CRISPR-Cas9 Protocol: A Comprehensive Guide
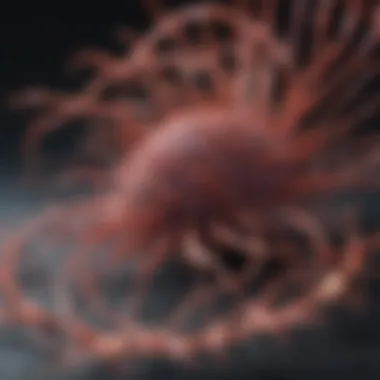
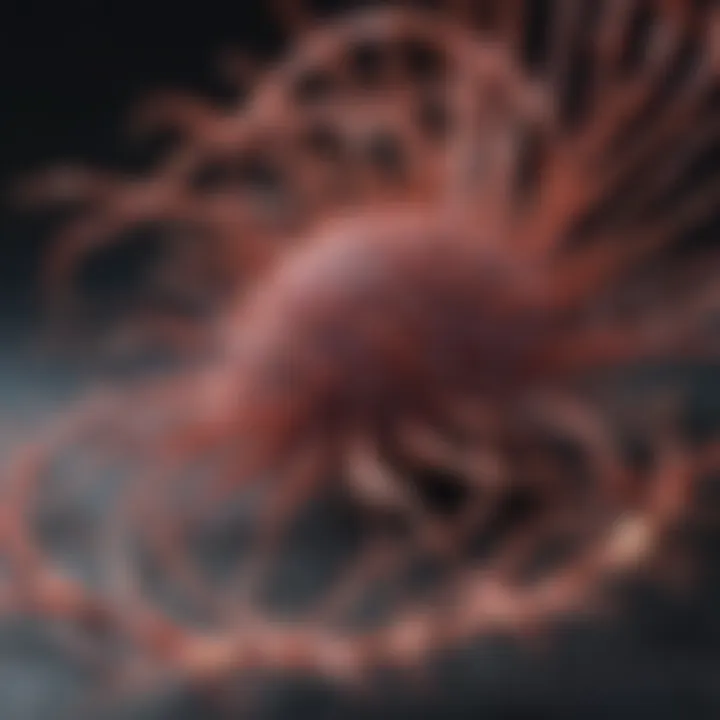
Intro
The rapid progression of genetic engineering has always sparked significant interest among scientists and researchers. At the forefront of these advancements is the CRISPR-Cas9 protocol, a groundbreaking technique that has changed the landscape of molecular biology. Understanding CRISPR-Cas9 is essential for anyone delving into genetic research. This guide serves to illuminate the complexities of this technique, providing comprehensive insights into its working mechanism, applications, and ethical implications.
Background and Context
Overview of the Research Topic
CRISPR-Cas9 stands for clustered regularly interspaced short palindromic repeats and CRISPR-associated protein 9. Originally discovered in bacteria, this system provides a form of immune response against viral threats. Scientists have adapted this mechanism for targeted gene editing. This innovative method is distinctly more precise, efficient, and cost-effective than earlier technologies, such as TALENs or zinc-finger nucleases.
Historical Significance
The journey of CRISPR-Cas9 began in the mid-1980s when researchers first identified its potential in prokaryotes. However, it wasn’t until 2012 that Jennifer Doudna and Emmanuelle Charpentier demonstrated its application in genome editing. This study ignited a revolution in genetics, enabling unprecedented control over the DNA of living organisms. The historical significance of the dual-function nature of this tool cannot be overstated. It has made complex genetic alterations straightforward, fostering developments in multiple fields including agriculture, medicine, and biotechnology.
Key Findings and Discussion
Major Results of the Study
The CRISPR-Cas9 protocol has led to a myriad of notable findings. Specifically, it has showcased:
- Precise Gene Editing: Researchers can alter specific genes with remarkable accuracy, which reduces the likelihood of off-target effects.
- Broad Application Scope: The versatility of CRISPR-Cas9 allows its use across various organisms, including plants, animals, and human cells.
- Cost Efficiency: Compared to other genetic editing methods, CRISPR-Cas9 significantly lowers the cost of experimentation and implementation.
Detailed Analysis of Findings
The components of CRISPR-Cas9 include the guide RNA and the Cas9 enzyme. The guide RNA directs Cas9 to the specific gene sequence to be modified. Once bound, Cas9 cuts the DNA, leading to either gene disruption or insertion of new genetic material. The simplicity of designing guide RNA has vastly democratized access to gene editing, allowing even smaller research labs to engage in groundbreaking projects.
Moreover, ethical considerations surrounding CRISPR-Cas9 are rapidly evolving. While the technology holds promise for combating genetic disorders and advancing agricultural resilience, it also raises questions about biodiversity, genetic privacy, and biosecurity. Addressing these concerns is crucial for the responsible use of CRISPR-Cas9 in future research.
"The power of CRISPR goes beyond gene editing; it is about shaping the future of genetic research and ethics combined."
In summary, the CRISPR-Cas9 protocol represents a monumental leap forward in genetic engineering. Its profound implications and potential applications underscore the importance of understanding both its mechanisms and its broader impact on society.
Foreword to CRISPR-Cas9
The CRISPR-Cas9 protocol represents a significant milestone in genetic engineering and molecular biology. Its rise has shifted paradigms in how scientists approach gene editing, making this technology crucial for a myriad of applications, from medical research to agricultural innovations. Understanding the principles and mechanisms behind CRISPR-Cas9 is essential for researchers and professionals, as it enables the exploration of genetic modifications with precision and efficiency.
This section will explore both the historical context of CRISPR technology and precisely define its core components, namely CRISPR and Cas9. By examining these elements, readers can grasp not only the innovative nature of the technology but also the underlying rationale for why CRISPR-Cas9 is a transformative tool in biological sciences.
Historical Context
The history of CRISPR technology is rooted in the study of bacterial immune systems. Initially, researchers uncovered that certain bacteria utilize a system for defending against viruses. This discovery led to insights regarding Clustered Regularly Interspaced Short Palindromic Repeats (CRISPR) and the associated proteins, particularly Cas9. The first significant use of this technology in gene editing was reported in 2012 by Jennifer Doudna and Emmanuelle Charpentier, marking a pivotal moment that sparked global interest and subsequent research.
Since then, the evolution of CRISPR-Cas9 has accelerated rapidly. It has allowed scientists to develop a method for precise gene editing with an unprecedented degree of efficiency. The ease of use, combined with the capacity for multiplexing—editing multiple genes at once—has propelled CRISPR-Cas9 to the forefront of genetic research. This allows for the broad application of genetic modifications across species, enhancing research capabilities and opening new avenues in science and medicine.
Defining CRISPR and Cas9
To understand CRISPR-Cas9, it is vital to clarify what its components are. CRISPR refers to a natural system that bacteria use to keep a record of viral invaders and mount immunity against them. In simple terms, it captures segments of DNA from viruses and stores them to recognize and cut these viruses efficiently if they invade again.
Cas9, on the other hand, is a protein that acts as molecular scissors. It cuts DNA at precise locations defined by the RNA guide. The combination of CRISPR sequences and the Cas9 protein allows for targeted editing in the genomes of various organisms, including plants and animals.
In summary, the significance of CRISPR-Cas9 stems not only from its ability to enhance research but also from its potential for practical applications. For instance, it holds promise for gene therapy, allowing for the correction of genetic disorders. The historical context provides a foundation that highlights the system’s scientific journey and evolution into a tool fundamental for today's biotechnological advancements.
Mechanism of Action
The mechanism of action of the CRISPR-Cas9 system is pivotal to understanding how this technology functions. It serves as the foundation for genome editing, providing insight into its efficiency, specificity, and potential application in various fields. A clear comprehension of how CRISPR-Cas9 works enables researchers to utilize this tool effectively, optimize protocols, and address scientific challenges by enhancing genetic manipulation capabilities.
CRISPR Components Overview
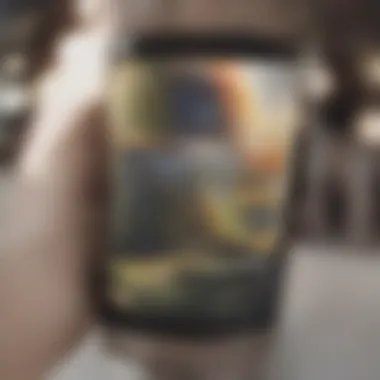
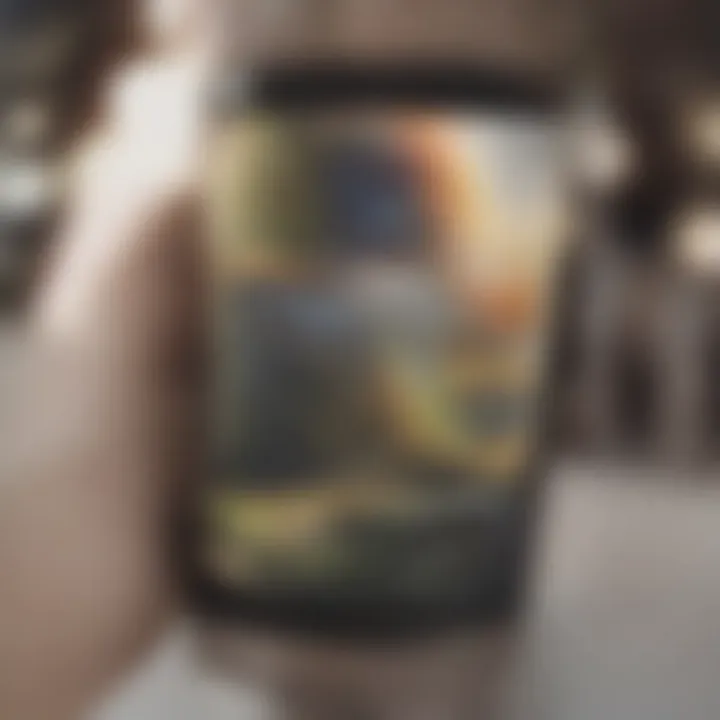
To grasp the function of CRISPR-Cas9, it is essential to recognize its core components. This system comprises two primary elements: the CRISPR RNA (crRNA) and the transactivating RNA (tracrRNA), which guide the Cas9 nuclease to the targeted DNA sequence. The Cas9 protein acts as molecular scissors, making precise cuts in the DNA. The interaction among these elements determines the overall functionality and specificity of the CRISPR approach.
Key components include:
- crRNA: Provides the sequence homology necessary to target specific genes.
- tracrRNA: Associates with crRNA and facilitates the binding to Cas9, forming a guide complex.
- Cas9 Protein: Executes the double-strand break in the target DNA, a critical step for editng the genome.
Understanding the roles of each component aids scientists in designing effective guide RNAs tailored for specific applications, improving the likelihood of successful gene editing.
DNA Targeting Process
The DNA targeting process is crucial in ensuring that CRISPR-Cas9 edits occur accurately at designated loci within the genome. This process begins with the formation of a complex between the guide RNA and the Cas9 protein, allowing the system to scour the genome for complementary sequences. Upon recognizing a match with the target DNA, the tracrRNA and crRNA stabilize the interaction, positioning the Cas9 for action.
Central to this targeting is the requirement for a protospacer adjacent motif (PAM) sequence. The PAM is essential as it distinguishes between potential off-target sites and actual targets, thus preventing unintentional genome alterations. The precise recognition of the target sequence greatly enhances the effectiveness of gene editing while minimizing the risk of adverse effects, a crucial consideration in both research and therapeutic settings.
Double-Strand Break Formation
Double-strand break formation is a vital outcome of the CRISPR-Cas9 editing process. Following successful target recognition, the Cas9 protein introduces a break in both strands of the DNA helix. This break triggers the cell’s inherent DNA repair mechanisms, which can be harnessed for gene editing.
There are two primary pathways through which cells can repair double-strand breaks:
- Non-Homologous End Joining (NHEJ): Often leads to insertions or deletions (indels) at the break site, potentially resulting in gene knockout.
- Homologous Recombination (HR): If a donor template is supplied, the repair can result in precise edits, such as gene insertion or correction.
Understanding these repair mechanisms allows scientists to design experiments that favor desired outcomes, whether it be knocking out genes to study their functions or introducing specific changes to create models for disease research.
In summary, the mechanism of action of CRISPR-Cas9 is a transformative process that combines RNA-guided specificity with controlled gene editing. A firm grasp on these principles is essential for successful application in various biological fields.
CRISPR-Cas9 Protocol Steps
The CRISPR-Cas9 protocol represents a pivotal advancement in the field of genetic engineering. This section covers the essential steps that researchers must follow to successfully implement the CRISPR-Cas9 technology in their projects. Understanding these steps is crucial for achieving precise genome editing and maximizing the potential of this innovative tool.
Designing Guide RNA
Criteria for Target Selection
Selecting the appropriate target sequence is essential in the CRISPR-Cas9 process. The most significant aspect of Criteria for Target Selection is the specificity of the RNA guide designed to bind to the target DNA. A prime characteristic of this selection is that the target sequence must have a near-perfect match with the guide RNA, ensuring accurate binding and editing. This precision mitigates off-target effects, which can lead to unintended mutations, making it a critical focus for effective gene editing projects. Moreover, the unique feature of leveraging specific motifs, such as the protospacer adjacent motif (PAM), is crucial since these motifs influence the binding efficiency of the Cas9 enzyme and consequently the success rate of genome editing studies.
Tools for Designing Guide RNA
Tools for Designing Guide RNA play a vital role in streamlining the CRISPR-Cas9 process. These tools facilitate the rational design of guide RNA sequences by using algorithms that predict off-target effects and potential efficiency. A key characteristic of these tools is their user-friendly interfaces and predictive capabilities. They often allow researchers to input a target sequence and receive guidance on optimal gRNA designs within minutes. One unique feature that stands out is the availability of online platforms like CRISPR Design Tool, which assists in selecting effective gRNA candidates while considering the specific genomic context of the research organism. However, disadvantages do exist, such as the possibility of relying too heavily on computational predictions without experimental validation.
Cloning the Cas9 Gene
Vector Selection
Vector Selection is fundamental to successfully host the Cas9 gene for expression in the target cells. The most significant aspect here is ensuring that the chosen vector can efficiently carry the Cas9 gene, along with the necessary regulatory elements like promoters and termination signals. A prominent characteristic of common vectors is their ability to accommodate various selection markers, enabling the identification of successfully transfected cells. Additionally, the vectors used often provide compatibility with various host organisms, enhancing their versatility. A unique feature is the adaptability of backbone plasmids, allowing researchers to incorporate restrictions sites or additional sequences as needed for their specific experiments. Nonetheless, the downside can involve complexity in choosing the correct vector from the vast range available, which can be overwhelming for researchers.
Transformation into Host Cells
Transformation into Host Cells is the step where the Cas9 gene is introduced into the target organism’s cells. The importance lies in effectively establishing these foreign DNA sequences within the host cells for subsequent expression of the Cas9 protein. A critical feature of this process is the efficiency with which the transformation method can deliver the plasmid, as variations in methods can dramatically influence outcomes. For example, electroporation usually achieves higher transformation rates than traditional methods. A unique aspec of transformation into host cells involves optimizing conditions for specific host types, which can significantly improve gene integration rates. However, drawbacks may arise, including cellular stress or toxicity associated with specific transformation techniques, which can lead to reduced cell viability.
Transfection Methods
Lipofection
Lipofection is a widely used transfection method that capitalizes on lipid-based carriers to deliver genetic material into cells. Its contribution to the CRISPR-Cas9 protocol is extensive, especially due to its relative simplicity and ability to transfect a variety of cell types. A key characteristic of lipofection is that it often achieves high transfection efficiency with lower cytotoxicity compared to other methods. The unique aspect of lipofection comes from its ability to encapsulate both the guide RNA and the Cas9 protein, allowing for synchronous delivery. However, its downsides include potential variability in efficiency based on lipid formulations and cell types.
Electroporation
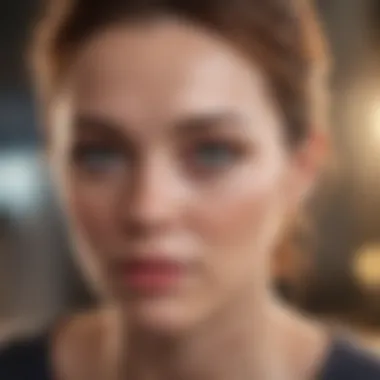
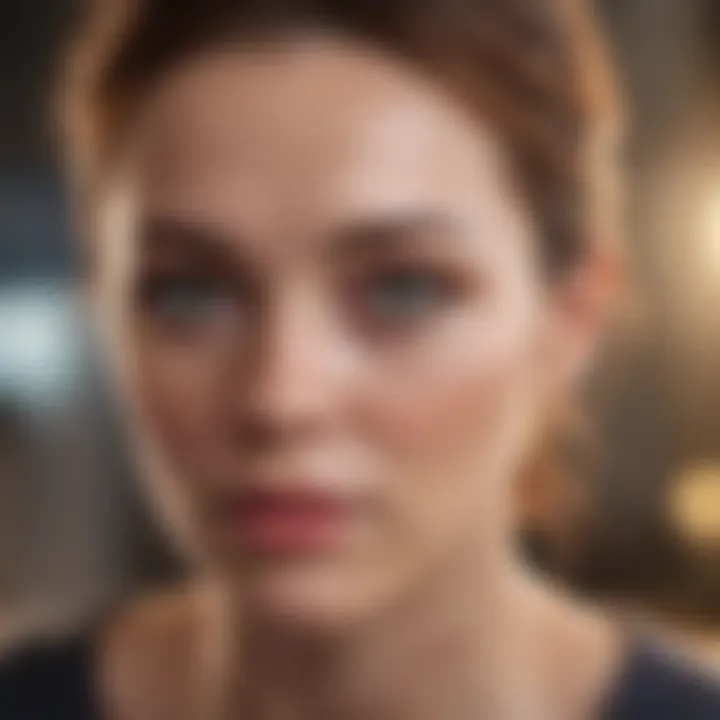
Electroporation stands out as another effective transfection technique where an electric field is applied to increase cell membrane permeability. This method contributes significantly to the protocol by enhancing the uptake of genetic constructs, including the CRISPR-Cas9 components. The primary characteristic of electroporation is its high efficiency, particularly in hard-to-transfect cell types, such as certain primary cells. An important feature is the precise control over the electric fields applied, which can be adjusted to optimize performance for specific cell lines. On the other hand, disadvantages include potential cellular damage due to high voltages or improper timing, leading to concerns about cell viability.
Viral Delivery
Viral Delivery methods utilize modified viruses to introduce genetic material into cells. This technique is known for its ability to achieve stable expression of the CRISPR-Cas9 components. The core strength of viral delivery lies in its high transduction efficiency and capability to infect a wide range of cell types, including non-dividing cells. A unique feature of viral vectors, like lentiviruses, is their capacity for integrating into the host genome, allowing for long-term expression of Cas9. However, one must consider potential safety concerns associated with the use of viral systems, as well as regulatory hurdles when developing gene therapies in clinical settings.
Selection and Screening of Positive Clones
Antibiotic Selection
Antibiotic Selection is crucial in identifying successfully edited cells after introducing CRISPR components. This process involves applying selective pressure to only allow cells that have integrated the resistance gene to survive. The key characteristic here is the reliability of antibiotic selection as a straightforward means to isolate transformed cells. One unique aspect is the timing of antibiotic application, which can influence the rates of successful clone identification. While this method is effective, it also presents disadvantages, such as the need for careful titration of antibiotic concentrations to balance between sufficient pressure and unnecessary lethality.
Genotyping Techniques
Genotyping Techniques are fundamental in confirming successful genome editing. The importance of these techniques lies in their capacity to verify the specific edits made to the target DNA. A key characteristic is the ability to employ various molecular techniques, such as PCR and sequencing, to validate changes at the genetic level. A unique feature of genotyping is the application of methods like Sanger sequencing or next-generation sequencing, which offer detailed insights into the modification outcomes. However, there can be challenges, such as the complexity of analyzing sequencing data and distinguishing between true edits and sequencing artifacts.
Applications of CRISPR-Cas9 Technology
The application of CRISPR-Cas9 technology has significantly influenced various fields, including basic research, agriculture, and medicine. Understanding these applications provides insight into the potential of this genome editing tool and its practicality in real-world scenarios. CRISPR-Cas9 offers reliable solutions for tasks like gene editing and gene therapy, emphasizing its versatility and efficiency. Moreover, with advancements occurring rapidly, keeping informed about these applications is crucial for researchers and practitioners alike.
Basic Research Applications
Gene Function Studies
Gene function studies using CRISPR-Cas9 are essential for understanding the role of specific genes in biological processes. In these studies, researchers can deactivate or modify genes in model organisms, observing resulting phenotypic changes. This approach allows for direct assessment of gene function and interaction with other biological components. One key characteristic of these studies is their ability to provide rapid and precise results, enhancing their appeal.
The unique feature of gene function studies is the manner in which they enable scientists to dissect complex genetic interactions. While advantageous in promoting swift breakthroughs, such studies can also present challenges such as off-target effects, which may complicate results.
Model Organisms
Model organisms are a cornerstone in the study of gene function, particularly with CRISPR-Cas9 technology. Species like Drosophila melanogaster and Mus musculus are commonly used due to their genetic accessibility. These organisms facilitate a comparison of genetic changes in controlled environments. Their key characteristic lies in their evolutionary relevance and significant genetic similarities to humans. Because of these advantages, they are pivotal for advancing basic research in genetics.
The strength of using model organisms is their ability to integrate and express human-relevant genetic traits in a simplified manner. However, the disadvantage can be the limitations in translating findings from these organisms directly to humans, due to differences in complexity and context.
Biotechnology and Agriculture
Crop Improvement
Crop improvement is a critical area where CRISPR-Cas9 shows substantial promise. Through targeted gene editing, researchers can enhance traits such as drought resistance or nutrient content. The efficiency of this technology allows for modifications that traditional breeding methods often cannot achieve. A significant advantage is the precise nature of the edits, which minimizes unintended alterations and increases edible crop varieties.
The unique feature of CRISPR-Cas9 in this context is its ability to create rapid changes in plant genetics, leading to accelerated development of new crop varieties. This acceleration can lead to challenges in regulatory approval and public perception about genetically modified organisms (GMOs).
Gene Therapy
Gene therapy stands as a beacon of hope for treating genetic diseases. By utilizing CRISPR-Cas9 technology, it is possible to directly correct mutations at the source. This tailored approach can potentially restore normal function to genes that would otherwise result in disease. A key characteristic of gene therapy is its focus on delivering a permanent solution rather than temporary fixes.
The unique aspect of gene therapy using CRISPR-Cas9 is its adaptability, allowing different methods for delivery into human cells. While gene therapy presents significant advantages, it can also encounter ethical considerations and safety concerns that demand careful scrutiny.
Potential in Medicine
Genetic Disorders
The impact of CRISPR-Cas9 on genetic disorders is profound. The ability to directly correct the mutations causing diseases like cystic fibrosis or sickle cell anemia offers exciting possibilities. One major characteristic of this application is the specific targeting of problematic DNA sequences, thus providing a precision that is hard to achieve with conventional therapies.
A unique feature is the extensive potential to customize treatments based on individual genetic profiles. However, pitfalls concerning the long-term effects and unintended genetic consequences pose concerns that need ongoing exploration.
Cancer Research
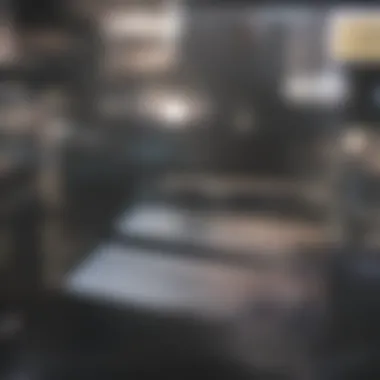
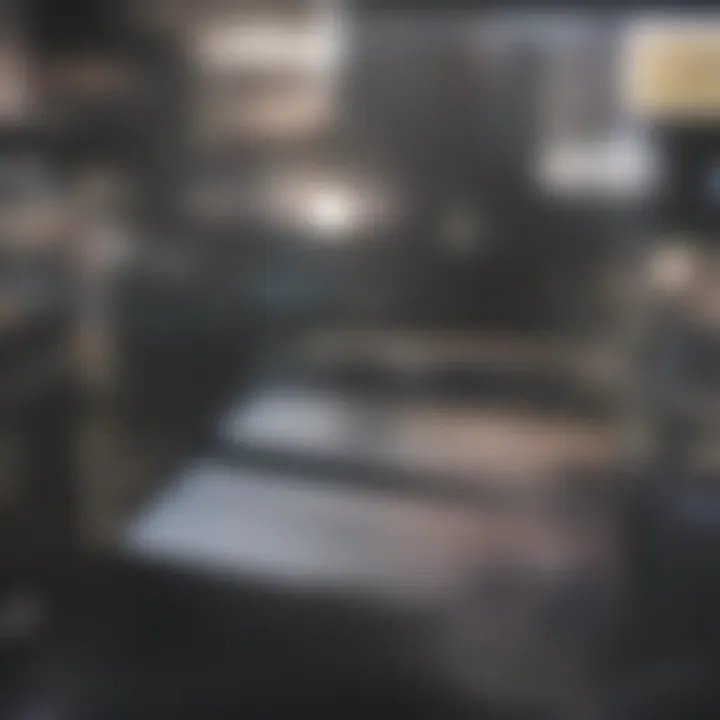
In cancer research, CRISPR-Cas9 serves as a tool for understanding tumorigenesis and developing targeted cancer therapies. By modifying genes associated with cancer progression, researchers can better comprehend the molecular mechanisms driving various cancers. The key aspect of cancer research with CRISPR-Cas9 is its capability to explore both therapeutic and preventative approaches.
One compelling feature is the technology's ability to generate accurate cancer models, which helps in the understanding of cancer biology. Although promising, the varying effectiveness of CRISPR methods across different cancer types presents a challenging landscape for ongoing research.
CRISPR technology represents a formidable opportunity to revolutionize the landscape of healthcare and agriculture, enabling substantial enhancements in both fields.
This overview encapsulates significant applications of CRISPR-Cas9 technology, emphasizing its dynamic role in advancing scientific knowledge and practical solutions across various sectors.
Ethical Considerations and Challenges
The advent of CRISPR-Cas9 technology has not only brought significant advancements in genetic engineering but has also sparked a myriad of ethical considerations. It is crucial to address these issues as they dictate the responsible use of this powerful tool. Ethical discussions in gene editing encompass safety concerns, regulatory limitations, and public perceptions. Each aspect influences not only research but the broader application in society.
Safety Issues in Gene Editing
Safety remains a paramount concern in genetic editing. The potential for unintended consequences from CRISPR technology raises alarms among scientists and ethicists. Off-target effects, where unintended sections of the genome are altered, can lead to harmful mutations. Moreover, the long-term repercussions of such modifications are still largely uncharted. For instance, gene drives, which aim to spread genetic alterations through populations, pose ecological risks that need thorough examination. Addressing these safety issues is essential for gaining public trust and ensuring ethical responsibility within research environments.
Regulatory Frameworks
Implementing suitable regulatory frameworks is vital to govern the use of CRISPR technology. Various countries have established guidelines to manage gene editing, ensuring that it aligns with ethical standards and safety measures. The challenge, however, is that these regulations vary widely. For example, while the United States allows certain types of research, countries such as Germany impose strict bans on germline editing. Organizations like the National Institutes of Health and similar bodies across the globe play a critical role in setting and enforcing these guidelines. A harmonized regulatory approach could greatly enhance the responsible application of CRISPR-Cas9.
Public Sentiment on Genetic Engineering
Understanding public sentiment towards genetic engineering is essential. The acceptance of gene editing technologies like CRISPR-Cas9 can influence funding, policy, and research directions. Surveys indicate that while some people embrace potential benefits, such as disease prevention, others express concerns over ethical implications. Issues of equity in access to such technologies also arise. Moreover, misinformation surrounding gene editing fuels apprehension among the public. Engaging in transparent conversations about the benefits and risks is critical.
"The discourse on gene editing goes beyond the scientific community; it encompasses societal norms and ethical dilemmas that need to be addressed collectively."
In summary, the ethical considerations surrounding CRISPR-Cas9 technology are fundamental. They are not only critical in shaping research directions but also in determining how society perceives and utilizes genetic engineering advances. As the technology develops, nuanced approaches addressing safety, regulatory frameworks, and public sentiment will be vital for its ethical deployment.
Future Directions
In the landscape of modern biology, the future directions of CRISPR-Cas9 technology hold significant promise. Understanding these dimensions is crucial for researchers, educators, and professionals who engage with genetic editing. The ongoing evolution in CRISPR applications suggests a trajectory that not only amplifies its utility but also challenges ethical and practical boundaries in science. This section reveals potential pathways, emphasizing advancements and new applications that are at the forefront of this technology's expansion.
Advancements in CRISPR Technology
The realm of CRISPR technology continues to evolve rapidly. Recent innovations have improved the precision and efficiency of DNA editing. Some of the notable advancements include enhanced Cas9 variants, like SpCas9-NG, which allow for a wider range of target sites. These improvements increase the versatility of gene editing, making it applicable to more complex genomes.
Another significant direction is the development of CRISPR systems that do not induce double-strand breaks. Techniques such as base editing and prime editing allow researchers to make more exact modifications. Base editing converts one DNA base into another with minimal off-target effects, while prime editing enables targeted insertions and deletions without cutting both strands of DNA, significantly reducing unwanted consequences.
Moreover, the integration of CRISPR technology with next-generation sequencing (NGS) is paving a new way for high-throughput screening and genome-wide association studies. Such synergy enhances not only the identification of gene functions but also the therapeutic potential for treating genetic disorders.
Expanding CRISPR Applications
The applications of CRISPR technology are not confined to traditional gene editing tasks. They extend into several diverse fields. In agriculture, CRISPR is being utilized to develop crops that are resistant to diseases, pests, and environmental stresses. Crops like CRISPR-edited soybeans aim for higher yields, signaling benefits for food security worldwide.
In medicine, CRISPR's potential extends to treating genetic disorders and complex diseases. For example, trials are ongoing where CRISPR is applied in therapies for conditions such as sickle cell disease and beta-thalassemia. These therapeutic approaches demonstrate an ability to correct or modify faulty genes.
Additionally, CRISPR shows great potential in the realm of infectious diseases. Its application in diagnosing viruses, such as in the case of COVID-19 testing, highlights its versatility. Tools like SHERLOCK and DETECTR developed from CRISPR technology can rapidly identify the presence of pathogens, making diagnostics faster and more efficient.
"The advancements and expanding applications of CRISPR technology not only unlock new possibilities in science but also require careful consideration of ethical implications and safety considerations."
Ending
The conclusion serves as a pivotal section of any comprehensive guide, as it encapsulates the essence of the information presented. In the context of CRISPR-Cas9, this article's conclusion reiterates the significant advancements in genetic research made possible by this technology. The CRISPR-Cas9 protocol offers a revolutionary approach to gene editing, making it easier and more precise than traditional methods.
Throughout this article, we have explored numerous aspects of CRISPR-Cas9, paying attention to its mechanism, applications, and the ethical considerations that arise from its use. With the rise of CRISPR-Cas9, researchers can conduct gene functions studies more efficiently and develop innovations in agriculture and medicine, underscoring the broad impact of this technology across various disciplines.
Furthermore, ethical considerations cannot be overlooked. As gene editing technologies advance, they confront society with complex questions about safety, regulation, and public perception. These factors should steer ongoing discussions around the use of CRISPR-Cas9 and its implications for future biological research.
In summary, the importance of the conclusion lies in synthesizing knowledge, emphasizing the benefits of understanding CRISPR-Cas9, and recognizing the inherent responsibilities involved. Understanding this technology will not only enhance scientific inquiry but also guide policy and public discourse regarding its applications in the future.
"The future of CRISPR-Cas9 is not just in the laboratory, but in our society as a whole, shaping how we approach genetic engineering ethically and safely."
By appreciating the full scope of CRISPR-Cas9, students, researchers, educators, and professionals alike can better leverage this powerful tool to address the biological challenges faced today.