Carbon Capture into Fuel: Technologies and Impact
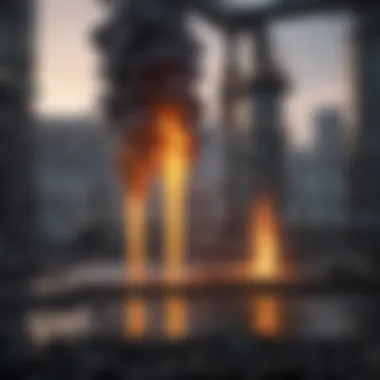
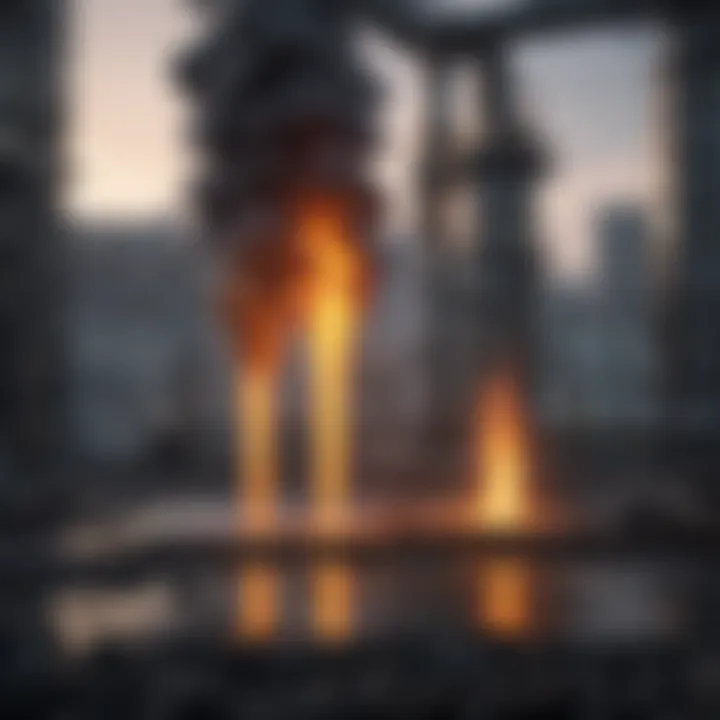
Intro
Carbon capture technology represents a crucial frontier in the fight against climate change. The ability to extract carbon dioxide from the atmosphere and transform it into usable fuel not only addresses the pressing need for cleaner energy sources but also offers a significant opportunity to manage the environmental impacts of fossil fuel consumption. This article will delve into the fascinating interplay between carbon capture and fuel production, examining the technological advancements, environmental ramifications, and the economic dynamics surrounding this innovative approach.
Background and Context
Overview of the Research Topic
Carbon capture, often referred to as carbon capture and storage (CCS), is gaining traction as a method to combat climate change. The process involves capturing carbon dioxide from the air or industrial processes before it can enter the atmosphere. Once captured, this CO2 can be converted into a variety of fuels, such as methanol or synthetic natural gas. This transformation presents a dual benefit: reducing greenhouse gas emissions while simultaneously generating energy.
Historical Significance
The concept of carbon capture emerged in the late 20th century when scientists recognized that rising levels of atmospheric CO2 were contributing to global warming. Early studies focused on identifying viable capture techniques, and over the years, various pilot projects have been initiated worldwide. These efforts set the stage for more extensive research and development, leading to the technologies we see today.
Key Findings and Discussion
Major Results of the Study
Research indicates that integrating carbon capture technology with existing energy infrastructures can significantly reduce emissions. According to a recent study, facilities that adopted carbon capture reported a decrease in carbon output of up to 90%. This showcases the potential effectiveness of the technology in achieving emission reduction targets.
Detailed Analysis of Findings
Critical examination of carbon capture methodologies reveals several innovative processes.
- Direct Air Capture (DAC): This technology extracts CO2 directly from ambient air using chemical processes.
- Post-Combustion Capture: This method captures CO2 from flue gases emitted after fossil fuels are burned.
- Bioenergy with Carbon Capture and Storage (BECCS): In this process, biomass is used as a source of energy, and the resultant CO2 is captured before being stored underground.
Effectively all these methods have their merits and they contribute to the broader goal of reducing carbon emissions significantly. The ongoing improvements and upgrades in technology mean that the costs associated with carbon capture are projected to decrease in the coming years, making it increasingly economically viable.
"Carbon capture technology can reduce our dependence on fossil fuels while helping us manage climate change."
Furthermore, the environmental implications of using captured carbon as a fuel source are profound. Not only does it limit waste emissions, but it also substitutes traditional fossil fuels, thereby reducing overall carbon buildup in the atmosphere.
The End
The integration of carbon capture into fuel production could redefine energy sustainability. By processing captured CO2 into usable fuel, this technology essentially closes the loop in the carbon cycle, facilitating a more sustainable approach to energy needs. As research continues and technology improves, carbon capture may become a standard practice, significant for both environmental stewardship and energy production.
Continuing to investigate these technologies is vital to both advancing the science and understanding the economic implications and sustainability potential.
Prolusion to Carbon Capture
The rising challenges posed by climate change demand multifaceted solutions. Among these, carbon capture emerges as a critically important technology. It offers an innovative approach to reducing carbon dioxide emissions, a prominent greenhouse gas, through direct intervention in various industrial processes and the atmosphere itself. This technology has the potential not only to mitigate climate change but also to convert captured carbon into usable and renewable fuel, contributing to energy sustainability. In this section, we will explore the definition and significance of carbon capture, alongside its historical evolution and context, laying the groundwork for understanding the full scope of its applications and implications.
Definition and Importance
Carbon capture refers to the methods and technologies used to capture carbon dioxide emissions from sources like power plants and industrial processes before the gas can enter the atmosphere. Once captured, this carbon dioxide can either be stored underground or transformed into fuel through various chemical and biological processes. The importance of carbon capture lies in its potential to significantly reduce the amount of greenhouse gases in the atmosphere, thus addressing one of the driving factors behind global warming.
Key benefits of carbon capture include:
- Reduction of Carbon Emissions: This technology can capture up to 90% of CO2 emissions from a point source, thereby lessening environmental impact.
- Conversion to Fuel: Captured CO2 can be used as a feedstock to produce fuel, creating sustainable energy solutions.
- Economic Opportunities: Developing carbon capture technology can lead to new job creation in technology development, installation, and maintenance.
Historical Context
The concept of carbon capture has its roots in the early efforts to understand greenhouse gas emissions and their effects on climate change. Initial research dates back to the 1970s, when scientists began to recognize the role of carbon dioxide in global warming. As awareness grew, so did the urgency to find solutions.
By the 1990s, pilot projects were setup, primarily focused on carbon capture and storage (CCS). These early ventures paved the way for more advanced techniques and raised awareness about efficacy and potential applications. For instance, the Sleipner project in Norway, initiated in 1996, became the first commercial project to store CO2 underground in a saline aquifer. Since then, interest and funding for carbon capture infrastructures have increased, leading to numerous advancements in technologies and methodologies that support both carbon capture and conversion into energy sources.
"Historical developments in carbon capture highlight the transition from theoretical concepts to real-world applications, demonstrating its viability in modern energy discussions."
In summary, carbon capture represents a critical technology in the fight against climate change. Understanding its definition and importance, as well as its historical background, forms the foundation for a deeper exploration of its implementation, technologies, and future prospects.
Sources of Carbon Emissions
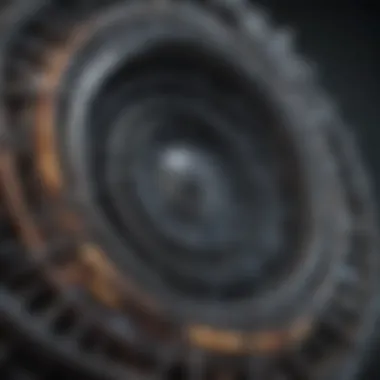
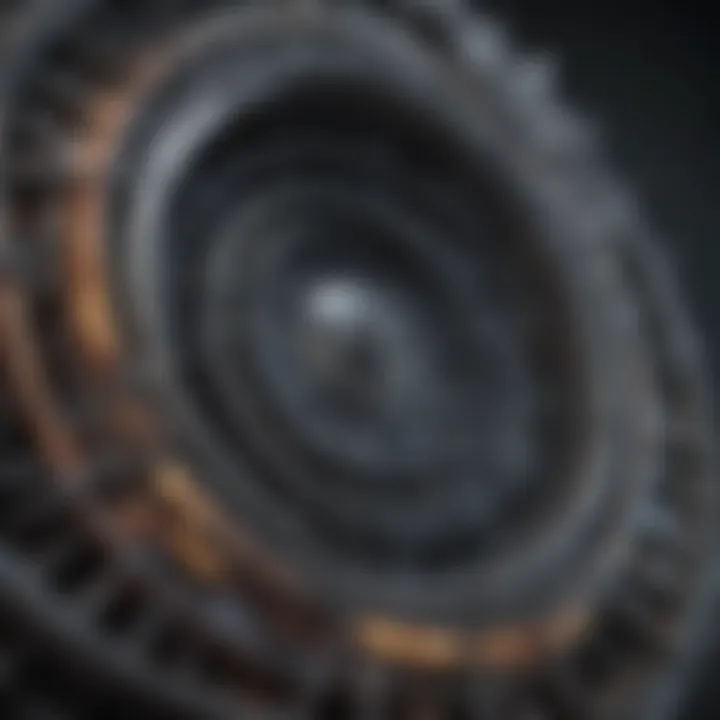
Understanding the sources of carbon emissions is critical to comprehending the relevance of carbon capture technologies. Much of our modern infrastructure and lifestyle depends on energy derived from fossil fuels. This dependency contributes significantly to the carbon dioxide released into the atmosphere. Identifying these sources helps in targeting specific areas for emissions reduction. The information gleaned can guide not only policy but also technological advancements in carbon capture and utilization.
Industrial Origins
The industrial sector is one of the largest contributors to carbon emissions. Industries such as cement, steel, and chemical manufacturing release substantial amounts of carbon dioxide as a by-product of various processes. For example, cement production alone contributes about 8% of global emissions. This is due to the decomposition of limestone into lime, which releases CO2.
The high energy demand of industry often relies on fossil fuels, heightening carbon emissions further. In contrast, transitioning to cleaner and more efficient production methods can significantly reduce carbon footprints.
Furthermore, industries can benefit from implementing carbon capture technologies. These systems can be integrated with existing facilities to capture emitted CO2 before it reaches the atmosphere. Such methods not only help in reducing greenhouse gases but also in creating opportunities for reusing the captured carbon as a resource for fuel synthesis.
Transportation Sector
The transportation sector also significantly contributes to carbon emissions. This includes all modes of transport like cars, trucks, ships, and airplanes. According to data, it accounts for roughly 24% of global greenhouse gas emissions. The reliance on petrol and diesel fuels links directly to emissions from vehicles.
Innovations in vehicle technology, such as electric and hybrid vehicles, are necessary to mitigate this impact. However, these alternatives still depend on energy sources for charging and manufacturing. Thus, if the underlying energy is derived from fossil fuels, emissions will continue.
Carbon capture can play a role here as well. Developing and investing in infrastructure for carbon capture at refineries and other fuel-producing sites may reduce emissions associated with various fuels. Additionally, promoting public transportation and non-motorized transport options can also contribute to emissions reductions.
The choices made in both industry and transportation have ramifications far beyond immediate emissions.
In summary, the sources of carbon emissions stem primarily from industrial activities and transportation. Addressing these sources through innovative technologies and sustainable practices is essential for achieving significant reductions. This understanding sets the stage for exploring how captured carbon can be transformed into usable fuels.
Carbon Capture Technologies
Carbon capture technologies are crucial in the context of mitigating climate change. They offer methods to capture carbon dioxide emissions before they enter the atmosphere or remove CO2 already present in the air. By transforming captured carbon into fuel, these technologies provide a dual benefit: reducing greenhouse gases while producing usable energy sources. The advancements in these technologies could shape a more sustainable future, serving both environmental and energy needs.
Direct Air Capture
Direct air capture (DAC) is a process that actively removes CO2 from the atmosphere. This technology employs large fans to pull in air and passes it through a chemical solution that binds with the carbon dioxide. The captured CO2 can be stored or utilized in various applications, including the production of synthetic fuels. One advantage of direct air capture is its ability to operate independently from emission sources. However, DAC faces criticism due to its energy requirements and the current high costs associated with its implementation.
"To make DAC economically viable, continued advancements in technology and reductions in operational costs are essential."
Point Source Capture
Point source capture focuses on capturing CO2 emissions from specific sources, such as power plants or industrial facilities. The process involves using absorption, adsorption, or membrane separation techniques to concentrate CO2 before it is released into the atmosphere. This technology is generally more developed than DAC, as it targets existing sources of emissions. Its major challenge lies in the integration into current industrial processes. Existing infrastructure might require significant modifications to accommodate these systems effectively.
Biological Carbon Capture
Biological carbon capture utilizes natural processes to absorb CO2. This includes photosynthesis performed by plants and algae. Certain methods involve cultivating algae in bioreactors to enhance their CO2 absorption capabilities. Biological methods often provide additional benefits, such as the generation of biomass, which can be further utilized for energy or as feedstock for various industries. One challenge of this method is the variability in effectiveness depending on environmental conditions and the types of organisms used. Therefore, research into optimizing these biological systems is ongoing, focusing on scalability and efficiency.
The Process of Converting CO2 to Fuel
Understanding the process of converting carbon dioxide (CO2) to fuel is critical in addressing climate change. This topic underlines the potential for turning greenhouse gases into valuable energy sources. The conversion processes not only mitigate emissions but also contribute to energy production efficiency. Efficient CO2 conversion could enhance energy security and sustainability, thereby securing a cleaner environment for future generations.
Chemical Conversion Methods
Chemical conversion methods form the backbone of how CO2 is transformed into usable fuel. Several approaches exist, each with unique mechanisms and efficiencies. Here are some common methods:
- Thermochemical conversion: Involves high temperature to drive reactions that convert CO2 into hydrocarbons. This method utilizes heat, often from renewable sources, enhancing the sustainability profile.
- Electrochemical reduction: This approach uses electricity to convert CO2 into hydrocarbons, alcohols or other fuels. Renewable energy can power the electrochemical cells, aligning with clean energy objectives.
- Biochemical processes: These utilize microorganisms or enzymes to convert CO2 into organic compounds. This method reflects natural processes, potentially leading to a sustainable pathway for fuel production.
Each conversion method carries specific advantages and challenges. Efficiency, cost, and scalability remain critical factors in determining their real-world application.
Fuel Synthesis Pathways
Once CO2 is chemically converted, the next step is synthesizing it into fuel. Various fuel synthesis pathways exist which further process the converted CO2 into different forms of fuel:
- Methanol synthesis: CO2 can be converted into methanol, a versatile fuel and chemical feedstock. This process involves multiple steps, including hydrogenation, and can be fed into existing infrastructure.
- Fischer-Tropsch synthesis: This pathway converts CO2 into liquid hydrocarbons, potentially usable as diesel and jet fuel. This method leverages a popular catalyst and requires CO or hydrogen, which can be obtained from CO2.
- Biomass pathways: Integrating biological processes with chemical conversion can generate fuels with lower emissions. The symbiosis between agriculture and energy generation expands the renewable energy footprint.
"The synthesis of fuel from CO2 represents a unique opportunity to both harness energy needs and combat global warming."
Each synthesis pathway offers insights into how carbon capture can transform the energy landscape. Recognizing these processes can influence policy and investment decisions in sustainable energy sectors.
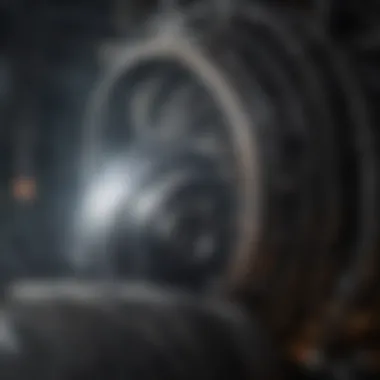
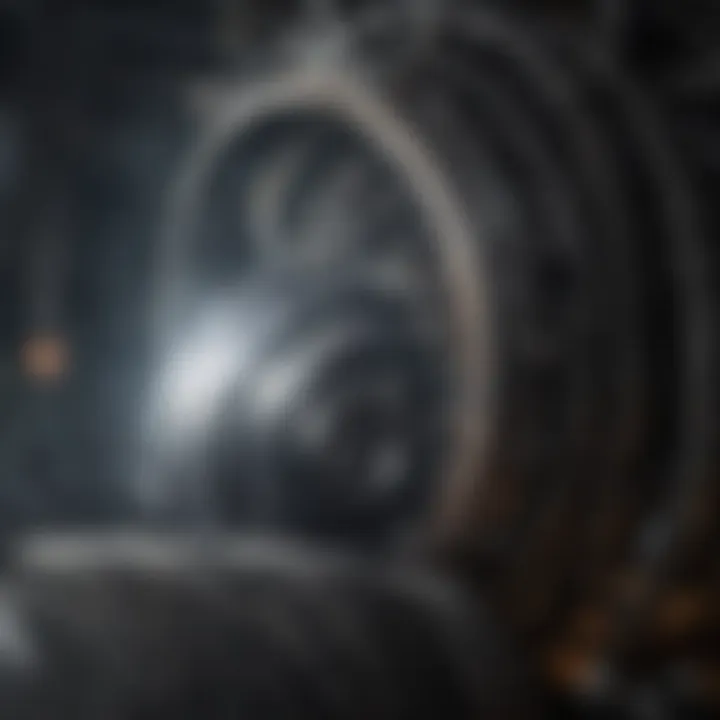
Environmental Implications
Understanding the environmental implications of carbon capture into fuel is essential for assessing its overall effectiveness. This section delves into the impact carbon capture can have on climate change mitigation and its nurturing relationship with ecosystems. The transformation of captured carbon dioxide into fuel has potential benefits that go beyond simple emission reductions, influencing various ecological and atmospheric processes.
Impact on Global Warming
One of the primary concerns about climate change is global warming. Carbon dioxide is a significant greenhouse gas, contributing to the greenhouse effect and subsequent temperature rise on Earth. By capturing carbon from industrial emissions or the atmosphere itself, we aim to reduce the overall concentration of CO2 in the atmosphere.
Carbon capture technology works by removing CO2 during combustion or production processes, preventing it from entering the atmosphere. This can lead to a measurable drop in global greenhouse gas emissions when implemented on a large scale. Moreover, converting captured CO2 into fuel allows for the utilization of a pollutant, turning it into a resource for energy rather than a liability.
"Reducing atmospheric CO2 is critical in averting the most severe consequences of climate change."
Thus, the effectiveness of carbon capture fuels can play a key role in achieving carbon neutrality and meeting international climate targets. As we pursue energy solutions, it's vital to incorporate strategies that focus not only on reducing emissions but also on controlling warming effects.
Ecosystem Effects
The introduction of carbon capture into energy systems presents various consequences for ecosystems. These effects can be both positive and negative. On one hand, capturing CO2 can lead to decreased acidity in oceans, benefiting marine life. High levels of CO2 contribute to ocean acidification, which harms coral reefs and aquatic organisms.
On the other hand, the technologies used in carbon capture fuel processes can have adverse effects on land and water resources. For instance, the infrastructure required for large-scale carbon capture may disrupt local habitats. Additionally, the energy-intensive nature of some capture processes could exacerbate other environmental issues, such as water usage and land degradation.
Therefore, careful evaluation of ecosystem impacts is crucial. Striking a balance between technological advancements in carbon capture and protecting biodiversity should be a priority. The net effects of carbon capture on ecosystems can vary significantly, depending on the methods used and the local context.
In summary, understanding the environmental implications of carbon capture into fuel involves comprehensive analysis of both its benefits and potential downsides. With careful planning and consideration, carbon capture could contribute positively to global efforts towards a sustainable future.
Economic Viability of Carbon Capture Fuels
The viability of carbon capture fuels is paramount in understanding the intersection of environmental sustainability and economic growth. As the world grapples with climate change, governments and organizations seek methods that lessen carbon footprints while maintaining energy demands. Economic viability involves assessing costs, potential market expansion, and long-term sustainability benefits that carbon capture technologies can offer.
Cost Analysis
Understanding the costs associated with carbon capture fuels is vital. The process of capturing CO2 from the atmosphere and converting it into fuel entails various expenses ranging from initial setup to operational costs. Key considerations in cost analysis include:
- Initial Investment: Capturing carbon necessitates significant capital to establish the appropriate infrastructure. This may involve high technology expenditures and facility modifications.
- Operational Costs: Daily operations also invoke expenses such as maintenance of the technology and energy used in carbon capture processes.
- Scale Economies: Larger installations may reduce per-unit costs. Thus, economies of scale can enhance economic viability by potentially lowering overall costs as production increases.
- Subsidies and Incentives: Government policies play a crucial role. Incentives for carbon capture initiatives can offset costs and bolster investments.
Despite high initial costs, the long-term economic benefits could outweigh expenses. Cost-effective advancements in technology continue to develop, making carbon capture a more attractive option.
Market Potential
The market potential for carbon capture fuels is promising, driven by a global shift towards greener energy solutions. Below are elements that illustrate this potential:
- Growing Demand for Clean Fuels: As public awareness of climate change rises, there is increased demand for sustainable energy sources. Carbon capture fuels can cater to this demand, bridging the gap between traditional fossil fuels and renewable energy.
- Diverse Applications: Carbon-derived fuels can serve various sectors, including transportation, electricity generation, and industry. This diversity strengthens market attractiveness and positioning.
- Investments and Collaborations: Corporations and governments increasingly invest in clean technologies. Partnerships with research institutions enhance innovation and market reach.
- Regulatory Support: Policies that prioritize carbon neutrality and emissions reductions provide a favorable landscape for carbon capture fuels. These regulations can spur growth in the sector, paving the way for extensive deployment of technologies.
Case Studies of Successful Implementation
Case studies play a crucial role in understanding the practical applications of carbon capture technologies. They serve as real-world examples that demonstrate the viability and effectiveness of these methods. Each case study provides insight into the nuances of implementation, helping stakeholders learn from successes and challenges. Overall, these examples significantly contribute to the broader narrative of carbon capture, showcasing its potential role in reducing atmospheric CO2 while generating usable fuel.
Pilot Projects
Pilot projects are essential for testing carbon capture technology on a smaller scale. These projects offer valuable data that help improve the efficiency of various carbon capture methods. They also provide a testing ground for innovations in chemical conversion processes and fuel synthesis pathways. Through these pilot implementations, researchers can refine technologies before scaling them for commercial use.
In several countries, pilot projects have been successfully launched. For instance, the Boundary Dam project in Canada features carbon capture technology integrated within a coal-fired power plant. This project not only captures CO2 emissions but also demonstrates the potential to convert the captured carbon into valuable products like synthetic fuels. It has achieved significant reductions in greenhouse gas emissions since its inception.
Pilot projects like these are vital. They validate the economic and technological feasibility of carbon capture. Moreover, they help in identifying potential risks and necessary regulatory measures in subsequent larger-scale implementations.
Global Initiatives
Beyond localized pilot projects, global initiatives provide a wider framework for collaborative efforts in carbon capture and utilization. Countries and organizations around the world are joining forces to share knowledge, technology, and resources. These global initiatives highlight the urgency of addressing climate change and promote the adoption of carbon capture methodologies.
One notable example is the Carbon Capture and Storage Association (CCSA), which works on advocacy and promotion of carbon capture initiatives globally. It engages in dialogues with governments, industries, and NGOs to enhance the understanding and implementation of carbon capture technologies. Their work emphasizes creating supportive policies and financial incentives that encourage investment in carbon capture projects.
Furthermore, initiatives like the United Nations Framework Convention on Climate Change (UNFCCC) aim to incorporate carbon capture as a key strategy in national climate plans. This global perspective encourages different countries to contribute to shared environmental goals. Through collaboration, these efforts aim to overcome barriers to technology transfer and adoption, making carbon capture solutions more accessible.
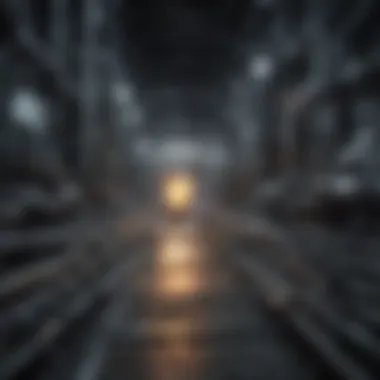
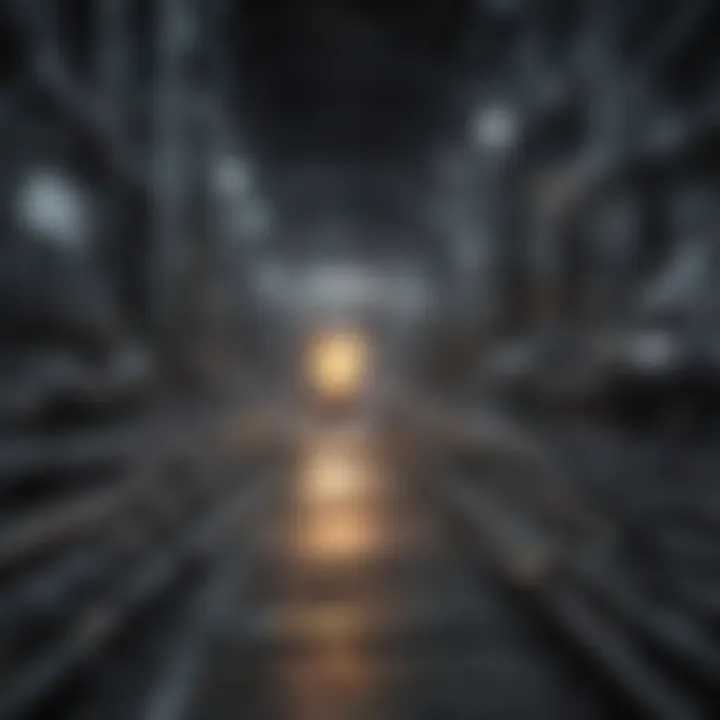
"Through collaborative efforts and pilot projects, the world can accelerate the journey towards sustainable energy solutions while tackling climate change."
Challenges and Limitations
The transition to carbon capture technologies faces distinct challenges that hinder its widespread implementation and effectiveness. Understanding these barriers is crucial for stakeholders in the scientific community, industry, and regulatory bodies as they strategize for future developments. Among these challenges are technological barriers and regulatory hurdles, which are intertwined yet distinctly require focused approaches to tackle.
Technological Barriers
Several technological challenges impede the adoption of carbon capture technologies. First, the efficiency of current carbon capture methods often needs improvement. Technologies such as direct air capture and point source capture require significant energy to operate. This additional energy demand can often offset the reduction in emissions achieved. Additionally, scaling these technologies for industrial applications remains a hurdle. While small-scale pilot projects demonstrate feasibility, larger installations introduce complexities that can increase costs significantly and impact overall efficiency.
Another critical barrier is the need for improved storage solutions. Capturing carbon dioxide is only part of the equation. Safely storing it is an entirely different issue. The lack of established infrastructure for long-term storage limits the potential benefits of carbon capture solutions. Furthermore, the research into sustainable and effective storage methods is still ongoing, leaving uncertainty about the longevity and safety of stored CO2.
"Technological advancement must keep pace with the growing urgency of climate change to enable the effective use of captured CO2."
This combination of energy requirements, scalability issues, and uncertain storage solutions presents a formidable challenge that must be comprehensively addressed to optimize carbon capture systems.
Regulatory Hurdles
In addition to technological challenges, regulatory hurdles play a significant role in the slow progress of carbon capture technologies. Policymaking often lags behind technological advancements, creating an environment where companies may hesitate to invest in carbon capture initiatives. Clear guidelines are necessary for the deployment of these technologies, but existing frameworks frequently do not account for the intricacies of carbon capture and utilization.
Moreover, regulations concerning the long-term storage of captured CO2 can vary widely by region, complicating operations for global enterprises. The uncertainty surrounding liability and accountability for stored carbon adds additional layers of complexity. Firms may be deterred by the potential legal repercussions associated with carbon leakages or failures in storage methods.
Finally, the economic landscapes shaped by policies can also hinder innovation. Subsidies and incentives, or lack thereof, greatly influence companies’ decisions to pursue carbon capture projects. Without robust policy support, many technologies may not achieve their true potential or financial viability, further perpetuating the reliance on fossil fuels.
In essence, overcoming these technological barriers and regulatory hurdles is paramount for the successful integration of carbon capture technologies into the broader energy landscape. Engaging in collaborative efforts between technologists, policymakers, and the industrial sector will be crucial for turning these challenges into opportunities for sustainable development.
Future Prospects of Carbon Capture into Fuel
The future of carbon capture technology in transforming CO2 into fuel holds significant promise. As global awareness of climate change intensifies, the demand for sustainable energy solutions is growing rapidly. Carbon capture, combined with cutting-edge fuel conversion technologies, represents a potential game changer in our efforts to address greenhouse gas emissions while meeting energy needs.
Innovative Research Directions
Current research initiatives are exploring various innovative approaches to enhance carbon capture effectiveness and conversion efficiency. This includes:
- Advancements in Material Science: Researchers are developing new sorbents and catalysts that can improve the efficiency of CO2 capture. Materials like metal-organic frameworks (MOFs) show great potential for higher absorption rates.
- Integration with Renewable Energy: Combining carbon capture processes with renewable sources of power, such as solar and wind energy, can lead to more sustainable fuel production. This synergy aims to reduce reliance on fossil fuels while creating a circular carbon economy.
- Biological Methods: Exploring biological carbon capture techniques, such as algal systems, is gaining traction. Algae can absorb CO2 and produce biofuels, providing a dual benefit in reducing emissions and generating energy.
This research not only focuses on improving current technologies but also seeks to find new methodologies that can be seamlessly integrated into existing industrial processes.
Role in Energy Transition
The transition to a cleaner energy landscape hinges on the successful implementation of carbon capture technologies. It plays a crucial role in several areas:
- Decarbonizing Heavy Industries: Industries like cement and steel manufacturing produce a large share of global CO2 emissions. Carbon capture can significantly reduce these emissions by capturing the CO2 generated in the production process.
- Oil and Gas Sector: Enhanced oil recovery techniques have integrated carbon capture processes to help extract more oil while simultaneously sequestering CO2. This dual approach could ease the transition from fossil fuels to more sustainable energy sources.
- Policy and Investment Influence: The advancement of carbon capture technologies could strengthen policy frameworks that promote cleaner energy. Increased public and private investments can fast-track research and development, leading to more practical applications in energy production.
In summary, carbon capture technology stands at the forefront of our energy transition strategy. By investing and innovating in this area, we can face future energy challenges while simultaneously mitigating climate change.
"The implementation of carbon capture into fuel processes is not just necessary; it is inevitable in our pursuit of a sustainable energy future."
The prospects are bright, and the collaborative efforts among scientists, policymakers, and industries are vital in unlocking the full potential of carbon capture technologies.
Culmination
The conclusion serves as a crucial element in understanding the broader implications of carbon capture technology. It encapsulates the essence of the discussions presented throughout the article, solidifying the importance of transforming carbon dioxide into usable fuel.
Summary of Key Findings
The analysis reveals several key findings:
- Technological Feasibility: Numerous innovative methods exist for capturing carbon and converting it into fuel. Both direct air capture and point source capture technologies have shown promise in various pilot projects.
- Environmental Impact: The transition to carbon-neutral fuel is an essential step in addressing global warming. Carbon capture can significantly reduce the overall carbon footprint of energy production.
- Economic Viability: Market potential for carbon capture fuels appears positive, especially with growing interest from industries to develop sustainable practices. However, cost analysis indicates that substantial investment is still necessary.
- Regulatory Challenges: The presence of regulatory hurdles may affect the pace and scale of implementation. Creating favorable policies is significant for fostering an environment conducive to innovation.
These elements underscore the potential of carbon capture fuels to contribute to energy sustainability while addressing pressing environmental concerns.
Call to Action for Research and Development
Given the urgency of climate change, it is imperative to advocate for continued research and development in the sphere of carbon capture. Investment in technology innovation can facilitate:
- The refinement of existing capture methods to enhance efficiency.
- The establishment of regulatory frameworks that promote sustainable practices.
- Collaboration among governments, educational institutions, and private sector organizations to share findings and best practices.
Researchers and industry leaders must work together to leverage existing advancements in carbon capture technology. This collective effort can not only lead to viable fuel solutions but also contribute to a more sustainable world.