals, potentially leading to the activation of genes that might otherwise remain silent. By doing so, sigma factors help bacteria adapt swiftly, promoting survival in tough conditions. The regulatory roles of these factors contribute to a broader understanding of gene expression dynamics, which is crucial when manipulating genetic material using technologies like CRISPR-Cas9.
A few noteworthy applications of sigma factors include:
- Stress Response: Inducing specific gene expression in reaction to environmental stressors like heat or salt.
- Biofilm Formation: Regulating the genes responsible for creating biofilms, which are critical for survival in certain conditions.
- Virulence: Influencing the expression of virulence factors in pathogens, which can be a target for therapy.
"Ultimately, understanding sigma factors not only reveals how bacteria manage their genetic toolkit but also highlights strategies to harness this knowledge in gene editing technologies."
Types of Sigma Factors
Sigma factors are categorized into several types based on their function and the genes they regulate. Here’s a glance at a few prominent types:
- Primary Sigma Factors (σ70): The most common sigma factor in E. coli, essential for the transcription of housekeeping genes, it works under standard growth conditions.
- Alternative Sigma Factors: These are specialized and typically activated under stress or specific developmental stages. Each alternative sigma factor is responsible for regulating a unique set of genes. For example:
- Extracytoplasmic Function (ECF) Sigma Factors: These sigma factors play roles in responding to external stimuli, such as antibiotics or changes in osmotic pressure. They help manage genes that protect the bacterial cell from these challenges.
- Spore Sigma Factors: Found in specific bacteria such as Bacillus, these factors are involved in the development of spores, allowing bacteria to survive adverse conditions in a dormant state.
- σ32: Responds to heat shock, regulating heat shock proteins crucial for survival when facing elevated temperatures.
- σ54: Involved in nitrogen metabolism, enabling the bacteria to adapt their metabolic pathways when nitrogen sources fluctuate.
By comprehending the diverse types of sigma factors and their particular functions, researchers can leverage this knowledge to optimize gene editing techniques, enhancing CRISPR-Cas9 applications across various domains, including medicine and agriculture. Their interplay helps ensure that the genetic changes made through gene editing are appropriately expressed, harmonizing with the targeted goals of the modifications.
Interconnection of CRISPR-Cas9 and Sigma
Understanding the interrelationship between CRISPR-Cas9 technology and sigma factors serves as a fundamental piece in the puzzle of gene editing. Both elements play pivotal roles in genetic regulation and modification, but their connection goes deeper than surface-level applications. The convergence of these technologies offers a more nuanced approach to gene editing, empowering scientists with a toolbox that not only provides precision in gene manipulation but also insights into the cellular machinery underlying these processes.
How Sigma Factors Affect CRISPR Outcomes
At first glance, the role of sigma factors might seem distinct from the cutting-edge methods of CRISPR-Cas9. Yet, sigma factors, which are proteins essential to initiating transcription in bacteria, can significantly influence how effectively CRISPR-Cas9 functions. To put it plainly, when you have the right sigma factor present, the CRISPR machinery can recognize and bind to target DNA more efficiently, thus enhancing editing outcomes.
For instance, studies reveal that the presence of specific sigma factors can lead to higher levels of transcription of CRISPR components, improving the overall success rates of gene editing endeavors. When sigma factors direct RNA polymerases, they assist in producing more guide RNA, which is crucial for the Cas9 enzyme to locate its target within the genome. Furthermore, the interaction between these factors can inform the choice of guide RNA design, creating avenues for more tailored and refined CRISPR applications.
While the specifics of these interactions are still being explored, the implications are profound.
“The interplay of sigma factors with CRISPR-Cas9 is like a well-rehearsed dance - the more in sync the partners, the better the performance.”
Applications Combining Both Technologies
As the research landscape regarding CRISPR-Cas9 and sigma factors evolves, innovative applications are beginning to emerge that harness the strengths of both technologies. The following areas are notable for their transformative potential:
- Targeted Gene Therapy: Utilizing sigma factors to enhance the expression of CRISPR components can lead to more efficient gene editing treatments for genetic disorders. This combination allows for the selective targeting of mutated genes while preserving the integrity of the surrounding genetic material.
- Synthetic Biology: In synthetic biology, designing sophisticated genetic circuits could benefit from employing sigma factors alongside CRISPR. By controlling the expression of various genes through sigma factors, researchers can create more intricate genetic networks that respond predictably to environmental stimuli.
- Agricultural Biotechnology: In crops, employing sigma factors to boost the efficacy of CRISPR-edited genes can accelerate the development of traits such as drought resistance or enhanced nutrient uptake. This can lead to more robust agricultural solutions, crucial in addressing food security challenges.
The fusion of CRISPR-Cas9 with sigma factor technology isn't just a passing trend. It's an evolving paradigm that could redefine our approach to gene editing and manipulation in multiple disciplines. As both fields advance, the synergy of CRISPR and sigma factors stands to open new doors to innovations, ultimately reshaping our understanding of genetics.
Ethical Considerations
In an era where gene editing technologies like CRISPR-Cas9 are fast advancing, ethical considerations arise as a paramount concern. While the scientific community marvels at the potential benefits—ranging from eradicating genetic diseases to enhancing agricultural yield—we must not lose sight of the moral and societal implications these advancements carry.
Gene editing is not merely a technical breakthrough; it's a powerful intervention that could reshape life as we know it. Thus, it's essential to delve deep into the ethical landscape that this technology treads upon. Addressing the ethical elements of gene editing helps determine what constitutes responsible use and where lines should be drawn.
Concerns about Gene Editing
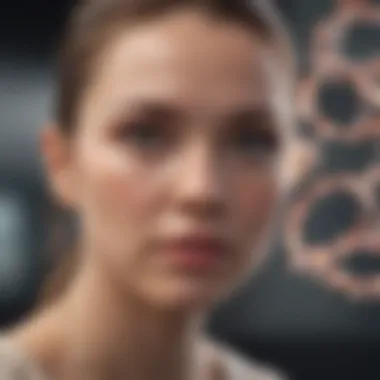
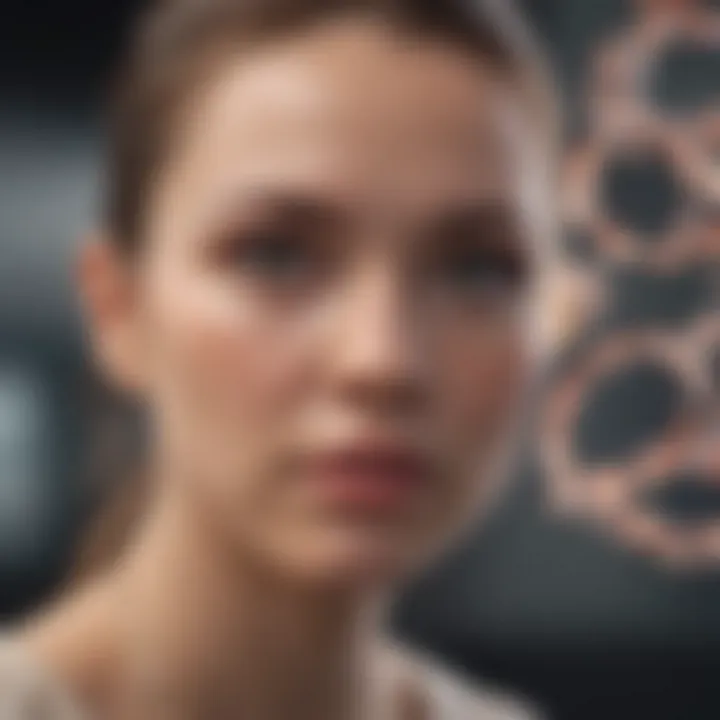
The discussion surrounding ethical concerns in gene editing is multifaceted and complex. Many worries rise to the surface:
- Safety Issues: One of the primary concerns pertains to off-target effects, where unintended alterations in the genome may lead to unforeseen health issues in organisms, including humans.
- Equity and Access: As this technology becomes more refined, there is a risk that only affluent individuals or countries may reap its benefits, widening health and economic disparities.
- Designer Organisms: The capability to not just correct genes but to select traits raises deep concerns about "designer babies". Many wonder where the line lies between correction and enhancement. Is it ethical to create individuals with artisanally selected traits?
- Biodiversity Risks: Modifying agricultural crops or animal species can lead to reduced genetic diversity. The long-term ecological impacts remain uncertain. How do we ensure that such interventions do not disrupt existing ecosystems?
Addressing these concerns isn’t about stifling innovation but promoting a cautious approach that weighs potential rewards against risks. Collectively, society needs to engage in meaningful discussions that integrate various perspectives, ensuring a balanced approach to gene editing.
Regulatory Frameworks Surrounding CRISPR
To navigate the minefield of ethical dilemmas, robust regulatory frameworks are vital. These structures can help steer gene editing practices toward responsible usage while enhancing public trust. Currently, various regulations are in place, though they differ significantly between countries.
The key aspects of these frameworks typically involve:
- Oversight Mechanisms: Governments or regulatory bodies implement strict oversight on research applications of CRISPR, scrutinizing proposed experiments carefully.
- Public Engagement: Engaging the public in discussions about gene editing creates awareness and allows for diverse opinions, guiding policy decisions.
- International Collaboration: Forming global alliances and treaties enhances communication about the potential consequences of gene editing, fostering shared ethical standards.
Additionally, organizations like the World Health Organization (WHO) have been active in setting guidelines, urging scientists and policymakers to ensure that ethical considerations remain at the forefront.
Following responsible gene editing practices ensures not only the safety of research subjects but also the integrity of scientific progress.
Future Directions in Gene Editing
The rapidly evolving landscape of genetic engineering is nothing short of groundbreaking. As we look ahead, it is crucial to understand the future directions in gene editing, specifically through the lens of CRISPR-Cas9 and associated technologies. The possibilities seem almost limitless, yet they come with their own complexities and nuances that researchers need to navigate. As innovations emerge, one must remain cognizant of both the practical benefits these technologies offer and the ethical ramifications they carry.
Novel Technologies on the Horizon
Emerging technologies are reshaping how we approach gene editing. The next generation of gene editing tools promises enhanced precision and efficiency, which will likely propel biological research forward. Technologies like prime editing—often dubbed as the 'next CRISPR'—allow for targeted changes without the risks associated with traditional methods.
- Prime Editing: This method uses a fusion of a Cas9-like protein and a reverse transcriptase to generate desired changes in DNA and could represent a significant stride towards error-free editing.
- CRISPR 2.0: Modifications to existing systems are also on the rise, like using Cas12 and Cas13 proteins that offer distinct advantages for specific applications, such as targeting RNA or promoting precise cuts in DNA.
By harnessing these advancements, researchers can tackle genetic disorders more effectively. Such breakthroughs in gene editing technology have the potential to pave the way for new gene therapies with higher success rates and reduced side effects.
Potential Transformations in Research Fields
The implications of advanced gene editing technologies stretch far and wide, influencing various research fields significantly. For instance, within agricultural science, the ability to edit multiple genes simultaneously can lead to the development of crops that are both drought-resistant and pest-resistant. This could ensure food security in regions facing climate change challenges.
In the realm of medicine, gene editing could revolutionize diagnostics and therapeutics for diseases once deemed untreatable. Imagine a world where conditions like cystic fibrosis or sickle cell anemia can be addressed at the molecular level. This perspective aligns with the growing trend of personalized medicine, where treatments are tailor-made based on an individual's genetic blueprint.
Moreover, the integration of bioinformatics with these technologies can enhance research methodologies. Tools that analyze genetic data rapidly and accurately will allow for quicker iterations and feedback loops in experimental designs. As we venture into this exciting future, it’s pivotal to consider regulatory frameworks and ethical considerations that accompany these innovations, ensuring they align with societal values and expectations. "While the technology propels us forward, an ethical compass must guide our momentum."
In summary, the future of gene editing through CRISPR-Cas9 and its evolving counterparts presents staggering possibilities. Understanding these advancements allows us to appreciate their implications in real-world contexts, albeit with a careful eye on ethical considerations—balancing the cutting-edge with responsibility.
Finale
In wrapping up our exploration of CRISPR-Cas9 and sigma factors, it’s vital to reflect on the key elements we’ve touched upon. This article underscores the transformative power of gene editing technologies, illustrating not only the mechanistic intricacies of CRISPR-Cas9 but also the pivotal role that sigma factors play in gene expression.
One of the major insights is the remarkable precision CRISPR technology offers in editing genetic material. Unlike older methods of gene editing, CRISPR-Cas9 enables researchers to target specific sequences with an accuracy that was previously unimaginable. This has unlocked numerous avenues in biotechnology, such as developing tailored therapies for genetic disorders and advancing agricultural practices to combat food scarcity.
“The implications of CRISPR-Cas9 technology extend far beyond mere scientific curiosity; they possess the potential to redefine medical practices and agricultural sustainability.”
Moreover, the integration of sigma factors adds a layer of complexity to our understanding of gene regulation. These factors determine how genes are expressed, impacting the outcomes of gene editing. The interaction between CRISPR consequences and sigma regulation provides a rich field of study for comprehension of genetic modifications. Consequently, scholars and practitioners must consider the broad spectrum of interactions within biological systems.
When examining the ethical implications, it becomes clear that the discourse surrounding gene editing is of immense import. The potential for unintended consequences or misuse requires a robust regulatory framework to ensure that innovations are implemented responsibly. The future of gene editing will largely depend on our ability to balance the scientific potential with societal considerations.
In summary, the possibilities that arise from CRISPR-Cas9 and sigma factors are exhilarating, yet they come with an array of responsibilities. As we move forward into this brave new world of genetic engineering, keeping a close eye on both the opportunities and challenges will be key. This will not only foster scientific advancements but also guide our ethical compass as we navigate uncharted territory in gene editing.
Summarizing Key Insights
The complex interplay between CRISPR-Cas9 and sigma factors unlocks many questions and possibilities. Here are some key insights we have examined:
- Precision of Gene Editing: CRISPR offers unprecedented accuracy in targeting genetic sequences, fundamentally altering the landscape of genetic engineering.
- Role of Sigma Factors: These proteins are crucial in regulating gene expression, affecting how CRISPR edits manifest in various organisms.
- Application Spectrum: From medicine to agriculture, the applications of these technologies are vast, presenting solutions to some of the world’s pressing challenges.
- Ethical Landscape: The implications of gene editing necessitate ongoing dialogue about safety, legality, and ethical considerations.
By understanding these components, researchers and stakeholders can work toward enhancing the benefits while mitigating potential hazards in gene editing.
Final Thoughts on Future Prospects
Looking ahead, the realm of gene editing stands on the brink of significant advancements. Future prospects in this area are bright for several reasons:
- Innovative Techniques: With ongoing research, we can expect the advent of novel gene editing tools that may surpass CRISPR's capabilities.
- Interdisciplinary Collaborations: Collaboration between biologists, engineers, ethicists, and policy-makers will likely yield more comprehensive insights into the implications of gene editing technology.
- Refinement of Ethical Guidelines: Enhanced focus on ethics will facilitate more equitable access and responsible use of gene editing technologies.
- Contextual Applications: The convergence of CRISPR and sigma factors may pave the way for tailored treatments specific to individual genetic profiles, ushering in a new era of personalized medicine.