Exploring the Applications and Impact of Spectroscopy
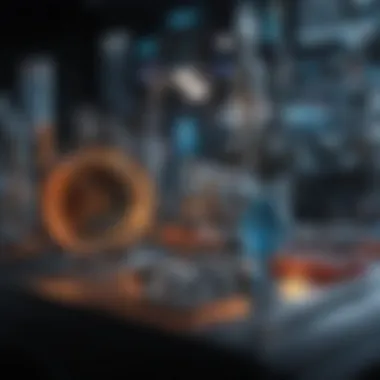
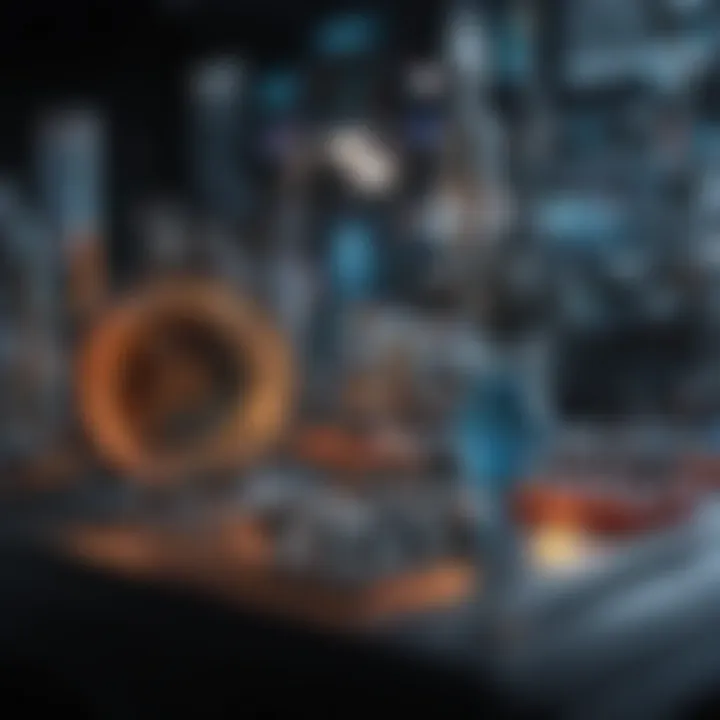
Intro
Spectroscopy stands out as a pivotal technique in scientific inquiry. By analyzing the interaction between matter and light, it provides insights into various material properties and chemical interactions. This article will take a thorough look at the realm of spectroscopy, unveiling its numerous applications across various scientific disciplines.
The key to understanding its significance lies in appreciating how spectroscopic methods evolve over time while fostering advances in research and industry. The integration of traditional and modern techniques creates a landscape rich with potential for discoveries yet to be made.
Background and Context
Overview of the research topic
Spectroscopy encompasses a diverse set of techniques employed to convert light into information. These methods range from basic absorption and emission spectroscopy to advanced techniques such as Raman and nuclear magnetic resonance (NMR) spectroscopy. Each technique serves specific purposes, with some focusing on chemical composition while others examine the physical structure of materials.
Today's research landscape relies heavily on the fundamental principles of spectroscopy to explore new frontiers in chemistry, biology, material science, and physics. This interconnectedness underscores the necessity of a well-rounded understanding of spectroscopic methods.
Historical significance
The origins of spectroscopy date back to the early 19th century with Isaac Newton's experiments on light. Newton's primary contribution was demonstrating that white light breaks down into a spectrum of colors. This historical event laid the groundwork for future advancements in understanding the relationship between light and matter.
In the decades that followed, pioneers such as Joseph von Fraunhofer and Gustav Kirchhoff played crucial roles in developing the field further. The creation of spectroscopy as a structured discipline involved identifying specific spectral lines based on elemental signatures. These breakthroughs paved the way for the robust applications of spectroscopy that we observe today, proving its value in both academic and industrial contexts.
Key Findings and Discussion
Major results of the study
From investigating molecular structures to analyzing star compositions, spectroscopy has demonstrated its versatility. One notable result is its ability to aid in determining the composition of distant celestial bodies through the analysis of their emitted light. Similarly, in biology, spectroscopic techniques find pivotal roles in understanding molecular interactions, thus contributing to breakthrough therapies and diagnostics.
Widespread implementation in quality control processes in industries like pharmaceuticals and food safety also exemplifies the practicality and necessity of spectroscopy. Such applications highlight why many contemporary research projects can be attributed to advancements in spectroscopic techniques.
Detailed analysis of findings
An in-depth look at the various techniques shows that no single method stands alone. Each spectral analysis method complements the others, forming a cohesive framework that enhances accuracy and understanding. For instance:
- Mass Spectrometry: This method provides information about molecular weights, giving insights into chemical structures.
- Infrared Spectroscopy: Commonly used to identify functional groups within compounds, aiding in material characterizations.
- Raman Spectroscopy: It offers advantages in studying molecular vibrations without requiring elaborate sample preparations.
The impact of these techniques extends beyond mere academic curiosity. They facilitate technological innovations and foster industries that are essential for contemporary society. As such, understanding their applications illuminates pathways to advancements in science and technology.
"The synthesis of theoretical principles with practical applications highlights the significance of spectroscopy in understanding material properties and chemical interactions."
Preamble to Spectroscopy
Spectroscopy is a pivotal technique in many scientific fields. The study of how different materials interact with light gives insights into their composition and structure. Understanding spectroscopy is not just about observing colors or patterns; it encompasses significant principles that have driven advancements in various disciplines such as chemistry, biology, and physics. The purpose of this section is to illuminate the concept of spectroscopy, address its historical context, and explain its fundamental principles.
Definition and Historical Context
Spectroscopy can be defined as the study of the interaction between electromagnetic radiation and matter. It allows scientists to analyze, identify, and quantify materials by examining the light emitted, absorbed, or scattered by them. The roots of spectroscopy can be traced back to ancient civilizations, where early philosophers considered the relationship between light and matter.
However, true scientific foundations of spectroscopy were established in the 17th century. Isaac Newton's experiments with prisms revealed how light is composed of different colors, laying the groundwork for further exploration. The 19th century saw the development of various spectroscopic techniques, particularly with the advent of the spectroscope. This instrument allowed for more detailed analysis of spectral lines, leading to fundamental discoveries such as the element composition of stars.
Over time, advancements continued to shape the field. Innovations in technology have enhanced both the resolution and sensitivity of spectroscopic techniques. Today, spectroscopy is a cornerstone of many scientific processes, providing critical data that informs research and industry while continuing its legacy of discovery.
Basic Principles of Spectroscopy
At the heart of spectroscopy lie several basic principles governing its operation. Firstly, the interaction of light with matter produces unique spectral fingerprints. When light hits an atom or molecule, it may be absorbed, emitted, or scattered, depending on the energy levels of its electrons. This interaction results in a spectrum, where wavelengths reveal information about the material's electronic structure.
Moreover, spectroscopy can broadly categorize into different types, depending on the interaction specifics. Absorption spectroscopy measures the light absorbed by samples, while emission spectroscopy assesses the light emitted after excitation. Additionally, Raman spectroscopy employs an inelastic scattering principle, providing insights into molecular vibrations.
These principles are not merely theoretical; they are applied in laboratory settings to carry out various analyses. For instance, by examining spectral patterns, researchers can determine the presence of specific compounds in a mixture. Likewise, the ability to quantify materials based on their spectral characteristics supports applications in field research, quality control, and industrial processes.
Understanding the role of light in spectroscopy opens pathways to countless applications across scientific fields, facilitating innovations and discoveries.
Types of Spectroscopy Techniques
Spectroscopy is an essential tool in modern science. It provides profound insights into the properties of matter through its various techniques. Each spectroscopy technique has unique benefits, applications, and considerations. Understanding these techniques is crucial for students, researchers, and professionals across various fields. The following sections will delve into the major types of spectroscopy, highlighting their principles and specific uses in scientific research and industry.
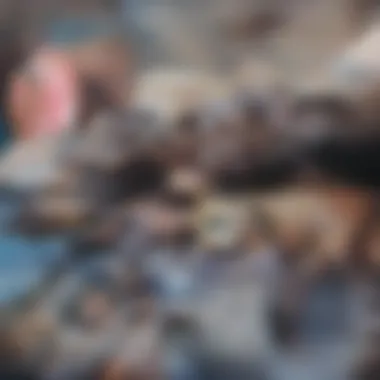
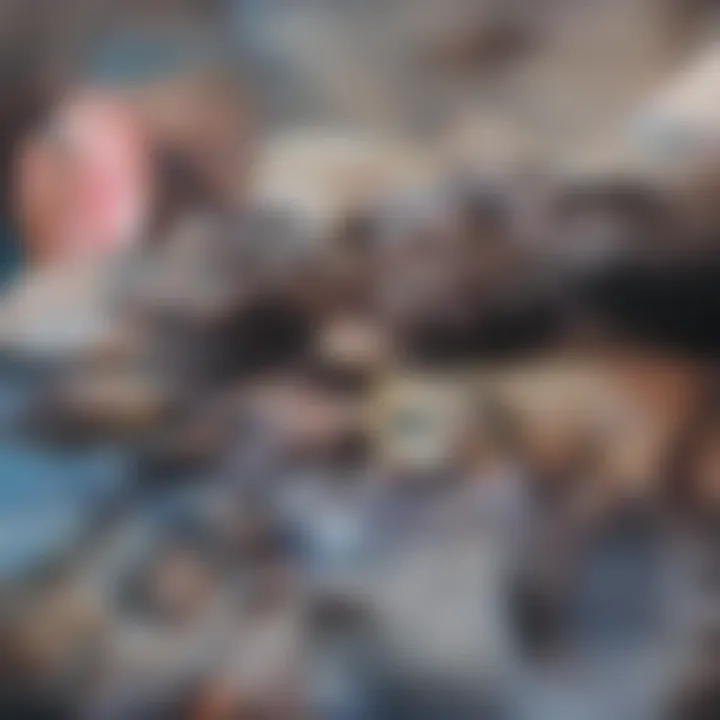
Absorption Spectroscopy
Absorption spectroscopy is a technique where a sample absorbs light at specific wavelengths. This absorption results in a spectrum that reveals information about the chemical composition of the sample. The absorbed wavelengths correlate with the energies of the electronic transitions of the molecules involved.
One significant application of absorption spectroscopy is in the analysis of gases and liquids. For instance, it is widely used in environmental monitoring. Instruments like ultraviolet-visible (UV-Vis) spectrophotometers play a central role in measuring pollutant concentrations in various settings.
Absorption spectroscopy provides insight into molecular structures and concentrations, making it a fundamental technique in analytical chemistry.
Emission Spectroscopy
Emission spectroscopy involves measuring the light emitted by a substance when it is excited by energy. The emitted light is typically from atomic or molecular transitions back to a lower energy state. This specific emission allows for the identification of the elements present in the substances.
A common use is in elemental analysis via flame emission spectrometry. It is particularly beneficial in studying metals in samples. This technique not only helps in determining the elemental composition but also plays a crucial role in many industrial applications, such as quality control in metal production.
Raman Spectroscopy
Raman spectroscopy is a vibrant technique based on inelastic scattering of monochromatic light, usually from a laser. When light interacts with molecular vibrations, it causes a shift in energy levels. The resulting Raman spectrum provides distinct information about molecular vibrations, which is useful for identifying molecular structures.
This method is especially valuable in the field of materials science. It aids in analyzing crystal structures and phase transitions. Research in areas like nanotechnology benefits from Ramanβs ability to provide rapid and non-destructive analysis of materials.
Nuclear Magnetic Resonance (NMR) Spectroscopy
Nuclear magnetic resonance spectroscopy is another powerful technique. It exploits the magnetic properties of atomic nuclei when placed in a strong magnetic field. Different nuclei resonate at characteristic frequencies, providing detailed information about the structure of organic compounds.
NMR spectroscopy is indispensable in organic chemistry for elucidating molecular structures and dynamics. It also finds applications in biochemistry for studying protein structures and interactions. The precision of this technique vastly contributes to advancements in drug development and molecular biology.
Mass Spectrometry
Mass spectrometry measures the mass-to-charge ratio of ions. It enables the determination of molecular weights and structures of compounds. In this technique, ions are generated from samples and then sorted based on their mass.
Mass spectrometry has diverse applications, from clinical diagnostics to environmental testing. It is essential in proteomics and metabolomics for identifying and quantifying biomolecules. The ability to analyze complex mixtures with high sensitivity makes it a favored choice in analytical labs.
Applications in Chemistry
The applications of spectroscopy in chemistry represent a cornerstone of modern analytical methods. Spectroscopy empowers chemists to decipher the makeup of substances, predict behaviors in reaction scenarios, and maintain the integrity of pharmaceutical products. Understanding these applications enhances both fundamental research and industrial practices within chemistry.
Analyzing Chemical Composition
Analyzing chemical composition is a primary application of spectroscopy. Techniques such as Infrared Spectroscopy and Ultraviolet-Visible Spectroscopy allow for the identification of functional groups and molecular structures. This process often involves obtaining spectra that reflect the unique characteristics of molecules. For instance, the peaks in an Infrared spectrum correspond to specific bond vibrations, helping chemists identify compounds present in a mixture.
A common practice involves the use of Mass Spectrometry, which can provide molecular weights and fragment structures. This can effectively help in characterizing unknown substances. Moreover, it assists in determining the purity of a sample, which is crucial in both research settings and product development.
Studying Reaction Mechanisms
Studying reaction mechanisms is essential for understanding chemical reactions at a molecular level. Spectroscopic techniques enable researchers to observe transient species formed during reactions. For example, time-resolved spectroscopy allows scientists to track changes over time, revealing the sequence of intermediate complexes. This detailed insight can lead to a more robust understanding of reaction pathways and kinetics.
By using Nuclear Magnetic Resonance (NMR) Spectroscopy, chemists can visualize the changes in molecular configuration as reactions proceed. NMR data can elucidate stereochemistry and dynamic interactions in real-time. This kind of analysis strengthens the predictive ability of reaction behaviors in different conditions, further enhancing the field of synthetic chemistry.
Quality Control in Pharmaceuticals
Quality control is crucial in pharmaceuticals, and spectroscopy plays a pivotal role in ensuring product safety and efficacy. Techniques like near-infrared spectroscopy are commonly applied for bulk material analysis in manufacturing. This nondestructive approach allows for the evaluation of physical properties without altering the sample's integrity.
Compliance with regulatory standards is vital in drug production. Spectroscopic methods aid in confirming chemical identity and concentration levels, fulfilling compliance requirements. For instance, high-performance liquid chromatography (HPLC) coupled with UV-Vis detection can assess whether formulation components are within acceptable limits.
"Spectroscopy in pharmaceutical applications not only ensures safety but also enhances the efficiency of the production process."
In essence, the integration of spectroscopy in chemistry not only streamlines analysis but also opens new avenues for research and development, ultimately leading to innovations in product formulation and safety standards.
Applications in Biology
Spectroscopy has become a pivotal tool in biological research and applications. Its ability to analyze the composition and structure of biomolecules provides significant advantages in understanding complex biological processes. The relevance of this topic cannot be overstated, as it informs advances in research that can lead to innovative solutions in medicine, environmental science, and biotechnology.
Characterizing Biomolecules
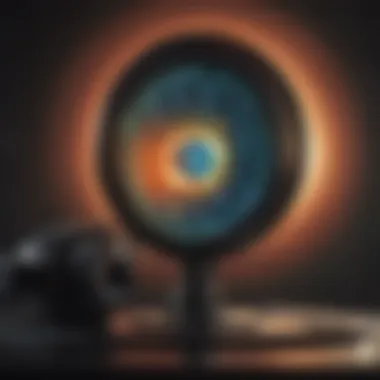
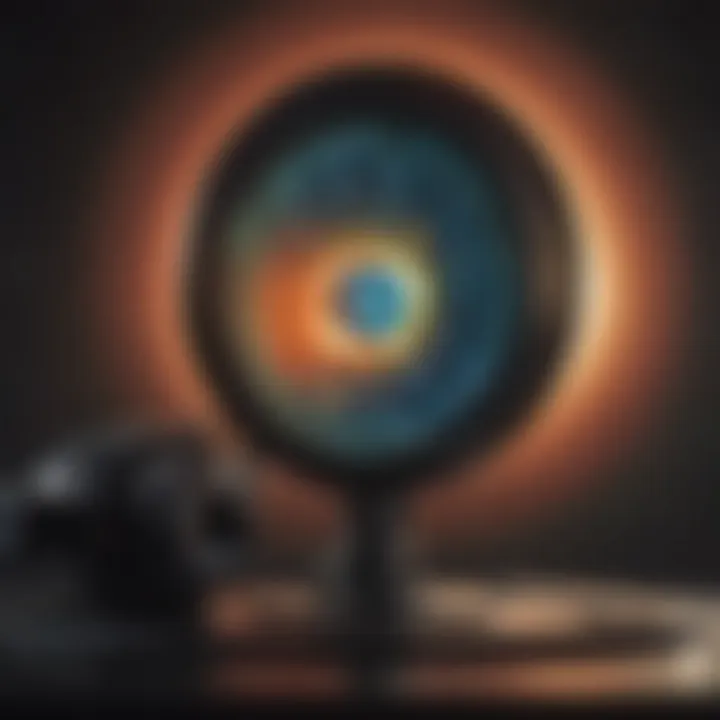
The characterization of biomolecules is essential for comprehending their function and interactions. Techniques such as Nuclear Magnetic Resonance (NMR) spectroscopy and Fourier-transform infrared (FTIR) spectroscopy allow researchers to elucidate the structure of proteins, nucleic acids, and other macromolecules. Understanding the three-dimensional structure of proteins, for instance, can reveal how these molecules perform their roles in the body. This information is critical for drug development, as it facilitates the design of molecules that can effectively target specific biomolecules.
- NMR Spectroscopy is particularly useful for studying protein folding and dynamics due to its ability to provide detailed information without needing large quantities of sample material.
- FTIR Spectroscopy helps to identify functional groups within biomolecules, offering insights into chemical bonding and interactions.
By employing these methods, scientists can gain insights into the biological pathways and mechanisms that underpin diverse physiological processes.
Metabolomics and Profiling Techniques
Metabolomics, the study of small molecules in biological samples, has been significantly advanced by spectroscopic techniques. Mass spectrometry and nuclear magnetic resonance are among the primary tools employed for this purpose. These methods allow researchers to profile metabolites in various biological samples, such as blood and tissues, which can highlight metabolic changes associated with diseases or other biological states.
- Mass Spectrometry can analyze complex mixtures and identify numerous metabolites, enabling a comprehensive view of metabolic pathways.
- Raman Spectroscopy can also be used for non-invasive analysis of metabolites in living organisms.
Understanding metabolomics through these techniques holds promise for personalized medicine, wherein metabolic profiles can guide treatment decisions tailored to individual patients.
Applications in Medical Diagnostics
Spectroscopy has greatly influenced medical diagnostics, enhancing the accuracy and speed of disease detection. Techniques such as optical spectroscopy and fluorescence spectroscopy enable the identification of disease markers in clinical samples, often at an early stage, which is crucial in effective disease management.
- Optical Spectroscopy can be utilized in detecting cancer by analyzing tissue samples. Changes in the spectrum can indicate malignancies, allowing for earlier interventions.
- Fluorescence Spectroscopy assists in immunoassays, identifying specific proteins or antigens related to diseases, providing rapid and reliable results.
The incorporation of these techniques into routine clinical practice continues to evolve, offering the potential to improve patient outcomes through better diagnostic capabilities.
Applications in Physics and Material Science
Spectroscopy plays a critical role in the realm of physics and material science. With its ability to analyze and characterize materials at the molecular and atomic levels, it informs our understanding of fundamental properties. The techniques employed in spectroscopy bridge theoretical models with practical applications, enabling researchers and engineers to harness material characteristics for various uses.
Among the benefits is the capacity to investigate the interaction between light and matter, which reveals essential information about materials' optical and electronic properties. Moreover, this understanding drives innovation in developing new materials with desired performance traits, such as semiconductors, superconductors, and nanomaterials. Several specific elements contribute to the importance of spectroscopy in this field:
- Accurate Characterization: Spectroscopic techniques allow precise identification of material composition and phase.
- Non-Destructive Analysis: Many methods offer non-invasive examination, preserving the integrity of samples.
- Versatile Applications: From fundamental research in quantum physics to practical uses in the electronics industry, spectroscopy offers broad applications.
Studying Optical Properties of Materials
Understanding the optical properties of materials is one of the primary applications of spectroscopy in physics and material science. Techniques such as UV-Vis spectroscopy and infrared spectroscopy provide insights into how materials absorb, reflect, and transmit light.
For instance, UV-Vis spectroscopy analyzes the absorption of ultraviolet and visible light by materials, revealing electronic transitions in molecules. This information is valuable for applications such as developing new pigments, dyes, and optical devices.
Key aspects to consider include the following:
- Band Gap Determination: Optical spectroscopy helps in measuring the band gap of semiconductors, which is critical for electronics.
- Quality Assessment: The degree of light absorption can indicate the purity and concentration of a material, thus supporting quality control efforts.
Surface Analysis Techniques
Surface analysis is another vital area where spectroscopy finds significant application. Techniques like X-ray photoelectron spectroscopy (XPS) and Auger electron spectroscopy (AES) enable detailed examination of the surface composition and chemical states of materials.
These techniques allow researchers to assess surface coatings, contaminants, and other factors that may affect material performance. In industries such as electronics and materials engineering, surface properties can dictate functionality and longevity.
Consider these points when discussing surface analysis techniques:
- Chemical State Identification: Spectroscopy can identify specific chemical states of elements on surfaces, aiding in process optimization.
- Thickness Measurement: Techniques like ellipsometry determine thin film thickness, essential in semiconductor fabrication.
"Spectroscopy reveals the hidden properties of materials, enabling advancements across multiple scientific disciplines."
Emerging Trends in Spectroscopy
The field of spectroscopy continually evolves, driven by technological advancements and the increasing need for precise analysis in various scientific disciplines. Emerging trends in this area highlight the necessity for researchers and professionals to stay updated on the latest techniques and applications. It is essential for enhancing analytical capabilities and addressing complex challenges in scientific research and industry. This section will explore recent advancements, integration with data science, and the progression of remote and in-situ spectroscopy.
Advancements in Spectroscopic Technology
Recent innovations have significantly improved the capabilities of spectroscopic methods. High-resolution instruments are now more accessible, allowing for better detection limits and precision. Techniques such as Fourier-transform infrared (FTIR) spectroscopy and high-resolution mass spectrometry are at the forefront of these advancements.
Some of the notable trends include:
- Miniaturization: Smaller, portable devices enable field measurements, making spectroscopy more practical in various environments.
- Improved Detectors: New detector technologies enhance sensitivity and dynamic range, allowing for the detection of trace compounds.
- Automation and Robotics: The integration of robotic systems with spectroscopic instrumentation increases throughput and reduces human error in data collection.
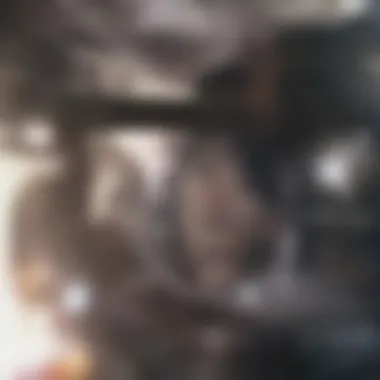
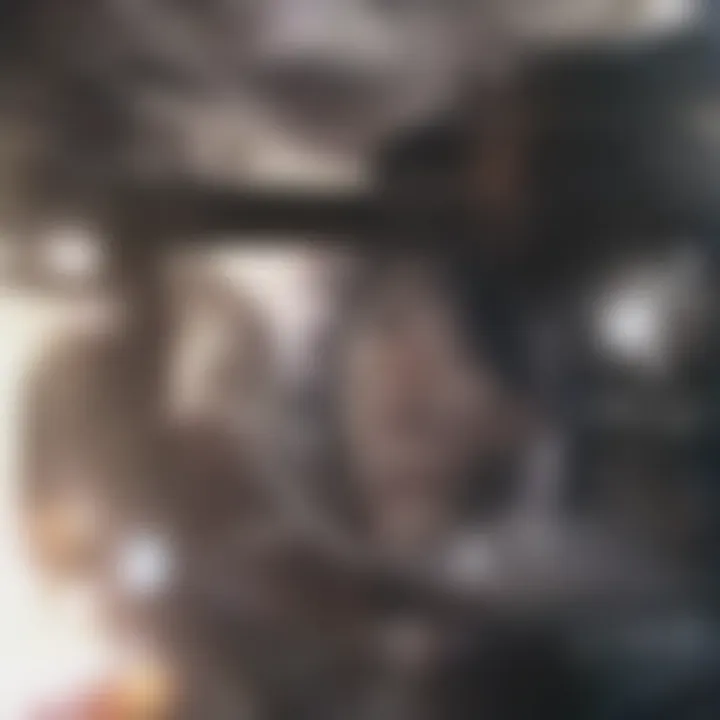
Integration with Data Science
As spectroscopy generates a vast amount of data, integrating data science techniques has become increasingly important. Machine learning and artificial intelligence can interpret complex spectroscopic data more efficiently than traditional methods. This integration aids in identifying patterns and making predictions that enhance scientific understanding.
Key aspects of this integration include:
- Data Analytics: Advanced analytical methods provide deeper insights into the data, allowing for better characterizations of materials.
- Predictive Modeling: Leveraging algorithms to predict the behavior of materials based on spectroscopic data enhances research efficiency.
- Visualization Tools: Powerful visualization techniques help in presenting complex data sets in more understandable formats, facilitating better communication of findings.
Remote and In-Situ Spectroscopy
Remote and in-situ spectroscopy represents a paradigm shift in how spectroscopy is applied. These techniques allow for real-time analysis without the need for sample preparation or laboratory setups. This is particularly beneficial in environmental monitoring and industrial applications.
Advantages of remote and in-situ techniques include:
- Real-Time Data: Immediate analysis of samples in their native environment leads to timely decision-making.
- Reduced Sample Handling: Decreasing sample handling minimizes contamination risks and potential alterations in chemical properties.
- Versatile Applications: These methods are crucial in various fields, including agriculture monitoring, pollution detection, and quality control in manufacturing.
"Emerging trends in spectroscopy facilitate the exploration and understanding of materials and interactions at unprecedented levels of detail."
Overall, understanding and adapting to these emerging trends is crucial for professionals in the field. These advancements not only enhance the capabilities of existing spectroscopic techniques but also open doors to new applications in multiple disciplines.
Challenges and Limitations
Analyzing the breadth of spectroscopy uncovers not only its numerous uses but also the challenges and limitations that accompany its application. Understanding these facets is crucial for scientists and professionals who rely on spectroscopic methods in their work. Such insight aids in the development of more robust techniques and better assessment of results, ensuring accuracy and relevance across various fields.
Interference and Noise in Spectra
One of the most significant challenges in spectroscopy is the presence of interference and noise in the spectra obtained. Various factors can contribute to this issue. External environmental elements, such as temperature fluctuations or electronic interference from nearby equipment, can distort the data.
Interfering substances within the sample can also impact the measurements. For instance, impurities in a chemical sample might absorb light wavelengths, affecting the readability of specific signals.
To address this, researchers often use several techniques:
- Careful sample preparation: Ensuring the purity of the sample helps mitigate interference.
- Use of control samples: This provides a baseline to compare against the measured data.
- Advanced algorithms: Signal processing tools can separate noise from relevant data, allowing for cleaner spectra interpretation.
The effectiveness of spectroscopic techniques heavily relies on reducing background noise and interference to achieve reliable results.
Calibration and Standardization Issues
Proper calibration is essential for achieving credible results in spectroscopy. Calibration ensures that the spectroscopic instruments are accurately measuring the spectral data as intended. If an instrument is not calibrated correctly, the resulting spectra may misrepresent the material's properties.
Standardization is another issue faced in this field. With numerous techniques available, varying standards often emerge for different applications. This leads to inconsistency in results across different laboratories and research studies.
To improve calibration and standardization, several approaches are effective:
- Regular calibration with known standards: This practice ensures that instruments maintain accuracy over time.
- Adoption of universal protocols: Establishing common protocols can enhance reproducibility and reliability across different studies and institutions.
In summary, consistently addressing the challenges of interference, noise, calibration, and standardization is vital for the integrity of spectroscopic analyses. By acknowledging these limitations, practitioners can work more effectively and reliably in their respective fields.
End and Future Outlook
The conclusion and future outlook section of this article encapsulates the significance of spectroscopy in various scientific domains. It reiterates the advantages and innovations that stem from further exploring this technique. Spectroscopy is not merely a scientific tool; it bends the boundaries of inquiry and opens up new avenues for understanding material behavior and interaction processes.
As technology advances, the integration of spectroscopy with other disciplines becomes increasingly vital. This synergy enhances analytical capabilities and broadens the scope of research initiatives. With developments in machine learning and artificial intelligence, the potential for real-time data analysis in spectroscopy is becoming more feasible. This could lead to faster and more accurate interpretations of spectral data, which is invaluable in both research laboratories and industrial applications.
Researchers must also consider the environmental impact of spectroscopic methods. By optimizing techniques and employing more sustainable practices, the scientific community can contribute to efforts aimed at minimizing environmental footprints.
The future of spectroscopy is interwoven with advancements in technology and a commitment to ethical research practices.
Summary of Key Insights
In summarizing the key insights of this article, it is evident that spectroscopy serves as a foundational tool in various fields of study. This method not only aids in understanding the composition and properties of materials but also plays a critical role in advancing scientific inquiry across disciplines.
- Integration with Technology: Spectroscopy techniques are evolving with advancements in technology. Enhanced software and hardware increase efficiency and precision.
- Applications Across Disciplines: From chemistry to biology and physics, spectroscopy has diverse applications that facilitate significant scientific breakthroughs.
- Role in Industry: Industries such as pharmaceuticals and material science rely heavily on spectroscopy for quality control and product development.
Potential Future Applications
Looking ahead, several potential applications of spectroscopy stand out. These developments hint at both challenges and exciting prospects for scientific research and its practical implementations.
- Personalized Medicine: As the healthcare sector evolves, spectroscopy may play a crucial role in tailoring treatments to individual patient needs. Analyzing biomarkers through spectroscopy could enhance diagnostic precision and treatment efficacy.
- Environmental Monitoring: Spectroscopic methods may evolve to assess environmental changes more accurately. By detecting pollutants or assessing soil and water quality in real time, professionals can address ecological issues more proactively.
- Security Screening: The technique could strengthen security measures in various industries. Non-invasive and rapid analysis using spectroscopy might enhance safety in public places, transportation hubs, and other critical infrastructures.
- Nanotechnology: As nanomaterials become more prevalent, spectroscopy may provide insight into their properties and behaviors at the molecular level. This could lead to advances in material science and engineering.
As the field of spectroscopy continues to expand, it will undoubtedly catalyze innovations that shape the future of science and industry.