AAV Vectors in Gene Therapy: Innovations and Insights
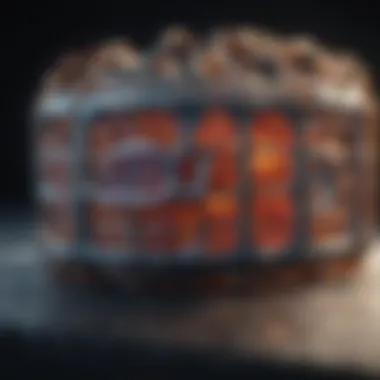
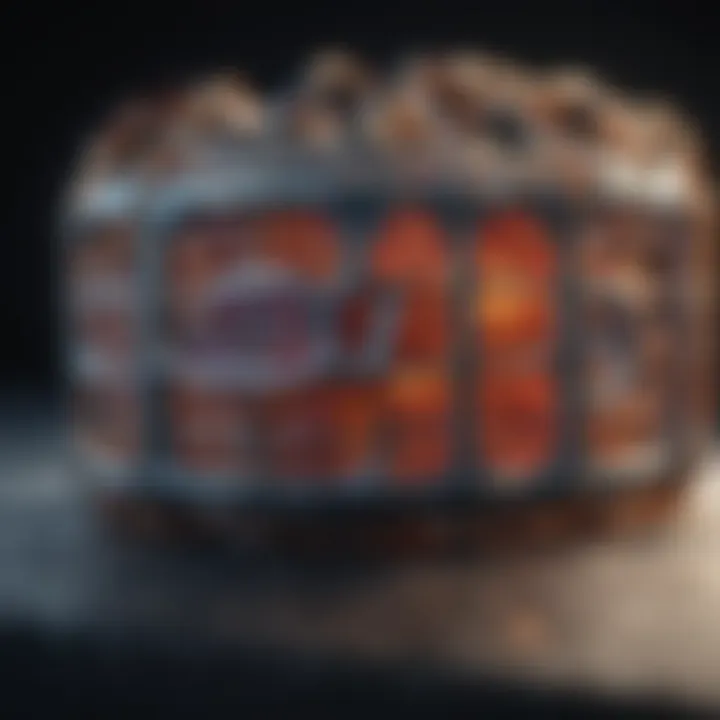
Intro
Adeno-associated virus (AAV) vectors play a crucial role in the emerging field of gene therapy. Their unique properties have made them a preferred tool for delivering genetic material into cells. This advancement in biotechnology has brought hope for treating a variety of genetic disorders. The versatility and effectiveness of AAV vectors make them a focal point in research and therapy development.
Background and Context
Overview of the Research Topic
Gene therapy seeks to correct defective genes responsible for disease development. AAV vectors are small viruses that can transport genetic information with low toxicity. They have gained attention for their safety profile and ability to transduce a range of cell types. These vectors can enable long-term expression of therapeutic genes, addressing chronic conditions effectively.
Historical Significance
The journey of AAV vectors began in the late 20th century. Initially, significant research focused on the characteristics of AAV. In the early 2000s, the use of AAV vectors started to gain footing in clinical trials, showing promise in treating disorders like hemophilia and retinal diseases. The innovative approaches in vector design and production methods contributed to the rapid progression of AAV-based therapies.
Key Findings and Discussion
Major Results of the Study
Current research into AAV vectors reveals multiple serotypes, which exhibit different tropisms. Each serotype offers distinct advantages for specific types of tissues. Recent advances highlight the potential of engineered AAV capsids that enhance transduction efficiency and specificity. Some studies have shown that modified capsids increase delivery accuracy, which is vital for therapeutic applications.
Detailed Analysis of Findings
The production methods for AAV vectors have improved significantly. Techniques such as the use of helper plasmids and transient transfection have streamlined the vector production process. The challenges of scalability and cost-effectiveness remain topics of ongoing research. Regulatory considerations are also critical; agencies such as the FDA provide guidelines that must be followed for clinical use.
"The potential applications of AAV vectors in various diseases demonstrate their significance in advancing gene therapy."
Research is continuously evolving, pointing toward broader applications in genetic disorders beyond the existing treatments. Areas under exploration include neurological disorders, muscular dystrophies, and some types of cancers. Understanding both the advantages and limitations of AAV vectors is essential to unlocking their full therapeutic potential.
Prelude to AAV Vectors
Adeno-associated virus (AAV) vectors represent a significant advancement in the field of gene therapy. Understanding AAV vectors is crucial because they offer a safer alternative for delivering therapeutic genes into cells. This section will provide a foundational knowledge necessary to comprehend their role, benefits, and the wider implications in the healthcare landscape.
Definition and Overview
AAV vectors are derived from a small virus that requires a helper virus for replication. They are classified as non-pathogenic and are attractive for gene delivery due to their ability to infect both dividing and non-dividing cells. Typically, an AAV vector contains a single-stranded DNA genome encapsulated within a protein coat known as a capsid. This structure allows for the targeted and stable integration of therapeutic genes into the host genome.
In gene therapy, the main goal is to correct or replace faulty genes. AAV vectors have been utilized in various applications, including hereditary diseases, neurological disorders, and some forms of cancer. Their use often results in a prolonged expression of the therapeutic gene, which is vital for diseases where continual treatment is necessary.
Historical Context
The history of AAV vectors dates back to the late 1960s when researchers first isolated the virus from human tissues. However, it was not until the late 1990s that significant progress was made in employing AAV for gene therapy. Early clinical trails highlighted the safety and potential of these vectors, leading to a peak interest in their therapeutic uses.
Over the years, many studies have demonstrated the efficacy of AAV vectors in various applications. For instance, the first successful human trials utilizing AAV vectors occurred in the 2000s aimed at addressing genetic disorders like Leber's congenital amaurosis. Such milestones cemented the viability of AAV vectors in clinical settings, paving the way for numerous ongoing research initiatives focused on refining their applications.
Advances in molecular biology techniques now allow for the engineering of AAV vectors with enhanced properties. These innovations include bioengineering the capsid to increase tissue specificity, improve transduction efficiency, and reduce immunogenic response. Such technologies are essential for translating AAV vectors from experimental environments into mainstream clinical therapies.
Molecular Structure of AAV Vectors
The molecular structure of AAV vectors is fundamental to their effectiveness and application in gene therapy. Understanding this structure allows researchers and practitioners to appreciate how these vectors operate within the biological systems of living organisms. Key elements include the genome organization and capsid structure, both of which contribute to the vector's functionality and versatility.
Genome Organization
The genome of AAV vectors is relatively compact compared to other viral systems. Typically, it consists of a single-stranded DNA that can efficiently encapsulate a therapeutic gene. This organization is crucial because it allows for precise targeting and expression of genes within host cells. The ability of the AAV genome to incorporate into the host DNA without disrupting essential functions is a significant advantage, reducing the risk of insertional mutagenesis.
AAV vectors often utilize inverted terminal repeats (ITRs) at both ends of the genome. These ITRs play several roles, including facilitating the replication of the viral genome and ensuring proper packaging during the assembly of the vector. The specific configuration influences how the vector interacts with the host's cellular machinery, enhancing transduction efficiency.
The structured nature of the AAV genome not only supports robust gene delivery but also offers stability, crucial for long-term therapeutic effects.
Capsid Structure
The capsid of AAV vectors is composed of proteins that form a protective shell around the genomic material. This structure is critical because it determines the vector's serotype and influences its immunogenic profile. The capsid is built from 60 subunits, predominantly comprising three main protein types: VP1, VP2, and VP3. Each of these proteins has distinct roles, contributing to the stability and infectivity of the vector.
Capsid proteins also dictate the vector's ability to bind to specific cellular receptors, allowing for targeted transduction. Different serotypes exhibit variations in their capsid composition, leading to differences in tissue tropism, or the specific types of cells they can effectively enter.
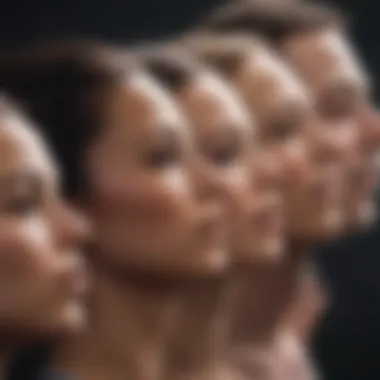
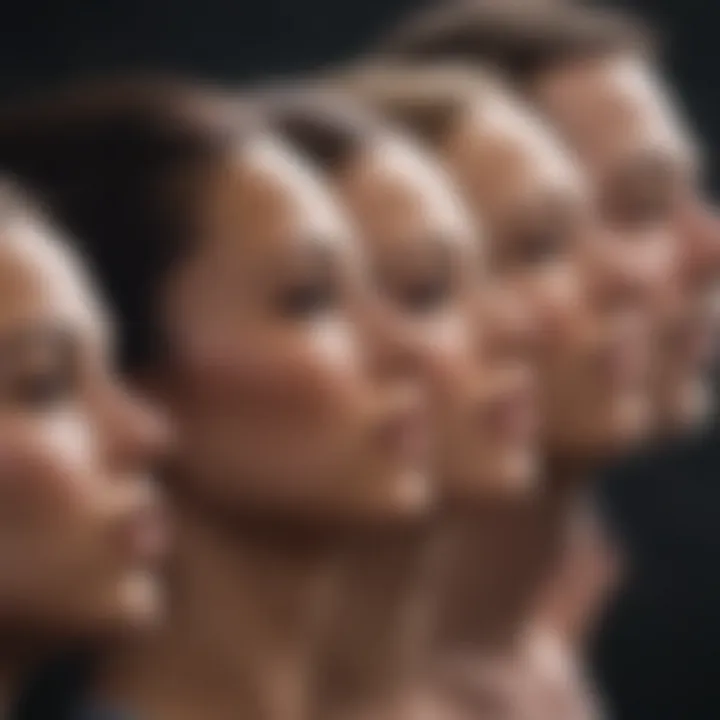
Moreover, innovations in capsid engineering are underway, aimed at enhancing target specificity and reducing immune response. Such advancements are critical for improving the safety and efficacy profiles of AAV vectors in clinical application. Understanding capsid structure thus not only aids in current applications but also sets the groundwork for future innovations in gene therapy.
In summation, the molecular structure of AAV vectors, encompassing both genome organization and capsid structure, is vital for their functionality in gene therapy. These structural components are essential in deciphering how AAV vectors can be optimized for various therapeutic applications.
Serotypes of AAV Vectors
The diverse world of AAV vectors is underscored by their various serotypes, which play a crucial role in defining their utility in gene therapy. Each serotype possesses unique properties that influence transduction efficiency, tissue tropism, and immunogenicity. Understanding these serotypes is essential for optimizing vector design and selecting appropriate candidates for specific therapeutic applications.
Common Serotypes
Common serotypes of AAV vectors have been extensively studied in the context of gene therapy. The most well-known among these are AAV2, AAV8, and AAV9. Each of these serotypes displays distinct characteristics:
- AAV2: This serotype is recognized for its ability to transduce a wide range of cell types and tissues, making it a popular choice in early gene therapy trials. AAV2 is favored for its integration capabilities in the host genome, offering prolonged gene expression.
- AAV8: Compared to AAV2, AAV8 exhibits enhanced efficiency in targeting liver tissues. This makes it a prime candidate for therapies focused on hepatic diseases, such as hemophilia and other metabolic disorders.
- AAV9: Known for its ability to cross the blood-brain barrier, AAV9 is vital for treating neurological disorders. Its unique ability to reach central nervous system tissues opens avenues for potential therapies in conditions like spinal muscular atrophy and neurodegenerative diseases.
These common serotypes have paved the way for understanding the functional capabilities of AAV vectors, driving advancements in gene therapy approaches. However, optimizing their use requires careful consideration of each serotype's specific properties.
Emerging Serotypes
In addition to the well-established common serotypes, emerging AAV serotypes are gaining attention for their potential applications in gene therapy. Researchers have been isolating and characterizing new AAV variants, leading to a more extensive toolbox for therapeutic options. Some notable emerging serotypes include AAVr0 and AAV-DJ.
- AAVr0: This serotype shows enhanced muscle tropism, making it suitable for gene delivery in muscle diseases such as Duchenne muscular dystrophy. Researchers are investigating its advantages in promoting efficient gene transfer and expression in muscle tissues.
- AAV-DJ: A hybrid serotype, AAV-DJ combines features from several AAV serotypes, including AAV2, AAV8, and AAV9. Its unique properties offer broad tissue targeting capability and lower immunogenic responses, enhancing its utility in clinical applications.
The continuous discovery of emerging serotypes expands the potential therapeutic landscape of AAV vectors. With ongoing research, the ability to tailor gene therapies to meet specific medical needs becomes increasingly feasible, marking a significant step forward in personalized medicine.
"Serotypes influence both the efficacy and safety of gene therapy, highlighting the importance of careful selection based on the therapeutic goal."
In summary, understanding the serotypes of AAV vectors is fundamental for advancing gene therapy. The characteristics of each serotype impact their viability for different conditions, providing a framework for optimizing therapeutic approaches.
Production of AAV Vectors
The production of AAV vectors is fundamental to their application in gene therapy. As the technology continues to evolve, understanding the various production methods and their implications becomes crucial. Effective production ensures that AAV vectors meet the necessary quality, efficacy, and safety requirements for therapeutic use. Inadequate production processes can lead to suboptimal vector yield, diminished integration capability, and various safety concerns, which ultimately affect treatment outcomes.
Methods of AAV Vector Production
AAV vectors can be produced using several methods, and each method offers distinct advantages and drawbacks. The primary approaches include:
- Plasmid Transfection: This method involves co-transfecting cells with multiple plasmids containing the AAV genome and helper genes. It is a straightforward approach that is cost-effective and enables scalability. However, the process can be inefficient and may result in variable yields.
- Infection of Packaging Cell Lines: This technique utilizes packaging cell lines, such as HEK293 or their derivatives, to produce AAV vectors. By infecting these cells with helper viruses like adenovirus, high-titer vectors can be obtained. This method supports large-scale production and offers consistent yields.
- Baculovirus Expression System: In this method, baculovirus is utilized to transduce insect cells that produce AAV vectors. This system allows for high yield production of vectors and avoids human cell contaminants. However, it also poses challenges with the purification process.
- Self-Complementary AAV (scAAV) Production: ScAAV vectors are produced by equipping the AAV with an additional sequence that enhances their transduction efficiency. This technique increases the speed of gene expression but may complicate the overall production workflow.
Overall, the choice of method depends on the desired yield, efficiency, and specific application of the AAV vector in therapeutic situations.
Quality Control Measures
Quality control is an essential aspect of AAV vector production. With the increasing focus on safe and effective gene therapy, quality assurance measures must be stringently followed. Key elements of quality control include:
- Characterization of Vector Preparation: Thorough characterization is necessary to confirm the identity, purity, and potency of the produced AAV vectors. Techniques like qPCR, ELISA, and Southern blotting are commonly employed for this purpose.
- Contamination Testing: Ensuring that the AAV vectors are free from contaminants, such as bacteria, residual nucleic acids, or proteins from the production system, is critical. Tests are done to detect the presence of these contaminants, which if undetected, can lead to adverse patient reactions.
- Stability Assessment: The stability of AAV vectors over time must be evaluated to guarantee that they remain effective throughout their intended shelf life. Stability studies help determine the conditions for storage and transport.
The regulations governing the production and quality control of AAV vectors are designed to protect both patient safety and therapeutic efficacy.
"The efficiency of the production process has a direct impact on the success rate of gene therapies using AAV vectors."
Mechanisms of AAV Vector Action
Understanding the mechanisms of AAV vector action is crucial for elucidating how these vectors function effectively in gene therapy. This section investigates the distinct processes through which AAV vectors enter host cells, transduce, and ultimately facilitate gene expression. Insights into these mechanisms provide foundational knowledge for students, researchers, and professionals interested in innovative therapeutic strategies using AAV technology.
Viral Entry and Transduction
The first step in the action of AAV vectors is viral entry into target cells. This stage is characterized by a complex interaction between the AAV capsid proteins and a variety of host cell surface receptors. For most AAV serotypes, including AAV2 and AAV8, the entry process is receptor-mediated endocytosis. Through this mechanism, the virus attaches to the cellular receptor, which induces the uptake of the viral particle into the cell.
Once internalized, AAV vectors must escape the endosome to reach the nucleus, where the genetic material can exert its therapeutic effects. Uncoating occurs, which involves a series of conformational changes in the capsid, liberating the viral genome. Importantly, the ability of AAV vectors to utilize various receptors offers a broad range of possibilities for targeting different cell types.
The efficiency of transduction is influenced by several factors:
- Receptor availability: The expression levels of receptors on target cells play a vital role in uptake.
- Cell cycle status: AAV vectors typically integrate their genetic material during the S phase of the cell cycle, making dividing cells a prime target.
- Capsid modifications: Engineering capsids can enhance binding affinities, thereby improving transduction efficiency.
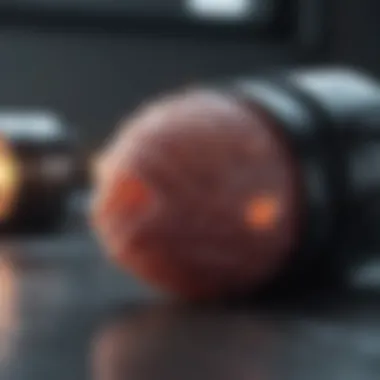
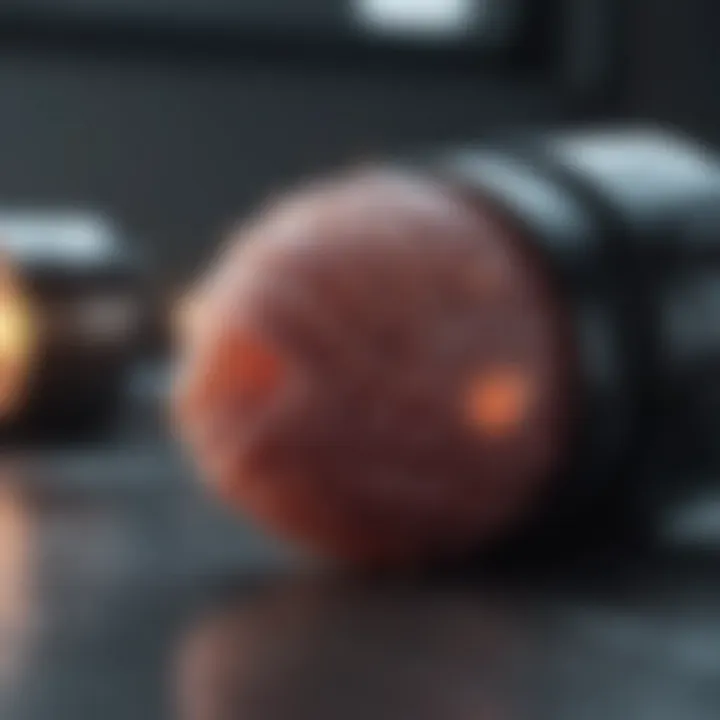
Gene Expression and Integration
Following successful transduction, the next critical phase involves gene expression. The AAV vector's genome, which consists of single-stranded DNA, requires conversion to a double-stranded form inside the nucleus. Once this conversion is complete, the vector can drive the production of therapeutic proteins. The expression duration may vary depending on several factors, including the serotype used, the administration route, and the cellular environment.
Notably, AAV vectors have a unique ability to integrate into the host genome, although they predominantly remain in an episomal state, which is non-integrating. However, for some serotypes, such as AAV8, integration into specific chromosomal sites can occur, allowing for stable, long-term expression of the transgene. This phenomenon provides an advantageous aspect in the treatment of genetic disorders, where a prolonged effect is desired.
"The mechanisms of AAV vectors highlight their potential as a powerful tool in gene therapy, particularly when understanding their entry and integration properties."
In summary, comprehending these mechanisms underscores the versatility and utility of AAV vectors in therapeutic contexts, paving the way for advancements in gene therapy methodologies. As research progresses, these insights will guide the design of next-generation vectors and refine strategies for targeting specific diseases.
Therapeutic Applications of AAV Vectors
The exploration of adeno-associated virus (AAV) vectors encapsulates a significant and evolving area of gene therapy. AAV vectors have gained prominence due to their ability to deliver therapeutic genes into target cells efficiently. This natural virus, which is non-pathogenic to humans, offers unique advantages such as a low immunogenic profile and the potential for long-term expression of the delivered genes. The growing body of research surrounding AAV vectors has opened new avenues for treating various diseases, making it a focal point in modern medical interventions.
Genetic Disorders
Genetic disorders arise from mutations or abnormalities in an individual's DNA. AAV vectors present a promising approach to correcting these disorders at the molecular level. For instance, certain conditions like spinal muscular atrophy and various forms of hemophilia can be addressed through the targeted delivery of functional copies of affected genes. The use of AAV vectors allows the introduction of therapeutic genes directly into affected tissues, potentially alleviating symptoms or, in some cases, providing a cure.
Noteworthy examples include the development of Zolgensma, an AAV-based gene therapy that has shown efficacy in treating spinal muscular atrophy. The outcomes for patients treated with this therapy have been significantly positive, demonstrating improved motor function and overall quality of life.
The application of AAV vectors in genetic disorders also brings some challenges. The need for precise targeting and potential immune responses must be carefully considered, as they can influence the therapy's success. However, ongoing research focuses on optimizing these vectors to enhance their efficacy and reduce adverse reactions.
Curative Approaches in Oncology
In the realm of oncology, AAV vectors are being explored not merely as delivery systems for tumor suppressor genes but also for generating antitumor immunity. The unique properties of AAV vectors allow them to transduce a wide variety of cell types, including those in solid tumors. This characteristic is crucial for gene therapies that aim to reprogram the tumor microenvironment or provoke a systemic immune response against cancer cells.
AAV-mediated expression of therapeutic proteins can inhibit tumor growth or even directly induce cancer cell death. Recent studies have shown promising results, particularly in cancers where traditional treatment options have failed. Targeting specific genetic defects in tumors with AAV vectors helps create personalized treatment strategies, maximizing therapeutic benefits while minimizing damage to surrounding healthy tissues.
Nevertheless, the translation of these therapies into clinical practice involves navigating complex challenges such as targeting efficiency, vector persistence, and potential off-target effects, which requires further investigation and refinement.
Infectious Diseases
AAV vectors have potential applications beyond genetic disorders and oncology; they are also being investigated for the treatment of infectious diseases. For instance, the delivery of genes encoding antiviral proteins or antibodies can be used to combat viral infections. Research has shown that AAV vectors can be effectively used to elicit protective immune responses against diseases like HIV and Hepatitis B.
The ability of AAV vectors to stably express these proteins presents a long-lasting defense mechanism against viral pathogens. This could lead to new preventative or therapeutic strategies that might reduce the burden of chronic viral infections.
However, several considerations arise with these applications, including the need for robust immune responses and the potential for the development of vector-neutralizing antibodies. These aspects necessitate thorough exploration to unlock the full potential of AAV vectors in treating infectious diseases.
AAV vectors demonstrate versatility as a therapeutic tool across various fields, from genetic disorders to oncology and infectious diseases, showcasing the profound impact they can have on gene therapy.
Regulatory Landscape for AAV Vectors
The regulatory landscape for AAV vectors plays a crucial role in ensuring the safety, efficacy, and ethical deployment of gene therapy products. Regulatory bodies establish guidelines that govern the research, development, and distribution of these vectors, impacting their acceptance in clinical practices. The thorough evaluation through stringent processes allows for advancements in therapeutic strategies while maintaining high standards of patient protection and ethical considerations. A clear understanding of this landscape is essential for researchers and developers working with AAV vectors.
Approval Processes
The approval processes for AAV vectors entail a series of steps that must be met before these products can be utilized in clinical settings. Regulatory agencies like the U.S. Food and Drug Administration (FDA) and the European Medicines Agency (EMA) mandate comprehensive data related to safety, quality, and efficacy.
Typically, these approval phases include:
- Preclinical Studies: Initial studies are conducted to evaluate the biological impact of the AAV vectors.
- Clinical Trials: Testing proceeds in phases involving human participants, which helps to assess safety and dosage.
- Regulatory Submission: A detailed dossier presenting research findings is submitted to the regulatory authority for review.
- Post-Marketing Surveillance: After approval, ongoing monitoring is required to track the long-term effects of AAV therapies.
A thorough approval process is key to ensuring that AAV vectors are both safe and effective for patients, which builds public trust in emerging gene therapies.
Ethical Considerations in Gene Therapy
When discussing the regulatory landscape, ethical considerations cannot be overlooked. Gene therapy holds transformative potential, but it also raises significant ethical questions. Some of these considerations include:
- Informed Consent: A critical aspect of conducting trials. Participants must fully understand the risks and benefits of AAV vector treatments.
- Equity in Access: Ensuring that all populations have access to gene therapies, regardless of socioeconomic status, is essential for ethical practice.
- Long-term Implications: The possibility of unintended effects or consequences must be considered, particularly with heritable gene therapies.
- Biologial Safety: Addressing concerns about the use of viral vectors and their potential to elicit immune responses.
"Ethical frameworks must adapt alongside scientific advancements in gene therapy to safeguard patient rights and promote equitable practices."
Challenges and Limitations of AAV Vectors
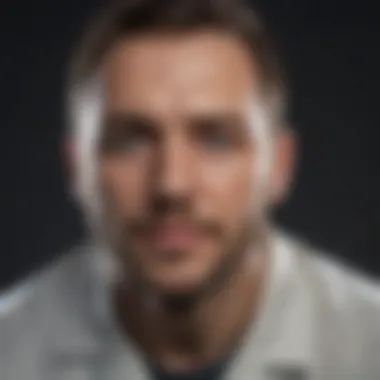
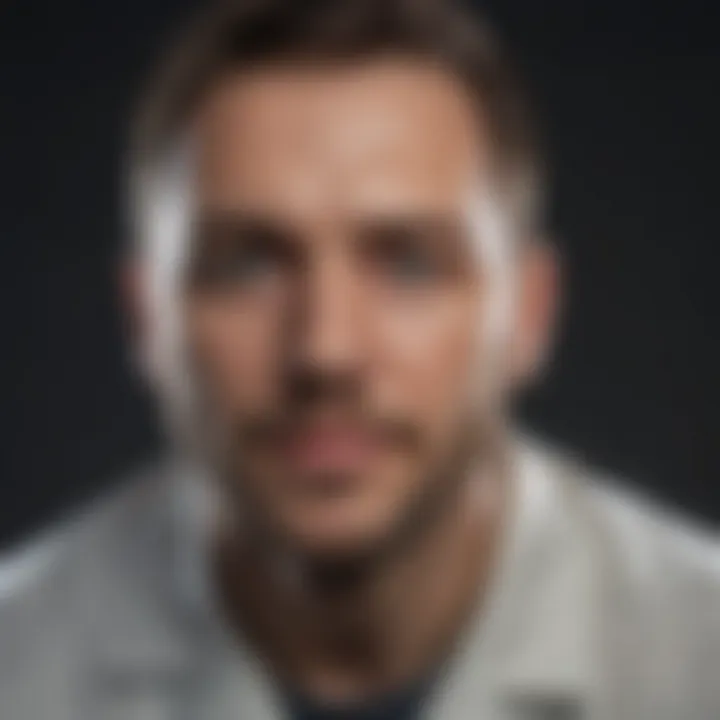
Adeno-associated virus vectors are widely revered for their potential in gene therapy. However, the path to their successful application is not devoid of challenges. Understanding the limitations of AAV vectors is crucial for researchers and clinicians alike, as it helps guide future developments and applications.
The significance of addressing these challenges is twofold. Firstly, it ensures safety and efficacy in clinical applications. Secondly, recognizing the limitations allows for innovation in vector design and delivery strategies, enhancing therapeutic outcomes.
Immunogenic Responses
Immunogenic response is a primary concern in the application of AAV vectors. The immune system may recognize AAVs as foreign particles, leading to the activation of both humoral and cellular immune responses. This can result in neutralization of the vector before it reaches the target cells, thus reducing the effectiveness of the therapeutic intervention.
In the clinical setting, pre-existing immunity to certain serotypes of AAV can also present a hurdle. In many patients, the presence of neutralizing antibodies means that previously administered doses may not deliver the desired therapeutic gene. As a result, the inducement of immune tolerance, along with devising serotypes that minimize immunogenicity, is a research priority.
Some strategies to manage immunogenic responses include:
- Selection of less immunogenic serotypes: Using emerging serotypes that the patient's immune system has not previously encountered.
- Immunosuppression protocols: Temporarily reducing the patient's immune response prior to vector administration. However, this approach carries its own risks.
Delivery Mechanisms and Limitations
The effectiveness of AAV vectors greatly depends on their ability to deliver therapeutic genes to the intended target cells. Yet, AAV vectors face inherent limitations in their delivery mechanisms.
Physical barriers in the body can impede effective delivery. These may include:
- Tissue permeability: Certain tissues exhibit limited permeability, making it challenging for the vectors to penetrate and transduce target cells.
- Intravenous delivery challenges: When administered through the bloodstream, vectors may not reach specific tissues effectively, potentially necessitating local administration methods, which may not always be possible.
Moreover, the vector's capacity for inserting the gene into the host genome is limited, which can hinder long-term expression. As a result, researchers continue to explore alternative delivery methods and enhance vector design.
The challenges related to AAV vectors must not deter advancement. Instead, they highlight areas for significant improvement. As the field progresses, addressing these limitations can lead to more efficient therapies that ensure both safety and efficacy in gene therapy.
Future Directions in AAV Research
The field of gene therapy is advancing rapidly, and with it, the future directions in adeno-associated virus (AAV) research continue to unfold. AAV vectors hold immense potential for treating a range of genetic disorders and immunological conditions. As the understanding of their capabilities improves, researchers are focusing on several key areas that promise to enhance the efficacy and application of these vectors.
Innovations in Vector Design
Innovations in vector design are crucial for increasing the versatility of AAV vectors. Modifications to both the capsid and genome are areas of intense study. Researchers are experimenting with synthetic biology findings to create customized AAV serotypes that can target specific tissues more effectively.
Some innovative strategies include:
- Capsid Engineering: By altering the capsid proteins, scientists can create variants with optimized tissue tropism. This ensures that the vector efficiently targets specific cell types, thereby improving the therapeutic outcomes.
- Dual AAV Systems: This involves using pairs of AAV vectors to deliver larger genes. By splitting a gene across two vectors, researchers can bypass the size limitations of AAV and still achieve efficient gene therapy.
- Self-Complementary AAV (scAAV): Innovations have led to the development of scAAVs, which can enhance gene expression more rapidly as they provide a double-stranded genome from the start. This can lead to quicker onset of therapeutic effects.
The drive for innovation is fueled by the need to improve specificity and effectiveness, ultimately broadening the range of disorders that can be treated.
Integration with Other Therapeutic Approaches
Integration of AAV vectors with other therapeutic modalities can lead to substantial advancements in treatment strategies. This multi-faceted approach can take advantage of the strengths of different therapies, providing a more comprehensive solution for patients. Some strategies include:
- Combination with CRISPR Technologies: AAV vectors can be utilized for the delivery of CRISPR components to enable precise editing of genetic information. This intersection between gene therapy and gene editing shows great promise for treating genetic diseases more effectively.
- AAV with Immunotherapy: Pairing AAV vectors with cancer immunotherapy can enhance the immune response to cancer cells. By delivering genes that code for immune signaling molecules, it can help in activating the immune system to recognize and attack tumors.
- Gene Therapy and Cell Therapy: The future may see more AAV vectors used alongside cell therapies, enhancing the genetic modification of re-introduced cells after obtaining them from a patient.
Overall, the integration of AAV vectors with various therapeutic approaches can yield synergetic effects, potentially revolutionizing treatment paradigms for complex diseases.
As research progresses, clinicians and scientists must remain vigilant about overcoming challenges such as immunogenicity and delivery efficiency. However, with the advances in vector design and integration of therapies, AAV's impact on gene therapy will likely continue to expand. Understanding and embracing these developments is essential for future applications of AAV in medicine.
End
In this article, the discussion surrounding AAV vectors culminates in a critical examination of their significance in gene therapy. To summarize, these vectors represent a vital advancement in the realm of genetic medicine, due to their unique characteristics and potential applications. AAV vectors provide numerous benefits, including low immunogenicity, the ability to support long-term gene expression, and versatility in targeting various tissues. These features make them a preferred choice for therapeutic interventions aimed at a wide range of genetic disorders.
Summary of Key Insights
AAV vectors have demonstrated a remarkable capacity to facilitate effective gene delivery systems. This article highlighted several key points that are essential to understanding their application:
- Safety and Efficacy: AAV vectors are generally regarded as safe due to their ability to induce a low immune response, which minimizes the risk of adverse effects in patients.
- Diverse Serotypes: The existence of multiple AAV serotypes allows for tailored therapeutic solutions by enhancing targeting specificity and efficiency.
- Clinical Applications: The expanding range of therapeutic applications showcases AAV vectors' potential to treat genetic disorders, infectious diseases, and even certain types of cancer.
- Regulatory Landscape: Understanding the regulatory environment is crucial as it determines the pathways through which AAV-based therapies can be approved and brought to the clinic.
These insights not only reflect the current state of AAV vector research but also underscore the growing body of evidence supporting their use in diverse clinical settings.
Implications for Future Studies
The future of AAV vector utilization in gene therapy is promising, yet it also poses several challenges that researchers must address. Future studies should focus on:
- Enhancing Vector Design: Innovations in vector engineering are necessary to improve the delivery efficiency of therapeutic genes and to combat the immune responses that may arise upon administration.
- Combination Therapies: Investigating how AAV vectors can be effectively combined with other therapeutic modalities—such as small molecules or CRISPR technology—can lead to more effective treatment regimens.
- Long-term Studies: Continuous monitoring of long-term safety and efficacy in clinical trials is essential to ensure the sustainability of AAV-based treatments.
- Expanding Applications: Research can also explore novel applications beyond current uses, such as neurological diseases and regenerative medicine.
In summary, AAV vectors stand as a critical component in advancing gene therapy. The points discussed in this article not only provide a comprehensive review of their capabilities but also signify the ongoing evolution of genetic treatment strategies. The implications of continued research in this area will undoubtedly shape the future landscape of medicine.